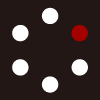
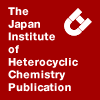
HETEROCYCLES
An International Journal for Reviews and Communications in Heterocyclic ChemistryWeb Edition ISSN: 1881-0942
Published online by The Japan Institute of Heterocyclic Chemistry
e-Journal
Full Text HTML
Received, 22nd September, 2008, Accepted, 5th November, 2008, Published online, 6th November, 2008.
DOI: 10.3987/COM-08-S(D)26
■ A New Trimeric Hydrolyzable Tannin, Oenotherin T2, Isolated from Aerial Parts of Oenothera tetraptera Cav.
Shoko Taniguchi, Yoko Imayoshi, Takashi Yoshida, and Tsutomu Hatano*
Graduate School of Medicine, Dentistry and Pharmaceutical Sciences, Okayama University, 1-1-1 Tsushima-naka, Okayama 700-8530, Japan
Abstract
A new hydrolyzable tannin, oenotherin T2, was isolated from the aerial parts of Oenothera tetraptera Cav., together with 15 known polyphenolic compounds. The trimeric structure of oenotherin T2 was elucidated based on spectroscopic data and chemical correlation with oenotherin T1.INTRODUCTION
Plants of Oenothera species (Onagraceae) produce oligomeric hydrolyzable tannins with unique macrocyclic structures, such as oenotheins A (1)1 and B (2).2 These tannins were first isolated as the major constituents of Oenothera erythrosepala Borbas and O. biennis L., and were later found in species of Lythraceae.1,2 We then isolated oenotherin T1 (3), structurally related to 1, from the aerial parts of O. tetraptera Cav.3 This compound was also obtained as a product of callus tissues induced from a leaf of O. laciniata Hill.3 Among the hydrolyzable tannins examined, oligomeric ones with macrocyclic structures showed potent antitumor effects.4,5 These tannins also showed antiviral effects on herpes simplex virus (HSV)6 and human immunodeficiency virus (HIV).7,8 Thus, we further investigated on the constituents of Oenothera species and isolated an additional trimeric hydrolyzable tannin named oenotherin T2 (4) from O. tetraptera. This report describes the elucidation of the structure of this trimeric tannin and the isolation of the accompanied known polyphenolics.
RESULTS AND DISCUSSION
Isolation of polyphenolic compounds
Dried leaves and stems of O. tetraptera were homogenized in 70% acetone, and the concentrated filtrate from the homogenate was fractionated by Diaion HP-20 column chromatography.3 The eluate containing 40% MeOH from the column was purified by column chromatography on Toyopearl HW-40, MCI-gel CHP-20, and ODS-gel, and also by preparative HPLC, to yield the new compound, oenotherin T2 (4), as well as 15 known polyphenolic compounds: (+)-catechin,9 quercetin 3-O-β-D-glucuronide,10 myricetin 3-O-β-D-glucuronide,11 1,2,6-tri-O-galloyl-β-D-glucose,12,13 gemin D,14 tellimagrandin I,15,16 tellimagrandin II,15,16 geraniin,17 heterophylliin A,18 oenotherin T1 (3),3 oenothein A (1),1 oenothein B (2),2 woodfordin E (5),19 woodfordin F (6),19 and woodfordin I (7).19
Structure of oenotherin T2
Oenotherin T2 (4) was obtained as a pale yellow amorphous powder. The trimeric molecular formula, C101H70O65, was indicated by the [M + NH4]+ ion peak at m/z 2340 in the electrospray-ionization (ESI)-MS. The 1H-NMR spectrum of 4 (in acetone-d6 + D2O) was complicated by the appearance of four sets of signals due to the α,β-anomerization of the glucose cores. The spectrum showed signals from eight aromatic protons [δ 5.91–6.20 (2H), 6.34–6.41 (1H), 6.46–6.48 (1H), 6.59–6.66 (1H), 7.00–7.04 (1H), 7.23–7.28 (2H in total)] in addition to the protons of three galloyl groups [δ 6.92–6.98 (2H), 7.03–7.06 (2H), 7.16–7.19 (2H in total)]. The spectrum also displayed glucose protons, which overlapped heavily with each other, and also characteristic doublets of methylene protons [δ 2.84, 2.86, 2.88, (1H), 3.05, 3.12, 3.29, 3.30 (1H in total), oenotheriyl H-3] other than the glucose protons in the aliphatic region.
The presence of three glucose cores was indicated by the anomeric protons at δ 6.03, 6.00 (glucose-I, α-anomer), 4.28, 4.26 (glucose-II, β-anomer), 5.42, 5.31 (glucose III, α-anomer), 5.05, and 4.98 (glucose III, β-anomer), which were correlated with the anomeric carbons at δ 91.2 (glucose-I, α-anomer), 96.9 (glucose-II, β-anomer), 90.8, 90.6 (glucose III, α-anomer), 94.9, and 94.8 (glucose III, β-anomer), in the 1H-13C heteronuclear single-quantum correlation (HSQC) spectrum.
Combined with the 1H-NMR spectral data, the presence of three galloyl groups, one hexahydroxydiphenoyl (HHDP), and one woodfordinoyl group in 4 was indicated by the 13C-NMR data (see experimental section). Although the 13C-NMR spectrum of oenotherin T2 (4) was similar to that of oenothein A (1),1 signals attributable to a new acyl group (named the oenotheriyl group) instead of those of the valoneoyl group in 1 were observed in the spectrum of 4 as follows: an oxygen-bearing quaternary carbon (δ 76.4–76.9, oenotheriyl C-2), a conjugated quaternary carbon (δ 128.3, 128.5, oenotheriyl C-2’), an oxygenated aromatic carbon (δ 147.8, oenotheriyl C-5), an ester carbonyl carbon (δ 171.5–171.7, oenotheriyl C-6), and a conjugated carbonyl carbon (δ 194.1–194.4, oenotheriyl C-4).
The heteronuclear multiple-bond correlation (HMBC) spectrum of 4 revealed the correlations of oenotheriyl H-3 with C-2, C-4, C-5, and C-6 (Figure 3). These correlations, in combination with MS data, suggested that the oenotheriyl group possesses a structure with a five-membered ring, as shown in formula 4.
Methylation of oenotherin T2 (4) with diazomethane and subsequent methanolysis gave methyl tri-O-methylgallate (8), dimethyl hexamethoxydiphenate (9), tetramethyl deca-O-methylwoodfordinate (10), and a product derived from the new acyl group (11). The production of 8, 9, and 10 from 4 was compared to that of an analogous treatment of oenothein A (1),3 indicating a molar ratio of 3:1:1 for 8:9:10 (Scheme 1). The strong positive Cotton effects at [θ]238 +1.77 × 105 and [θ]219 +3.18 × 105 in the CD spectrum of 4 suggested the S-configuration of the HHDP and woodfordinoyl groups.20
The structure of 11 was further investigated. The high resolution ESI-MS of 11 indicated its molecular formula, C28H28O14, based on the [M + H]+ ion peak. The 1H-NMR spectrum of 11 (in acetone-d6) showed two aromatic singlets [δ 7.39 (H-6”), 7.54 (H-3’)], eight 3H singlets arising from methoxyl groups [δ 3.55 (OCH3 at C-7’), 3.56 (OCH3 at C-7”), 3.57, 3.67 (OCH3 at C5’, C-3”), 3.77 (OCH3 at C-6), 3.88 (OCH3 at C-4”), (3.95 OCH3 at C-5”), 4.14 (OCH3 at C-5)], and a pair of geminal protons [δ 2.93, 3.17 (each 1H, d, J = 17.5 Hz, H-3)]. The 13C-NMR spectrum showed a conjugated carbonyl carbon (δ 191.9, C-4), three ester carbonyl carbons [δ 166.3 (C-7’), 167.3 (C-7”), 169.3 (C-6)], eight oxygenated aromatic carbons [δ 137.9 (C-5), 140.2 (C-2”), 141.1 (C-4’), 146.4 (C-4”), 147.8, 152.2 (C-5’, C-3”), 150.3 (C-6’), 153.5 (C-5”)], four quaternary aromatic carbons [δ 125.2, 126.1, 126.4 (C-1, C-1’, C-2’), 130.6 (C-1”)], and an oxygen-bearing quaternary carbon [δ 76.1 (C-2)], in addition to two aromatic methine carbons [δ 109.9 (C-6”), 114.1 (C-3’)], eight methoxy carbons [δ 51.9 (OCH3 at C-7’), 52.0 (OCH3 at C-7”), 54.1 (OCH3 at C-6), 56.3 (OCH3 at C-5”), 59.1 (OCH3 at C-5), 60.68, 60.74 (OCH3 at C-5’, C-3”), 60.9 (OCH3 at C-4”)], and a methylene carbon [δ 44.3 (C-3)]. The methoxy protons were correlated with three ester carbonyl carbons and five oxygenated aromatic carbons on the HMBC spectrum, as shown in Figure 4(a). HMBC correlations were also observed for H-3 with C-2, C-4, C-5, and C-6’. The correlation H3/C-6’ via four bonds indicated the presence of an ether linkage between C-2 and C-6’. This linkage was consistent with the molecular formula provided by the ESI-MS. Structure 11 for the new methylated acyl group was therefore assigned.
The CD spectrum of 11 showed a negative couplet at around 280 nm ([θ]307 –1.09 × 105, [θ]264 +4.13 × 104), corresponding to the S-configuration at C-2. This couplet is attributable to the helicity between the conjugated carbonyl system on the five-membered ring and the phenyl group, as shown in Figure 4(b).
Oenotherin T1 (3) is a trimeric hydrolyzable tannin having the isodehyrovaloneoyl group (IDV), in which a galloyl group and a dehydro-HHDP (DHHDP) group are linked via an ether oxygen (Figure 2). Heating a solution of oenotherin T1 (3) gave oenotherin T2 (4) (Scheme 1). The conversion of oenotherin T1 (3) to oenotherin T2 (4) may be explained by oxidative cleavage of the cyclohexene ring followed by a rearrangement, as shown in Scheme 2. Since 3 is abundant in the aerial parts of O. tetraptera, 4 might be formed from 3 in plant tissues.
Hydrolyzable tannins with an acyl group having analogous five-membered ring structures, such as repandinins A and B were previously reported,21,22 and these structures were assumed to be formed via an oxidative change of the DHHDP group.23
EXPERIMENTAL
General procedures. 1H- and 13C-NMR spectra were measured in acetone-d6 on a Varian INOVA AS600 spectrometer. Chemical shifts are given in δ (ppm) values relative to that of the solvent signals of acetone-d6 (δH 2.04; δC 29.8) on a tetramethylsilane scale. ESI-MS were recorded using a Micromass AutoSpec OA-Tof mass spectrometer with a solvent of 50% MeOH, 0.1% AcONH4. Optical rotations were measured on a JASCO DIP-1000 digital polarimeter, CD spectra were recorded on a JASCO J-720 spectrophotometer, and elemental analysis was performed on a Yanaco CHN recorder MT-5.
Extraction and isolation. The O. tetraptera plants used in this experiment were cultivated in the medicinal botanical garden of Okayama University Graduate School of Medicine, Dentistry and Pharmaceutical Sciences. Dried leaves and stems (245 g) of O. tetraptera were extracted with 70% acetone, and the extract was subjected to column chromatography using Diaion HP-20 (Mitsubishi Chemical).3 A portion (1.8 g) of the 40% MeOH eluate (14.9 g) from the column was chromatographed on a column of Toyopearl HW-40 (Tosoh) with 70% EtOH and then with EtOH–H2O–acetone (9:2:1), to give (+)-catechin (2.6 mg), 1,2,6-tri-O-galloyl-β-D-glucose (4.0 mg), gemin D (10.1 mg), tellimagrandin I (25.2 mg), tellimagrandin II (38.0 mg), oenothein B (2) (105.6 mg), oenotherin T1 (3) (101.5 mg), and oenothein A (1) (297.1 mg), along with a flavonoid fraction and a tannin fraction. The tannin fraction was purified on a Sep-Pak C18 cartridge (Waters) to give geraniin (4.0 mg). The flavonoid fraction was further purified on a Sep-Pak C18 cartridge and by preparative HPLC to give quercetin 3-O-β-D-glucuronide (3.0 mg) and myricetin 3-O-β-D-glucuronide (0.9 mg). In a separate experiment, the 40% MeOH eluate (5.0 g) from the Diaion HP-20 column was purified by column chromatography on Toyopearl HW-40, MCI-gel CHP-20P (Mitsubishi Chemical), and YMC-gel ODS AQ 120-S-50 (YMC) and by preparative HPLC to give 1,2,6-tri-O-galloyl-β-D-glucose (20.5 mg), tellimagrandin I (37.3 mg), oenothein A (1) (1.07 g), oenotherin T2 (4) (93.4 mg), woodfordin E (5) (23.1 mg), woodfordin I (7) (3.1 mg), woodfordin F (6) (199.9 mg), and heterophylliin A (0.8 mg).
Oenotherin T2 (4). A pale yellow powder, [α]D16 +68.4 (c 0.5, MeOH). ESI–MS m/z: 2340 [M+NH4]+. Anal. Calcd. for C101H70O65•16H2O: C, 46.5; H, 3.9%, Found: C, 46.4; H, 3.9%. UV λ max (MeOH) nm (log ε): 220 (5.84), 271 (5.53). CD (MeOH): [θ]314 –7.72 × 104, [θ]279 +1.26 × 105, [θ]258 +2.28 × 103, [θ]238 +1.77 × 105, [θ]219 +3.18 × 105. 13C-NMR (150 MHz, acetone-d6+D2O) δ: 45.9–46.0 (oenotheriyl C-3), 63.5, 64.2–64.3, 64.9, 65.5 (glucose C-6), 66.7–66.8, 69.0, 70.9–73.0, 73.7–75.6 (glucose C-2, C-3, C-4, C-5), 76.4–76.9 (oenotheriyl C-2), 90.6–91.2 [glucose C-1(α)], 94.8–96.0 [glucose C-1(β)], 102.8, 103.7, 106.1–108.1, 109.6–110.9 (galloyl C-2, C-6, HHDP C-3, C-3’, oenotheriyl C-3’, C-6”, woodfordinoyl C-6, C-3’, C-3”, C-6”’), 113.4–114.8, 115.5–116.8, 119.4–121.4 (galloyl C-1, HHDP C-1, C-1’, oenotheriyl, C-1, C-1’, C-1”, woodfordinoyl C-1, C-1’, C-1”, C-1”’), 125.2–126.3 (HHDP C-2, C-2’, woodfordinoyl C-2’, C-2”), 128.3, 128.5 (oenotheriyl C-2’), 133.3–133.4, 135.8–137.4, 138.5–141.0, 142.8–143.4, 144.2–145.7 (galloyl C-3, C-4, C-5, HHDP C-4, C-5, C-6, C-4’, C-5’, C-6’, oenotheriyl C-5’, C-6’, C-2”, C-3”, C-4”, C-5”, woodfordinoyl C-2, C-3, C-4, C-5, C-5’, C-6’, C-5”, C-6”, C-2”’, C-3”’, C-4”’, C-5”’), 146.4–147.0 (oenotheriyl C-4’, woodfordinoyl C-4’, C-4”), 147.8 (oenotheriyl C-5), 164.4–165.0, 167.1–169.6 (galloyl C-7, HHDP C-7, C-7’, oenotheriyl C-7’, C-7”, woodfordinoyl C-7, C-7’, C-7”, C-7”’), 171.5–171.7 (oenotheriyl C-6), 194.1, 194.3, 194.4 (oenotheriyl C-4).
Methylation of 4 followed by methanolysis. Compound 4 (10 mg) in EtOH (0.5 mL) was treated with CH2N2–Et2O for 1 h. After removal of the solvent, the residue was treated with 0.2% NaOMe in MeOH (0.1 mL) at rt for 6 h. The residue was partitioned between EtOAc and H2O, and the solvent of the EtOAc layer was removed in vacuo. The residue was further treated with CH2N2–Et2O. The product mixture obtained from 90 mg of 4 was combined and subjected to preparative TLC over Kieselgel PF254 (Merck) with n-hexane–CHCl3–acetone (6:3:1), to give 8 (18.8 mg), 9 (7.1 mg), 10 (10.3 mg) and a product mixture (1.3 mg). The mixture was purified by preparative HPLC (column, YMC-pack ODS A-303, 4.6 × 250 mm; detection, 280 nm; solvent, 58% MeOH) to give 11 (0.5 mg) as a white powder, [α]D27 –180.1 (c 0.2, MeOH). ESI-MS m/z: 589 [M+H]+, 606 [M+NH4]+, 611 [M+Na]+. HRE-ESI-MS m/z: 589.1578 [M+H]+ (C28H28O14 + H, 589.1557). UV λ max (MeOH) nm (log ε): 220 (4.76), 260 (4.59), 280 (infl.). CD (MeOH): [θ]307 –1.09 × 105, [θ]264 +4.13 × 104, [θ]248 +2.40 × 103, [θ]229 +1.28 × 105. The identification of compounds 9 and 10 was based on comparisons of the 1H-NMR spectral data with those of authentic specimens.3
Quantitative analysis of the constituent polyphenolic acids of 4. An ethereal CH2N2 (1 mL) was added to an EtOH solution (0.1 mL) of 4 (1 mg) and the mixture was left to stand for 2 h. After removal of the solvent under a N2 stream, the residue was treated with 0.2% NaOMe in MeOH (1 mL) overnight at rt. The reaction mixture was then acidified with 10% HCl and the solvent was removed by evaporation in vacuo. The reaction mixture was analyzed by HPLC [column, YMC-pack SIL A-003, 4.6 × 250 mm; solvent, n-hexane–EtOAc (2:1)] to show the presence of 8 (Rt 3.7 min), 9 (Rt 7.3 min), and 10 (Rt 29.1 min) in the mixture. The amounts of the products were estimated based on the comparison of the peak areas of 8, 9 and 10 obtained by an analogous treatment of oenothein A (1).
Conversion of 3 to 4. An aqueous solution (2 mL) of 3 (20 mg) was heated in a boiling water bath for 2.5 h. The solution was then passed through a Sep-Pak C18 cartridge. The adsorbed materials were eluted successively with H2O, followed by 10%, 20%, 30%, and 40% MeOH. The eluates of 20% and 30% MeOH were combined and purified by preparative HPLC [column, YMC-pack ODS A-324, 10 × 300 mm; solvent, 0.01 M H3PO4–0.01 M KH2PO4–EtOH–EtOAc (43:43:9:5)] to give 4 (1.7 mg), which was identified based on co-HPLC, [α]D (+64.5, MeOH) and the 1H-NMR spectral comparison.
ACKNOWLEDGMENTS
The Varian INOVA 600AS NMR instrument used in this study is the property of the SC-NMR laboratory of Okayama University, Japan.
References
1. T. Yoshida, T. Chou, M. Matsuda, T. Yasuhara, K. Yazaki, T. Hatano, A. Nitta, and T. Okuda, Chem. Pharm. Bull., 1991, 39, 1157.
2. T. Hatano, T. Yasuhara, M. Matsuda, K. Yazaki, T. Yoshida, and T. Okuda, J. Chem. Soc., Perkin Trans. 1, 1990, 2735. CrossRef
3. S. Taniguchi, Y. Imayoshi, R. Yabu-uchi, H. Ito, T. Hatano, and T. Yoshida, Phytochemistry, 2002, 59, 191. CrossRef
4. K. Miyamoto, M. Nomura, T. Murayama, T. Furukawa, T. Hatano, T. Yoshida, R. Koshiura, and T. Okuda, Biol. Pharm. Bull., 1993, 16, 379.
5. K. Miyamoto, M. Nomura, M. Sasakura, E. Matsui, R. Koshiura, T. Murayama, T. Furukawa, T. Hatano, T. Yoshida, and T. Okuda, Jpn. J. Cancer Res., 1993, 84, 98.
6. K. Fukuchi, H. Sakagami, T. Okuda, T. Hatano, S. Tanuma, K. Kitajima, Y. Inoue, S. Inoue, S. Ichikawa, M. Nonoyama, and K. Konno, Antiviral Res., 1989, 11, 285. CrossRef
7. M. Asanaka, T. Kurimura, R. Koshiura, T. Okuda, M. Mori, and H. Yokoi, Fourth International Conference on Immunopharmacology Osaka, 1988, p. 35.
8. H. Nakashima, T. Murakami, N. Yamamoto, H. Sakagami, S.Tanuma, T. Hatano, T. Yoshida, and T. Okuda, Antiviral Res., 1992, 18, 91. CrossRef
9. Y. Cai, F. J. Evans, M. F. Roberts, J. D. Phillipson, M. H. Zenk, and Y. Y. Gleba, Phytochemistry, 1991, 30, 2033. CrossRef
10. M. A. M. Nawwar, A. M. A. Souleman, J. Buddrus, and M. Linscheid, Phytochemistry, 1984, 23, 2347. CrossRef
11. A. Hiermann, M. Reidlinger, H. Juan, and W. Sametz, Planta Med., 1991, 57, 357. CrossRef
12. T. Okuda, T. Hatano, K. Yazaki, and N. Ogawa, Chem. Pharm. Bull., 1982, 30, 230.
13. E. A. Haddock, R. K. Gupta, S. M. K. Al-Shafi, E. Haslam, and D. Magnolato, J. Chem. Soc., Perkin Trans. 1, 1982, 2515. CrossRef
14. T. Yoshida, Y. Maruyama, M. U. Memon, T. Shingu, and T. Okuda, Phytochemistry, 1985, 24, 1041. CrossRef
15. T. Okuda, T. Hatano, and T. Yasui, Heterocycles, 1981, 16, 1321. CrossRef
16. C. K. Wilkins and B. A. Bohm, Phytochemistry, 1976, 15, 211. CrossRef
17. T. Okuda, T. Yoshida, and T. Hatano, J. Chem. Soc., Perkin Trans. 1, 1982, 9. CrossRef
18. T. Yoshida, Z.-X. Jin, and T. Okuda, Chem. Pharm. Bull., 1991, 39, 49.
19. T. Yoshida, T. Chou, A. Nitta, and T. Okuda, Chem. Pharm. Bull., 1992, 40, 2023.
20. T. Okuda, T. Yoshida, T. Hatano, T. Koga, N. Toh, and K. Kuriyama, Tetrahedron Lett., 1982, 23, 3937. CrossRef
21. Y. Amakura, M. Miyake, H. Ito, S. Murakaku, S. Araki, Y. Itoh, C.-F. Lub, L.-L. Yangb, K.-Y. Yenb, T. Okuda, and T. Yoshida, Phytochemistry, 1999, 50, 667. CrossRef
22. M. Xu, Z.-J. Zha, X.-L. Qin, X.-L. Zhang, C.-T. Yang and Y.-J. Zhang, Chem. Biodivers., 2007, 4, 2246. CrossRef
23. T. Okuda, T. Yoshida, and T. Hatano, Phytochemistry, 2000, 55, 513. CrossRef