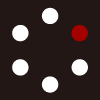
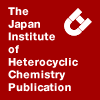
HETEROCYCLES
An International Journal for Reviews and Communications in Heterocyclic ChemistryWeb Edition ISSN: 1881-0942
Published online by The Japan Institute of Heterocyclic Chemistry
e-Journal
Full Text HTML
Received, 16th September, 2008, Accepted, 15th October, 2008, Published online, 23rd October, 2008.
DOI: 10.3987/REV-08-SR(D)1
■ Epibatidine Analogs Synthesized for Characterization of Nicotinic Pharmacophores—A Review
F. Ivy Carroll*
Organic and Medicinal Chemistry, Research Triangle Institute, Research Triangle Park, NC 27709, U.S.A.
Abstract
In 1992 Daly and co-workers reported the isolation of a new natural product, epibatidine. Future studies showed that epibatidine was an nAChR ligand with analgesic potency 200-400 times greater than that of morphine. However, its potential as a new drug was limited by its toxic side effects, probably resulting from its activity at a number of nAChR subtypes. Epibatidine's unique structure and potent activity made it an ideal lead structure for the development of nAChR ligands with reduced side effects and better nAChR subtype selectivity. This review presents the synthetic methods we have used to synthesize a number of epibatidine agonists, antagonists, and mixed agonists/antagonists to better characterize the α4b2 nAChR pharmacophore and hopefully provide compounds that have potential for treating nicotine addiction.In 1992 Daly and co-workers were able to isolate trace amounts of a natural product from the skin of the Ecuadorian poison frog Epipedobates tricolor that was found to be 200-400 times more potent than morphine as an analgesic.1 The analgesic activity was not blocked by naloxone, showing that the activity was not due to interaction with opioid receptors. Subsequent studies showed that the analgesic activity resulted from interaction with nicotinic acetylcholine receptors (nAChRs).2, 3 Daly reviewed these early studies.4 Epibatidine (1a) has a 7-azabicyclo[2.2.1]heptane structure to which is attached an exo-5-(2'-chloropyridinyl) substituent. Fletcher and co-workers5 showed that the natural alkaloid possessed the (1R,2R,4S)-stereochemistry. Epibatidine (1a) and its enantiomer possess negative and positive rotations of the plane of polarized light, respectively (Figure 1). However, salts showed opposite signs of rotation from the free bases.
The unique structure and biological activity of epibatidine generated considerable interest in this compound and precipitated the development of numerous routes for its synthesis from simple starting materials. A number of these syntheses have been detailed in prior reviews.6-13 In this review, we report the synthetic methods we used to prepare epibatidine as well as a number of epibatidine analogs that were used in a structure activity relationship (SAR) study to help characterize the pharmacophores for nAChRs and hopefully lead to new pharmacotherapies for treating nicotine addiction.
SYNTHESIS OF EPIBATIDINE
Our first synthesis of epibatidine was based on a synthesis reported by Huang and Shen14 (see Scheme 1).15 In this approach, the least substituted double bond of the cycloadduct 2, prepared by a method similar to that used by Huang and Shen to prepare the N-carbomethoxy analog, was selectively reduced using nickel boride to give 96% of 3. Desulfonation and concomitant reduction of 3 proceeded cleanly to give 65% of a 2:1 mixture of the endo and exo isomers 4 and 5. The endo isomer 4 was epimerized to the exo isomer 5 in 46% yield using the reported procedure of Fletcher and co-workers.5 Deprotection of the N-Boc epibatidine (5) with trifluoroacetic acid at room temperature gave 97% of racemic epibatidine as a white solid. Optical resolution of (±)-1 using di-p-toluoyltartaric acid gave (+)- and (-)-1a, which were converted to their hydrochloride salts.
Tritium labeled (+)- and (-)-norchloroepibatidine [(+)- and (-)-6] were synthesized by reductive tritiation of epibatidine (Scheme 2).16 These radiolabeled compounds proved useful for in vivo labeling of nAChRs.16
IMPROVED SYNTHESIS OF EPIBATIDINE AND 2’-SUBSTITUTED DESCHLORO¬EPIBATIDINE ANALOGS
In 2001 we reported an improved synthesis of 1a.17 The new route also proved useful for the synthesis of the 2’-substituted deschloroepibatidine analogs 1b-i (Scheme 3).17 Heating a solution of N-(tert-butoxycarbonyl)pyrrole (7)18 and p-tolylsulfonylacetylene19 at 80 °C yielded diene 8. Selective reduction of the 5,6-double bond using nickel boride15 in ethanol gave monoolefin 9. Originally, desulfonation of 9 to give 10 was carried out in 55% yield using 2.5% sodium amalgam in a 1:1 mixture of ethyl acetate and tert-butyl alcohol containing disodium hydrogen phosphate.20 Later we discovered that 9 could be converted to 10 in higher yield and on larger scale by adding tributyltin hydride to 9 in benzene containing 2,2'-azabisisobutyronitrile (AIBN) followed by treatment of the resulting addition product with tetrabutylammonium fluoride in tetrahydrofuran.21 Coupling of 10 with 2-amino-5-iodopyridine using palladium acetate as catalyst in dimethylformamide containing tetrabutylammonium chloride and potassium formate at 100 °C for 12 h provided 7-tert-butoxycarbonyl-exo-2-(2'-amino-5'-pyridinyl)-7-azabicyclo[2.2.1]heptane (11). Diazotization22 of 11 in pyridine containing 70% hydrogen fluoride effected conversion of the 2-amino group to a fluoro group and deprotection of the N-Boc group gave 46% of the 2’-fluoro analog 1b. Compound 1b could also be obtained by direct reductive Heck coupling of 2-fluoro-5-iodopyridine (obtained by diazotization of 2-amino-5-iodopyridine19 in pyridine•HF) with 10 to give intermediate 12a, which gave the desired 1b on removal of the N-Boc-protecting group with trifluoroacetic acid. However, this route was less desirable since the overall yield was 39% and the coupling of 2-fluoro-5-iodopyridine with 10 took 4 days, whereas the coupling of 2-amino-5-iodopyridine with 10 was complete in 12 h. We also found that diazotization of 11 in hydrochloric acid in the presence or absence of cuprous chloride gave a 76% yield of epibatidine (1a) which was identical to an authentic sample, thus, providing an improved synthesis of 1a.15 Diazotization of 11 using sodium nitrite in hydrobromic acid containing bromine or acetic acid containing potassium carbonate gave the 2'-bromo analogs 1c and the N-tert-butoxycarbonyl 2'-hydroxy compound 12b, respectively. Treatment of 12b with trifluoromethanesulfonic anhydride gave the 2'-triflate 13. Diazotization of 11 with isoamyl nitrite in methylene iodide containing hydrogen iodide afforded the N-tert-butoxycarbonyl 2'-iodo compound 12c. Reductive Heck coupling of 10 with 3-iodopyridine yielded N-tert-butoxycarbonyl 2'-norchloro analog 12d. Reductive methylation of 11 with formaldehyde using sodium cyanoborohydride yielded the N-tert-butoxycarbonyl 2'-dimethylamino analog 14. Treatment of 12b-d, 13, and 14 with trifluoroacetic acid yielded the 2'-substituted epibatidine analogs 1d, 1e, and lg-i. Treatment of 11 with methanolic hydrogen chloride provided 1f.
[18F]-2’-Fluorodeschloroepibatidine and [18F]-N-methyl-2’-fluorodeschloroepibatidine proved useful as positron emission tomography (PET) imaging agents.22-25 Scheme 4 outlines the method used to synthesize these two PET ligands. Methylation of 14 with iodomethane affords the 2’-(N,N,N-trimethylammonium) analog 15.20 Nucleophilic heteroaromatic substituents of 15, using no-carrier added potassium [18F]fluoride followed by deprotection with trifluoroacetic acid, afforded [18F]-2’-fluorodeschloroepibatidine ([18F]-1b).26 Reductive N-methylation with formaldehyde and sodium cyanoborohydride yielded [18F]-N-methyl-2’-fluorodeschloroepibatidine ([18F]-16).26
SYNTHESIS OF 2’,3’-DISUBSTITUTED DESCHLOROEPIBATIDINE ANALOGS
As a continuation of our SAR studies, we synthesized 2’,3’-disubstituted deschloroepibatidine analogs 17a-n (Figure 2).27
Scheme 5 outlines the synthesis of the intermediates 18-20, which were used to prepare target compounds 17a-n. The reductive palladium acetate-catalyzed addition of 3-amino-2-fluoro-5-iodopyridine (21a), 3-amino-2-chloro-5-iodopyridine (21b), and 2-amino-3,5-diidopyridine (21c) to 7-tert-butoxy¬carbonyl-7-azabicyclo[2.2.1]hept-2-ene (10) in dimethylformamide (DMF) containing tetrabutyl¬ammonium chloride and potassium formate at 100 °C provided the intermediates 18-20.
Scheme 6 outlines the routes used to prepare tert-butoxycarbonyl intermediates 22-26 and their conversion to target compounds 17a–n. Bromination of 7-tert-butoxy¬carbonyl-2’-aminodeschloroepibatidine (11)20 using bromine in acetic acid provided intermediate 22. Diazotization of 18, 19, and 22 with isoamyl nitrite containing hydroiodic acid in methylene iodide gave 26, 25, and 23, respectively. Diazotization of 22 with tert-butyl nitrite in DMF yielded intermediate 24. Diazotization of 18 and 19 with sodium nitrite in pyridine containing 70% hydrogen fluoride-pyridine affected conversion of the 3-amino group to a fluoro group and deprotection of the N-tert-butoxycarbonyl group to give 17a,f, respectively. Diazotization of 18 and 19 with sodium nitrite in hydrochloric acid in the presence of cuprous chloride gave 17b,e, respectively. Diazotization of 22 with pyridine containing 70% hydrogen fluoride-pyridine provided 17c. Diazotization of 22 using sodium nitrite in hydrochloric acid in the presence of copper (I) chloride or hydrobromic acid in the presence of copper (I) bromide yielded 17g and 17j, respectively. Treatment of 26, 25, 19, 23, 24, 22, and 20 with trifluoroacetic acid provided 17d,h,i,k-n, respectively.
SYNTHESIS OF 3’-SUBSTITUTED DESCHLOROEPIBATIDINE ANALOGS
In efforts to further characterize the nAChR pharmacophore, we synthesized 3’-substituted deschloroepibatidine analogs 27a-g (Figure 3).28
Scheme 7 outlines the synthesis of 3'-substituted deschloroepibatidine analogs of 27a,b,d,e. Catalytic reductive dechlorination of 3'-aminoepibatidine (17i) using 10% palladium on carbon catalyst in ethanol provided the intermediate 7-tert-butoxycarbonyl-3'-aminodeschloroepibatidine (28). Diazotization of 28 with sodium nitrite in pyridine containing 70% hydrogen fluoride-pyridine affected conversion of the 3'-amino group to a fluoro group and deprotection of the N-tert-butoxycarbonyl group to give the 3'-fluoro analog 27a. Diazotization of 28 with sodium nitrite in hydrochloric acid in the presence of cuprous chloride yielded the 3'-chloro analog 27b. Diazotization of 28 with isoamyl nitrite containing hydroioidic acid in methylene iodide gave the 3'-iodo intermediate 29. Treatment of 28 and 29 with trifluoroacetic acid provided the 3'-amino and 3'-iodo analogs 27e and 27d, respectively. Resolution of 27d with (+)- and (-)-di-p-toluoyl-tartaric acid afforded (-)- and (+)-27d.
The 3'-bromo-(27c), 3'-vinyl-(27f), and the 3'-ethynyl-(27g) analogs were prepared as shown in Scheme 8. Reductive palladium acetate-catalyzed addition of 3,5-dibromopyridine to 7-tert-butoxy¬carbonyl-7-azabicyclo[2.2.1]hept-2-ene (10) in DMF containing tetrabutylammonium chloride and potassium formate at 80 °C provided the intermediate 7-tert-butoxy¬carbonyl-3’-bromodeschloroepibatidine (30). Coupling of 30 with vinyltributyltin, catalyzed by tris(dibenzylideneacetonyl)bis-palladium, in N-methyl-2-pyrrolidinone (NMP) containing tris(2-furyl)¬phosphine (TFP) at 80 °C afforded the 7-tert-butoxycarbonyl-protected 3'-vinyl intermediate 31. The reaction of 30 with trimethylsilylacetylene in the presence of a catalytic amount of copper (I) iodide and bis(triphenylphosphine)-palladium (II) chloride in degassed disopropylamine in a sealed tube at 50 °C produced 32. Removal of the silyl protecting group with tetrabutyl ammonium fluoride in THF afforded the tert-butoxycarbonyl 3'-ethynyl intermediate 33. Treatment of 30, 31, and 33 with trifluoroacetic acid in methylene chloride yielded the 3'-bromo, 3'-vinyl-, and 3'-ethynyl- analogs 27c,f,g, respectively.
The iodine-123 analog of 27d and [11C]-N-methyl-3’-iododeschloroepibatidine (35) have been shown to be useful single photon emission computed tomography (SPECT) and PET agents for in vivo imaging of α4β2 nAChRs.29, 30 The synthesis of these two compounds is shown in Scheme 929 and Scheme 10,30 respectively. Treatment of 29 with hexamethylditin in the presence of tetrakis(triphenylphosphine)palladium (0) yielded the trimethyltin precursor 34. Reaction of iodine-123 sodium iodide with 34 in the presence of chloramine-T followed by deprotection with trifluoroacetic acid afforded [123I]-27d. Treatment of 27d with [11C]methyliodide in dimethylforamide gives [11C]-35.
SYNTHESIS OF 2’-FLUORO-3’-(SUBSTITUTED PHENYL)DESCHLOROEPIBATIDINE ANALOGS
In a preliminary study we found that 2’-fluoro-3’-phenyldeschloroepibatidine (36a) (Figure 4) was, to our knowledge, the first epibatidine analog reported to show nAChR antagonist properties.31 In order to further develop this finding, compounds 36b-k (Figure 4) were synthesized and evaluated.32
The synthesis of 36a and 36k is outlined in Scheme 11. Palladium acetate-catalyzed reaction of 22 with phenylboronic acid or 3-methoxyphenylboronic acid in dimethoxyethane (DME) in the presence of tri-(o-tolyl)phosphine and sodium carbonate gave the tert-butoxycarbonyl-protected 2'-amino-3'-phenyl analog 37a and 37b, respectively. Diazotization of 37a,b using sodium nitrite in pyridine containing 70% hydrogen fluoride/pyridine yielded the desired compounds 36a and 36k, respectively. Resolution of 36a using (+)- and (-)-di-p-toluoyltartaric acid afforded (+)- and (-)-36a. After completion of the synthesis of 36a and 37k, we discovered that the palladium catalyzed coupling of arylboronic acids could be carried out without protecting the azabicyclo[2.2.1]heptane amino group. This provided a more efficient route to compounds 36b-j, which is outlined in Scheme 12. Palladium acetate catalyzed reaction of 17c with the appropriate 3- or 4-substituted phenyboronic acid (38) in dimethoxyethane (DME) in the presence of tri(o-tolyl)phosphine and sodium carbonate gave the desired compounds 36b–h and 36j. Reduction of the 4'-nitro analog 36g using iron and hydrochloride acid afforded the 4'-amino analog 36i.
It was particularly interesting to note that compounds 36a-k possessed varying degrees of nAChR agonist and antagonist properties in studies using mice.32
SYNTHESIS OF 3’-(SUBSTITUTED PHENYL)DESCHLOROEPIBATIDINE ANALOGS
In a continuation of our SAR studies to identify compounds having antagonist and mixed agonist/antagonist properties, we synthesized the 3’-(substituted phenyl)deschloroepibatidine analogs 39a-j (Figure 5).
The synthesis of 39a-h is shown in Scheme 13.33 Palladium acetate catalyzed coupling of tert-butoxycarbonyl-3'-bromodeschloroepibatidine (30) with the appropriately substituted phenylboronic acid in dimethoxyethane (DME) in the presence of tri-(o-tolyl)phosphine and sodium carbonate gave the tert-butoxycarbonyl-protected 3'-(substituted phenyl)deschloroepibatdine analogs (40a-g). Reduction of the 4-nitrophenyl intermediate 40f with iron powder in hydrochloric acid gave the 4-aminophenyl compound 41. Treatment of 40a-g and 41 with trifluoroacetic acid in methylene chloride removed the protecting tert-butoxycarbonyl group and afforded the desired 3-(substituted phenyl)deschloroepibatidine analogs 39a-h.
The 3'-(3-aminophenyl)- and 3'-(3-methoxyphenyl)deschloroepibatidine analogs 39i and 39j, respectively, were synthesized as outline in Scheme 14 before the more efficient synthesis used for 39a-g was developed. Palladium acetate catalyzed addition of 3-nitrophenylboronic acid or 3-methoxyphenylboronic acid to 7-tert-butoxy¬carbonyl-2’-amino-3’-bromodeschloroepibatidine (22) provided the tert-butoxy¬carbonyl-protected 3'-(3-nitrophenyl)- and 3'-(3-methoxyphenyl)deschloroepibatidine analogs 42a and 42b, respectively. Diazotization of 42a and 42b using sodium nitrite in hydrochloric acid yielded the 3'-(3-methoxyphenyl)epibatidine analog 43a and the 3’-(3’-nitrophenyl)epibatidine analog 43b, respectively. Catalytic hydrogenation of 43a and 43b using 10% palladium on carbon catalyst in methanol yielded the desired 39i and 39j, respectively.
SYNTHESIS OF BRIDGED AND FUSED RING ANALOGS OF EPIBATIDINE
Since reduction of the conformational freedom of a lead compound like epibatidine can provide useful SAR information concerning the nAChR pharmacophore, we synthesized the conformationally restricted epibatidine analogs 44-49 (Figure 6).34
The fused ring epibatidine analogs 44 and 45 were synthesized as outlined in Scheme 15. 3-Pyridyne, generated by treating 4-triethylsilylpyridin-3-yl trifluoromethanesulfonate (50)35 with cesium fluoride in acetonitrile, was added to 7 to give 51. Catalytic hydrogenation of 51 using 10% palladium on carbon in ethyl acetate yielded 52. Treatment of 51 and 52 with hydrogen chloride in methanol provided the desired 3,4-pyridine fused ring epibatidine analogs 44 and 45, respectively. A totally different synthesis of 45 which involved sixteen steps and a very low overall yield has been reported.36
The 2,3-pyridine ring based analog 46 was synthesized by a procedure similar to that for 45 (Scheme 16).34 2-Pyridyne, generated by treating 3-trimethylsilylpyridin-2-yl trifluoromethanesulfonate (53)37 with cesium fluoride in acetonitrile, was added to 7 to give 54. Catalytic reduction of 54 in ethyl acetate using 10% palladium on carbon catalyst yielded 55. Removal of the tert-butoxycarbonyl protecting group using trifluoroacetic acid in methylene chloride provided 46.
The bridged epibatidine analog 47 was synthesized as shown in Scheme 17 starting with 2-amino-4-methylpyridine (56). Iodination of 56 using iodine in a periodic, sulfuric, acetic acid mixture afforded a 71% yield of 2-amino-5-iodo-4-methylpyridine (57).34
Reaction of 57 with m-chloroperbenzoic acid in acetone gave the N-oxide 58, which was isolated as the hydrochloride salt in 85% yield. Treatment of the hydrochloride salt of 58 with acetic anhydride in dioxane was expected to give the 4-acetoxymethyl or 4-hydroxymethyl compounds 59a or 59b, respectively. Surprisingly, 2-acetamido-4-chloromethyl-5-iodopyridine (59c) was isolated in 56% yield. Apparently, chloride ion displaced the acetoxy or hydroxy group from the expected 4-acetoxymethyl or 4-hydroxymethyl intermediate to give 59c. Alkylation of 7-azabicyclo[2.2.1]hept-2-ene (60), generated from tert-butoxycarbonyl-7-azabicyclo[2.2.1]hept-2-ene (10) using trimethylsilyl iodide in chloroform, with 59c provided the N-alkylated product 61 in 43% yield. Two possible approaches for the conversion of 61 to 62 were the Heck cyclization38-41 and a radical initiated cyclization.42, 43 We found that intramolecular cyclization of 61 using reductive Heck conditions similar to that used for intermolecular coupling20 (palladium diacetate, potassium formate, and tetrabutyl ammonium chloride in dimethylformamide at 90 °C) provided the hexahydro-7,10-methanopyrrolo-2-[1,2-b]-2,6-naphthyridine 62 in 45% yield. Hydrolysis of 62 using refluxing 3 N hydrochloric acid gave a 90% yield of the 3-amino analog 63. Diazotization of 63 using sodium nitrite in concentrated hydrochloric acid yielded the desired epibatidine analog 47 in 28% yield.
Bridged epibatidine analog 48 was synthesized from 2-amino-6-methylpyridine (64) by a set of reactions exactly analogous to those used to prepare analog 47 that proceeded through intermediate 65-70 (see Scheme 18).34 The yield in each step was similar to the analogous step in the synthesis of 47.
The 5,6-benzofusion ring epibatidine analog 49 was synthesized as outlined in Scheme 19.34 Benzyne, generated by treating 2-trimethylsilyl trifluoromethanesulfonate (71)44-46 with cesium fluoride in acetonitrile, was added to 7 to give 72. Subjection of 72 to reductive Heck conditions using 2-amino-5-iodopyridine gave 73. Diazotization of 73 using sodium nitrite in concentrated hydrochloride acid yielded the desired 49.
SYNTHESIS OF EXO-2-(2’-CHLORO-5-PYRIDINYL)-7-(ENDO AND EXO)-AMINOBICYCLO¬[2.2.1]HEPTANES
In one of our SAR studies, we reported the syntheses of epibatidine analogs where the nitrogen in the 7-azabicyclo[2.2.1]heptane ring was moved outside the ring (see structures 74 and 75, Figure 7).47
The synthesis of 74 is outlined in Scheme 20.47 The reductive palladium acetate catalyzed addition of 2-amino-5-iodopyridine to exo-7-hydroxybicyclo[2.2.1]heptane (76)48 in dimethylformamide (DMF) containing potassium formate and tetrabutylammonium chloride at 110 °C provided an 82% yield of less sterically hindered exo-2-(2'-amino-5'-pyridinyl)-7-exo-hydroxybicyclo[2.2.1]heptane (77).49,50 Diazotization of 77 using sodium nitrite in concentrated hydrochloric acid afforded the 2'-chloro compound (78) in a 73% yield. Oxidation of 78 using pyridine sulfur trioxide complex in DMSO provided a 50% yield of the 7-oxo compound 79. Potassium carbonate catalyzed condensation of 79 with methoxylamine in ethanol provided an 84% yield of the O-methyloxime compound 80. Reduction of 80 from the least hindered face with diborane in tetrahydrofuran afforded exo-2-(2'-chloro-5'-pyridinyl)-7-endo-aminobicyclo[2.2.1]heptane (74) in 32% yield.
We were able to obtain 75 by the route shown in Scheme 21.47 Swern oxidation of 76 using oxalyl chloride, dimethyl sulfoxide, and triethylamine gave ketone 81 in 70% yield. Treatment of 81 with hydroxylamine hydrochloride in aqueous methanol containing sodium acetate provided a 36% yield of oxime 82. Lithium aluminum hydride reduction of 82 followed by acetylation gave the N-acetamido protected intermediate 83 (69%). Reductive palladium acetate catalyzed addition of 2-amino-5-iodopyridine to 83 in DMF containing potassium formate and tetrabutylammonium chloride yielded 20% of the less sterically hindered exo-2-(2'-amino-5’-pyridinyl)-7-exo- acetamidobicyclo[2.2.1]heptane (84).49,50 Diazotization of 84 using sodium nitrite in concentrated hydrochloric acid afforded a 40% yield of 2'-chloro compound 85. Removal of the protecting acetyl group from 85 using potassium hydroxide in ethylene glycol at 150 °C provided the desired 75 in 73% yield.
SUMMARY
In this review, synthetic methods used to prepare a number of different types of epibatidine analogs have been presented. In addition, the epibatidine analogs have been evaluated for their biological activity. For example, Ki values for the inhibition of [3H]epibatidine binding at α,β-nAChR in male rat cerebral cortex and inhibition of binding to the α7 nAChR using [125I]iodoMLA for the epibatidine analogs were determined and compared to epibatidine (1a), nicotine, and the α4β2 mixed agonist/antagonist varenicline, which is now an approved drug for treating smokers.
The compounds were also evaluated for analgesic activity in the tail-flick and hot-plate tests as well as for spontaneous activity and hypothermia in the mouse. Compounds whose in vivo activity was less than that expected from their Ki values in the α,β-nAChR screens were evaluated for antagonism of nicotine-induced activity in the mouse test. The Ki values for the 2’-substituted deschloroepibatidine analogues 1b-d possessing electron withdrawing groups and the unsubstituted analogue 1i were essentially identical to that of epibatidine (1a).17 Analogues with electron releasing groups had higher Ki values than epibatidine (1a). A good correlation was found between Ki binding affinity and ED50 values in the tail flick test.17 The 2’,3’-disubstituted deschloroepibatidine analogues 17a-k and 17m-n and the 3’-substituted deschloroepibatidine analogues 27a-g had high affinity for the α4β2 nAChR.27,28 In contrast to the 2’-substituted deschloroepibatidine analogues, the agonist activity seen in the tail flick for 2’,3’-disubstituted or the 3’-substituted analogues did not correlate well with their binding affinity.27, 28 The 2’-chloro-3’-amino disubstituted analogue 17i and most of the 3’-substituted analogs 27a-g were found to have both agonist and antagonist properties in the mouse tests.27, 28 Several epibatidine analogs from the 2’-fluoro-3-(substituted phenyl)deschloroepibatidine (36a-k) and the 3’-(substituted phenyl)deschloroepibatidine (39a-j) classes possessed high affinity for the α4β2 nAChR and showed varying degrees of mixed agonist/antagonist activity in the mouse functional test, some with activity similar to varenicline.32, 33 Future nicotine drug discrimination (DD), condition place preference (CPP), and self-administration (SE) studies may suggest that one or more of these analogs should be considered for development as a pharmacotherapy for treating nicotine addiction.
All bridged and fused ring analogues of epibatidine possessed low nAChR affinity.34 The binding affinity and in vivo functional activity of the (endo and exo)-aminobicyclo[2.2.1]heptane analogs 74 and 75 were comparable to those of nicotine.47
ACKNOWLEDGEMENTS
The research reported in this review was supported under NIDA grant DA12002.
References
1. T. F. Spande, H. M. Garraffo, M. W. Edwards, H. J. C. Yeh, L. Pannell, and J. W. Daly, J. Am. Chem. Soc., 1992, 114, 3475. CrossRef
2. B. Badio, D. Shi, M. Garraffo, and J. W. Daly, Drug Dev. Res., 1995, 36, 46. CrossRef
3. M. I. Damaj, K. R. Creasy, A. D. Grove, J. A. Rosecrans, and B. R. Martin, Brain Res., 1994, 664, 34. CrossRef
4. J. W. Daly, Cell. Mol. Neurobiol., 2005, 25, 513. CrossRef
5. S. R. Fletcher, R. Baker, M. S. Chambers, R. H. Herbert, S. C. Hobbs, S. R. Thomas, H. M. Verrier, A. P. Watt, and R. G. Ball, J. Org. Chem., 1994, 59, 1771. CrossRef
6. C. A. Broka, Med. Chem. Res., 1994, 4, 449.
7. C. Szantay, Z. Kardos-Balogh, and C. Szantay, Jr., ‘The Alkaloids,’ Vol. 46, Academic Press, 1995, pp. 95–125.
8. Z. Chen and M. L. Trudell, Chem. Rev., 1996, 96, 1179. CrossRef
9. D. Bai, R. Xu, and X. Zhu, Drugs Future, 1997, 22, 1210.
10. D. Bai, R. Xu, G. Chu, and X. Zhu, J. Org. Chem., 1996, 61, 4600. CrossRef
11. H. F. Olivo and M. S. Hemenway, Org. Prep. Proced. Int.
12. S. R. Breining, Current topics in medicinal chemistry, 2004, 4, 609. CrossRef
13. J.-E. Backvall, Pure and Applied Chemistry, 1999, 71, 1065. CrossRef
14. D. F. Huang and T. Y. Shen, Tetrahedron Lett., 1993, 34, 4477. CrossRef
15. P. L. Kotian and F. I. Carroll, Synth. Commun., 1995, 25, 63. CrossRef
16. U. Scheffel, G. F. Taylor, J. A. Kepler, F. I. Carroll, and M. J. Kuhar, NeuroReport, 1995, 6, 2483. CrossRef
17. F. I. Carroll, F. Liang, H. A. Navarro, L. E. Brieaddy, P. Abraham, M. I. Damaj, and B. R. Martin, J. Med. Chem., 2001, 44, 2229. CrossRef
18. E. Albertini, A. Barco, S. Benetti, C. De Risi, G. P. Pollini, R. Romagnoli, and V. Zanirato, Tetrahedron Lett., 1994, 35, 9297. CrossRef
19. S. C. Clayton and A. C. Regan, Tetrahedron Lett., 1993, 34, 7493. CrossRef
20. F. Liang, H. A. Navarro, P. Abraham, P. Kotian, Y.-S. Ding, J. Fowler, N. Volkow, M. J. Kuhar, and F. I. Carroll, J. Med. Chem., 1997, 40, 2293. CrossRef
21. Lawrence E. Brieaddy, F. Liang, P. Abraham, Jeffrey R. Lee, and F. I. Carroll, Tetrahedron Lett., 1998, 39, 5321. CrossRef
22. Y.-S. Ding, S. J. Gatley, J. S. Fowler, N. D. Volkow, D. Aggarwal, J. Logan, S. L. Dewey, F. Liang, F. I. Carroll, and M. J. Kuhar, Synapse, 1996, 24, 403. CrossRef
23. P. E. Molina, Y. S. Ding, F. I. Carroll, F. Liang, N. D. Volkow, N. Pappas, M. Kuhar, N. Abumrad, S. J. Gatley, and J. S. Fowler, Nucl. Med. Biol., 1997, 24, 743. CrossRef
24. S. J. Gatley, Y.-S. Ding, D. Brady, A. N. Gifford, S. L. Dewey, F. I. Carroll, J. S. Fowler, and N. D. Volkow, Nucl. Med. Biol., 1998, 25, 449. CrossRef
25. Y.-S. Ding, P. E. Molina, J. S. Fowler, J. Logan, N. D. Volkow, M. J. Kuhar, and F. I. Carroll, Nucl. Med. Biol., 1999, 26, 139. CrossRef
26. Y.-S. Ding, F. Liang, J. S. Fowler, M. J. Kuhar, and F. I. Carroll, J. Label. Compds. Radiopharm., 1997, 39, 827. CrossRef
27. F. I. Carroll, J. R. Lee, H. A. Navarro, W. Ma, L. E. Brieaddy, P. Abraham, M. I. Damaj, and B. R. Martin, J. Med. Chem., 2002, 45, 4755. CrossRef
28. F. I. Carroll, W. Ma, Y. Yokota, J. R. Lee, L. E. Brieaddy, H. A. Navarro, M. I. Damaj, and B. R. Martin, J. Med. Chem., 2005, 48, 1221. CrossRef
29. G. Tamagnan, A. O. Koren, D. Alagille, J. Staley, I. Carroll, and J. Seibyl, J. Nucl. Med., 2007, 48, 129P.
30. Y. S. Ding, K. E. Kil, K. S. Lin, W. Ma, Y. Yokota, and I. F. Carroll, Bioorg. Med. Chem. Lett., 2006, 16, 1049. CrossRef
31. F. I. Carroll, J. R. Lee, H. A. Navarro, L. E. Brieaddy, P. Abraham, M. I. Damaj, and B. R. Martin, J. Med. Chem., 2001, 44, 4039. CrossRef
32. F. I. Carroll, R. Ware, L. E. Brieaddy, H. A. Navarro, M. I. Damaj, and B. R. Martin, J. Med. Chem., 2004, 47, 4588. CrossRef
33. F. I. Carroll, Y. Yokota, W. Ma, J. R. Lee, L. E. Brieaddy, J. P. Burgess, H. A. Navarro, M. I. Damaj, and B. R. Martin, Bioorg. Med. Chem., 2008, 16, 746. CrossRef
34. F. I. Carroll, T. P. Robinson, L. E. Brieaddy, R. N. Atkinson, S. W. Mascarella, M. I. Damaj, B. R. Martin, and H. A. Navarro, J. Med. Chem., 2007, 50, 6383. CrossRef
35. M. Tsukazaki and V. Snieckus, Heterocycles, 1992, 33, 533. CrossRef
36. S. C. Turner, Z. Hongbin, and H. Rapoport, J. Org. Chem., 2000, 65, 861. CrossRef
37. M. A. Walters and J. J. Shay, Synth. Commun., 1997, 27, 3573. CrossRef
38. A. de Meijere and F. E. Meyer, Angew. Chem., Int. Ed. Engl., 1994, 33, 2379. CrossRef
39. I. Ojima, M. Tzamarioudaki, Z. Li, and R. J. Donovan, Chem. Rev., 1996, 96, 635. CrossRef
40. L. Ripa and A. Hallberg, J. Org. Chem., 1997, 62, 595. CrossRef
41. A. P. Marchand and R. W. Allen, J. Org. Chem., 1975, 40, 2551. CrossRef
42. A. Hoepping, K. M. Johnson, C. George, J. Flippen-Anderson, and A. P. Kozikowski, J. Med. Chem., 2000, 43, 2064. CrossRef
43. C. P. Jasperse, D. P. Curran, and T. L. Fevig, Chem. Rev., 1991, 91, 1237. CrossRef
44. K. P. Constable, B. E. Blough, and F. I. Carroll, J. Chem. Soc., Chem. Commun., 1996, 717. CrossRef
45. Y. Himeshima, T. Sonoda, and H. Kobayashi, Chem. Lett., 1983, 12, 1211. CrossRef
46. K. Shankaran and V. Snieckus, Tetrahedron Lett., 1984, 25, 2827. CrossRef
47. F. I. Carroll, L. E. Brieaddy, H. A. Navarro, M. I. Damaj, and B. R. Martin, J. Med. Chem., 2005, 48, 7491. CrossRef
48. P. R. Story, J. Org. Chem., 1961, 26, 287. CrossRef
49. A. Arcadi, F. Marinelli, E. Bernocchi, S. Cacchi, and G. Ortar, J. Organometal. Chem., 1989, 368, 249. CrossRef
50. R. C. Larock and P. L. Johnson, Chem. Commun., 1989, 1368. CrossRef