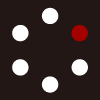
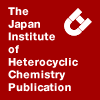
HETEROCYCLES
An International Journal for Reviews and Communications in Heterocyclic ChemistryWeb Edition ISSN: 1881-0942
Published online by The Japan Institute of Heterocyclic Chemistry
e-Journal
Full Text HTML
Received, 20th September, 2008, Accepted, 10th November, 2008, Published online, 11th November, 2008.
DOI: 10.3987/COM-08-11556
■ First Entry to [4+2] Cycloadditions Involving 5-Amino-2-furanmethanols
Raouf Medimagh, Sylvain Marque,* Damien Prim,* and Saber Chatti
Institut Lavoisier de Versailles, UMR CNRS 8180, University of Versailles-Saint-Quentin-en-Yvelines, 78035 Versailles Cedex, France
Abstract
The preparation of new 5-amino-2-furanmethanols bearing various amino and primary or secondary alcohol groups is described. The structures of 5-amino-2-furanmethanols as dienes are consistent with 1H NMR data and cyloadditions of them allows the selective synthesis of tetrasubstituted aminobenzylic alcohols, amino phenols or lactones through Diels–Alder reactions.INTRODUCTION
Furans and their derivatives are known to undergo [4+2] cycloadditions for at least one century. Such synthetic transformations represent one of the most popular ways to obtain six-membered ring systems. This area of intense research has been recently reviewed by Padwa and co-workers.1 Variously substituted furans undergo Diels-Alder cycloadditions and afford highly functionalized cycloadducts.2 Among these, 2-aminofurans emerged as powerful dienes allowing elegant accesses to structures with enhanced molecular complexity.3,4 We recently reported a metal-free amination of various heterocycles including furans,5 and addressed the ability of 5-amino-2-furancarboxaldehydes to afford polysubstituted anilines or oxabicyclic adducts through Diels–Alder cycloadditions (Table 1).6 Although various anilines and stable oxabicyclic adducts (entries 1 and 4) could be selectively obtained in high yields, this strategy suffered from the lack of reactivity of the diene and was depending on the nature of the dienophile. Indeed, lowering the reaction temperature from 110 to 70 °C led to dramatic decrease of isolated yields of Diels-Alder adduct. In the case of N-phenylmaleimide only traces of the corresponding aniline could be observed (compare entries 1 and 2). In addition, no reaction took place, when lesser reactive dienophiles, such as diethyl maleate, were used as partners in these cycloadditions (entry 3). Extension of this methodology to other dienophiles and thus the obtention of new polysubstituted aromatics require an increase of the substrate dienic character. Starting from this statement, we anticipated that moving from the carboxaldehyde to a alcohol moiety would increase the dienic character of 2-aminofurans and become beneficial to the diene reactivity. Such an investigation required the preparation of 5-amino-2-furanmethanols which have, to the best of our knowledge, not been previously reported.7
The focus of the present work begins with the preparation of new 5-amino-2-furanmethanols and is followed by a first evaluation of these new dienes in Diels–Alder reactions (Figure 1).
RESULTS AND DISCUSSION
We first investigated the preparation of alcohols 2-5 as described in Scheme 1. Although initial attempts allowed the formation of alcohol 2 in low yields, the latter revealed highly unstable under both classical purification methods (silica gel chromatography, crystallization) and storage conditions (low temperature, inert atmosphere). Similarly, such alcohols proved unstable and could not be purified. Extensive experimentations were required to obtain quantitative formation of 2. For example, careful and slow addition of 1.5 eq of lithium aluminium hydride (LAH) to the parent carboxaldehyde at 0 °C in THF was found essential. After 1 h at rt, the reaction mixture was treated with 1 M NaOH solution, extracted with diethyl ether and the solvent was evaporated. This procedure allowed a clean and quantitative preparation of crude alcohol 2 which could be used in Diels–Alder reactions. Secondary alcohols 3-5 were synthetized by nucleophilic addition of alkyl- or phenyl- magnesium bromide or chloride to the corresponding 5-amino-2-furancarboxaldehydes 1a-c. The optimized conditions allowed reaching various secondary alcohols obtained in nearly quantitative conversions without traces of by-products according to NMR data.
As already observed in the furan series,6 the overall reactivity, especially the dienic character may be evaluated by 1H NMR data. It is worth noting that chemical shifts in all 5-(morpholin-4-yl)-2-furanmethanols are close and confirm an overall increase of dienic character (Table 2).
Comparison of chemical shifts of the furan ring residual protons between 5-(morpholin-4-yl)-2-furancarboxaldehyde (1a) and 5-(morpholin-4-yl)-2-furanmethanols (2) is illustrated in Table 2. We anticipated that reducing the carboxaldehyde moiety in 1a into the corresponding hydroxymethyl group in 2, would further increase the dienic character and resulted in additional negative shieldings. Negative shieldings, observed for H-4 and especially H-3, from the parent 5-(morpholin-4-yl)-2-furancarboxaldehyde 1a to 2, resulted in a clear increase of dienic character and was attributed to a concomitant decrease of the electron withdrawing character of aldehyde moiety with an increase of the donating character of the alcohol group. Characteristic 1H NMR data for 5-amino-2-furanmethanols 3a, 5a and 5b confirmed this observation as depicted in Table 2.
Encouraged by the difference in 1H NMR data between 5-amino-2-furancarboxaldehydes and 5-amino-2-furanmethanols, we started to evaluate the latter as potential dienes in Diels-Alder reactions. With N-phenylmaleimide as dienophile, various amino benzylic primary and secondary alcohols were obtained in moderate to good yields (30-85% for two steps, Scheme 2).
When using moderate dienophile as diethyl maleate, the latter failed to react with 5-amino-2-furancarboxaldehydes 1. Interestingly, various substituted butyrolactones 12-14 were reached with modest yields, when reacting with 5-amino-2-furanmethanols (Scheme 3). The moderate overall yields were likely due to the instability of 5-amino-2-furanmethanols: control experiments with the last ones conducted in toluene at 70 or 110 °C, without dienophile afforded only degradation of the reactants 2 evidencing that modest yields arised from a low stability of the dienes in these conditions. The competitive thermal degradation of the diene 2 became the major process when acrylonitrile or naphthoquinone were used as dienophiles. Indeed, only traces of corresponding rearomatized cycloadducts could be identified in the crude material, whereas completed deacetylation of the cycloadduct was observed using the methyl vinyl ketone.
Moving from alkenic to alkynic dienophile such as dimethyl acetylene dicarboxylate (DMAD) afforded unexpected 4-aminophenols (Scheme 4).8
Surprisingly, phenol derivatives 10 and 11 were obtained through a concomittant rearrangement and rearomatization process, irrespective of the amino and primary (R3 = H) or secondary (R3 ≠ H) alcohol groups. Using 2, expected oxabicyclic adduct or benzylic alcohol were not observed. Attempts to vary the reaction temperature were unsuccessful. Indeed, at 50 °C, no reaction took place and at higher temperatures (110 °C) degradation of the furan was mainly observed. These results using DMAD with 2 are totally different of the Diels–Alder reactions carried out with 5-amino-2-furancarboxaldehydes 1 in our previous work where corresponding oxabicycle intermediates were isolated and relatively stable (Table 3).
CONCLUSIONS
In summary, we described the first preparation of 5-amino-2-furanmethanols. The presence of both amino and alcohol groups in positions 2 and 5 respectively, of the heterocycle induces a decrease of aromatic character, in good agreement with 1H NMR data. Table 3 evidences the major differences observed in cycloadditions involving 5-amino-2-furanmethanols by comparison with the parent carboxaldehydes, in terms of reaction temperature, range of reactive dienophiles, and target structures. Indeed, we succeeded in the selective preparation of tetrasubstituted aminobenzylic alcohols, 4-aminophenols and benzo-fused lactones through cycloaddition reactions.
EXPERIMENTAL
Unless otherwise noted, all starting materials were obtained from commercial suppliers and used without purification. Cyclohexane and petroleum ether were distilled under argon. Toluene was used without distillation. THF was distilled from sodium benzophenone ketyl under argon. All reactions were carried out under an atmosphere of dry argon. Solutions were evaporated under reduced pressure with a rotary evaporator and the residue was purified by silica gel chromatography using an ethyl acetate–cyclohexane mixture as the eluent unless specified otherwise. NMR spectra were recorded on a 300 MHz and 200 MHz Brucker spectrometer. Chemical shifts were reported in ppm relative to the residual solvent peak (7.26 ppm for CHCl3) for 1H spectra and (77.00 ppm for CDCl3) for 13C spectra. Chemical ionisation spectroscopy were recorded on a HP5989 B device of Hewlett-Packard ; for electrospray mass spectroscopy an add-on 59 987 A was used ; Branford source-type ; 4 µL/min. High Resolution Mass spectroscopy data were recorded on a Autospec Ultima (Waters/Micromass) device with a resolution of 5000 RP at 5%. Elemental analyses were performed at I.C.S.N. of Gif-sur-Yvette, France.
Procedure for 5-morpholinofur-2-ylmethanol (2)
To a stirred solution of 5-morpholino-2-furancarboxaldehyde (1a) (100 mg, 0.55 mmol) in THF (3 mL) was added 31.3 mg (0.825 mmol, 1.5 eq) of LiAlH4 at 0 °C. The solution was allowed to warm up at rt and stirred during 2 h. The reaction medium was quenched with aq. NaOH (1M) at 0 °C and stirred during 1 h at rt. The crude was filtred, washed with Et2O and dried over MgSO4. The combined organic layer was concentrated over reduced pressure to afford 102 mg (0.55 mmol, 100%) of the desired product 2 as yellow oil. 1H NMR (CDCl3, 200 MHz): δ 3.05–3.09 (m, 4H, NCH2), 3.75–3.79 (m, 4H, OCH2), 4.43 (s, 2H, CH2OH), 5.09 (d, 3J = 4.7 Hz, 1H, CH=CHCCN), 6.13 (d, 3J = 4.6 Hz, 1H, CH=CHCCH2OH).
General procedure for secondary alcohols:
1-(5-Morpholinofur-2-yl)ethanol (3a)
To a stirred solution of 5-morpholino-2-furancarboxaldehyde (1a) (100 mg, 0.55 mmol) in THF (10 mL) was added 0.3 mL (0.55 mmol, 1 eq) of methylmagnesium bromide (1.9 M in THF) at 0 °C. The solution was allowed to warm up at rt and stirred overnight. The reaction was quenched using a saturated aqueous solution of NH4Cl (1.5 mL) at 0 °C and then extracted with Et2O 3 × 15 mL. The combined organic layer was dried over MgSO4 and then concentrated under reduced pressure to afford 186 mg (0.55 mmol, 100%) of the desired product 3a as brown oil. 1H NMR (CDCl3, 200 MHz): δ 1.50 (d, 3J = 9.9 Hz, 3H, CHCH3OH), 1.80–1.88 (m, 1H, CHCH3OH), 3.07–3.12 (m, 4H, NCH2), 3.78–3.83 (m, 4H, OCH2), 4.92 (q, 3J = 9.8 Hz, 3H, CHCH3OH), 5.11 (d, 3J = 4.9 Hz, 1H, CH=CHCCN), 6.10 (d, 3J = 4.9 Hz, 1H, CH=CHCCH3OH).
1-(5-(N-Allyl-N-methylamino)fur-2-yl)ethanol (3b)
Following general procedure for secondary alcohols 1b (100 mg, 0.60 mmol) led to 3b as brown oil. 1H NMR (CDCl3, 200 MHz): δ 1.47 (d, 3J = 6.6 Hz, 3H, CHCH3OH), 2.74 (s, 3H, NMe), 3.73 (d, 3J = 6.1 Hz, 2H, NCH2CH=CH2), 4.74 (q, 3J = 6.4 Hz, 1H, CHMeOH), 4.94 (d, 3J = 3.1 Hz, 1H, CH=CHCCN), 5.14–5.25 (m, 2H, NCH2CH=CH2), 5.73–5.92 (m, 1H, NCH2CH=CH2), 6.08 (d, 3J = 3.1 Hz, 1H, CH=CHCCHOHMe).
1-(5-(N-4-Methoxyphenyl-N-methylamino)fur-2-yl)ethanol (3c)
Following general procedure for secondary alcohols 1c (150 mg, 0.64 mmol) led to 3c as brown oil. 1H NMR (acetone-d6, 200 MHz): δ 1.41 (d, 3J = 6.4 Hz, 3H, CHCH3OH), 3.19 (s, 3H, NCH3), 3.74 (s, 3H, OCH3), 4.67–4.73 (m, 1H, CHOHMe), 5.55 (d, 3J = 3.1 Hz, 1H, CH=CHCCN), 6.15 (d, 3J = 3.1 Hz, 1H, CH=CHCCHOHMe), 6.81–6.96 (m, 4H, Ph).
1-(5-Morpholinofur-2-yl)-1-phenylmethanol (4)
Following general procedure for secondary alcohols 1a (165 mg, 0.91 mmol) led to 4 as brown oil. 1H NMR (CDCl3, 200 MHz): δ 3.01–3.11 (m, 4H, NCH2), 3.75–3.82 (m, 4H, OCH2), 5.09 (d, 3J = 3.3 Hz, 1H, CH=CHCCN), 5.92 (d, 3J = 3.1 Hz, 1H, CH=CHCCHOHPh), 5.73 (d, 3J = 3.5 Hz, 1H, CHOHPh), 7.33–7.62 (m, 5H, Ph).
1-(5-Morpholinofur-2-yl)but-3-en-1-ol (5a)
Following general procedure for secondary alcohols 1a (75 mg, 0.41 mmol) led to 5a as brown oil. 1H NMR (CDCl3, 200 MHz): δ 2.59 (t, 3J = 6.8 Hz, 2H, CHCH2CH=CH), 3.02–3.12 (m, 4H, NCH2), 3.78–3.83 (m, 4H, OCH2), 4.62 (t, 3J = 6.6 Hz, 1H, CHOHAllyl), 5.08–5.21 (m, 2H, CH=CHCCN + CHCH2CH=CH2), 5.71–5.90 (m, 1H, CHCH2CH=CH2), 6.13 (d, 3J = 3.3 Hz, 1H, CH=CHCCHOHallyl).
1-(5-(N-Allyl-N-methylamino)fur-2-yl)but-3-en-1-ol (5b)
Following general procedure for secondary alcohols 1b (100 mg, 0.60 mmol) led to 5b as brown oil. 1H NMR (acetone-d6, 200 MHz): δ 2.64–2.70 (m, 2H, CHOHCH2CH=CH), 2.84 (s, 3H, NMe), 3.69–3.72 (m, 2H, NCH2CH=CH2), 4.96–5.26 (m, 5H, NCH2CH=CH2 + CHOHCH2CH=CH2 + CH=CHCCN), 5.75–5.90 (m, 3H, NCH2CH=CH2 + CHOHCH2CH=CH2 + CH=CHCCHOHallyl).
General procedure for cycloaddition
In a 10 mL round-bottom flask, 5-amino-2-furanmethanol (1 eq) and dienophile (3 eq) were stirred in toluene (3 mL) at 75 °C overnight. The crude was allowed to cool down at rt and concentrated under reduced pressure. The crude was purified by silica gel chromatography (cyclohexane:EtOAc).
(2,3-Dihydro-7-morpholino-1,3-dioxo-2-phenyl)-1H-isoindolin-4-ylmethanol (6)
Following general procedure of cycloaddition, 2 and N-phenylmaleimide led to 72.6 mg (62%) of desired product 6 after flash chromatography (cyclohexane:EtOAc 6:4) as yellow oil. 1H NMR (CDCl3, 300 MHz): δ 3.32–3.35 (m, 4H, NCH2), 3.91–3.96 (m, 5H, OCH2 and OH), 4.90 (d, 3J = 7.1 Hz, 2H, CH2OH), 7.18 (d, 3J = 8.5 Hz, 1H, CH=CHCN), 7.38–7.54 (m, 5H, Ph), 7.58 (d, 3J = 8.5 Hz, 1H, CH=CHCCH2OH); 13C NMR (CDCl3, 75 MHz): δ 51.5 (2C, NCH2), 62 (CH2OH), 66.9 (2C, OCH2), 118.3 (C), 123.3 (CH, CH=CHCN), 126.9 (CH, Ph), 128.3 (CH, Ph), 129.2 (CH, Ph), 130.8 (C), 131.4 (C), 133.5 (C), 135.9 (CH, CH=CHCCH2OH), 150.1 (C), 166.5 (C, CON), 168.8 (C, CON); CI-MS NH3 m/z (rel int): 321 (60, (MH+)-H2O), 339 (100, MH+); EsI-MS m/z (rel int): 361.3 (100, M+Na+), 699.3 (32, (2M)+Na+).
1-{(2,3-Dihydro-7-morpholino-1,3-dioxo-2-phenyl)-1H-isoindolin-4-yl}ethanol (7a)
Following general procedure for cycloaddition, 3a and N-phenylmaleimide led to 76.3 mg (80%) of the desired product 7a after flash chromatography (cyclohexane:EtOAc 65:35) as yellow oil. 1H NMR (CDCl3, 300 MHz): δ 1.58 (d, 3J = 6.7 Hz, 3H, CHOHMe), 3.30–3.34 (m, 4H, NCH2), 3.90–3.93 (m, 4H, OCH2), 4.17 (d, 3J = 7.5 Hz, 1H, OH), 5.26–5.35 (m, 1H, CHOHMe), 7.20 (d, 3J = 8.7 Hz, 1H, CH=CHCN), 7.38–7.54 (m, 5H, Ph), 7.65 (d, 3J = 8.7 Hz, 1H, CH=CHCCHOHMe); 13C NMR (CDCl3, 75 MHz): δ 23.7 (CH3), 51.6 (2C, NCH2), 66.9 (2C, OCH2), 67.4 (CHMe), 118.4 (C), 123.6 (CH, CH=CHCN), 126.9 (CH, Ph), 128.3 (CH, Ph), 129.2 (CH, Ph), 129.5 (C), 131.4 (C), 134.1 (CH, CH=CHCCH2OH), 138.9 (C), 149.7 (C), 166.4 (CON), 168.8 (CON); CI-MS NH3 m/z (rel int): 370 (45, M+NH4+), 306 (100); HRMS m/z calcd for C20H20N2O4 352.1423, found 352.1424 ± 0.0001; Anal. Calcd for C20H20N2O4.H2O: C 64.85, H 5.99, N 7.56. Found: C 64.52, H 5.71, N 7.14.
1-{(2,3-Dihydro-7-(N-allyl-N-methylamino)-1,3-dioxo-2-phenyl)-1H-isoindolin-4-yl}ethanol (7b)
Following general procedure of cycloaddition, 3b and N-phenylmaleimide led to 50.8 mg (30%) of desired product 7b after flash chromatography (petroleum ether:EtOAc 7:3) as yellow oil. 1H NMR (CDCl3, 300 MHz): δ 1.58 (d, 3J = 6.7 Hz, 3H, CHOHCH3), 3.01 (s, 3H, NCH3), 4.00 (d, 3J = 6.0 Hz, 2H, NCH2CH=CH2), 4.32 (d, 3J = 5.1 Hz, 1H, CHOHCH3), 5.16–5.26 (m, 3H, NCH2CH=CH2 + CHOHMe), 5.94–6.02 (m, 1H, NCH2CH=CH2), 7.16 (d, 3J = 9.0 Hz, 1H, N-HAr), 7.39–7.42 (m, 3H, Ph), 7.48–7.50 (m, 2H, Ph), 7.55 (d, 3J = 9.0 Hz, 1H, HArCHMeOH); 13C NMR (CDCl3, 75 MHz): δ 23.6 (CHOHCH3), 39.5 (NCH3), 59.1 (NCH2CH=CH2), 67.4 (CHOHCH3), 115.4 (C), 118.1 (NCH2CH=CH2), 123.7 (CHAr-N), 126.9 (CH, Ph), 128.1 (NCH2CH=CH2), 129.0 (CH, Ph), 129.5 (C), 131.6 (C), 133.6 (CH, Ph), 134.3 (CH, CHArCHOHMe), 136.9 (C), 149.4 (C), 166.3 (C, NCO), 169 (C, NCO); Anal. Calcd for 3(C20H20N2O3).H2O: C 70.16, H 6.08, N 8.18. Found: C 70.05, H 5.92, N 7.68.
1-{(2,3-Dihydro-7-(N-4-methoxyphenyl-N-methylamino)-1,3-dioxo-2-phenyl)-1H-isoindolin-4-yl}eth- anol (7c)
Following general procedure of cycloaddition, 3c and N-phenylmaleimide led to 104.0 mg (83%) of desired product 7c after flash chromatography (petroleum ether:EtOAc 8:2 to 7.5:2.5) as yellow oil. 1H NMR (CDCl3, 300 MHz): δ 1.59 (d, 3J = 6.7 Hz, 3H, CHOHCH3), 3.48 (s, 3H, NCH3), 3.79 (s, 3H, OCH3), 5.28 (q, 3J = 7.0 Hz, 1H, CHOHMe), 6.85 (d, 3J = 9.1 Hz, 2H, PhOMe), 7.03 (d, 3J = 9.0 Hz, 2H, PhOMe), 7.24 (d, 3J = 8.7 Hz, 1H, N-HAr), 7.38–7.41 (m, 3H, Ph), 7.46–7.51 (m, 3H, Ph + HArCHOHMe); 13C NMR (CDCl3, 75 MHz): δ 23.7 (CHOHCH3), 42.5 (NCH3), 56.5 (OCH3), 67.5 (CHOHCH3), 114.7 (CH, NMePhOMe), 118.9 (C), 122.5 (CH, NMePhOMe), 126.8 (CH, Ph), 128.1 (CH, CHAr-N), 129 (CH, Ph), 129.3 (CH, CHArCHMeOH), 129.4 (C), 131.6 (C), 133.2 (CH, Ph), 139.4 (C), 141.7 (C), 147.8 (C), 155.6 (C), 165.3 (C, NCO), 168.8 (C, NCO); Anal. Calcd for C24H22N2O4.0.5H2O: C 70.06, H 5.63, N 6.81. Found: C 69.46, H 5.56, N 6.86.
1-{(2,3-Dihydro-7-morpholino-1,3-dioxo-2-phenyl)-1H-isoindolin-4-yl}-1-phenylmethanol (8)
Following general procedure for cycloaddition, 4 and N-phenylmaleimide led to 46.5 mg (60%) of the desired product 8 after flash chromatography (cyclohexane:EtOAc 8:2 to 7:3) as a yellow oil. 1H NMR (CDCl3, 300 MHz): δ 3.31–3.33 (m, 4H, NCH2), 3.90–3.91 (m, 4H, OCH2), 4.30 (brs, OH), 6.44 (s, 1H, CHOHPh), 7.19 (d, 3J = 8.2 Hz, 1H, CH=CHCN), 7.34–7.50 (m, 5H, Ph), 7.62 (d, 3J = 7.6 Hz, 1H, CH=CHCCHOHPh); 13C NMR (CDCl3, 75 MHz): δ 51.5 (2C, NCH2), 66.9 (2C, OCH2), 71.9 (CHOHPh), 118.2 (C), 123.4 (CH, CH=CHCN), 126.5 (CH, Ph), 126.9 (CH, Ph), 127.6 (CH, Ph), 128 (CH, Ph), 128.4 (CH, Ph), 129.1 (C), 129.8 (C), 131.4 (C), 135.3 (CH, CH=CHCCHOHPh), 136.6 (C), 142.4 (C), 149.8 (C), 166.3 (CON), 168.5 (CON); CI-MS NH3 m/z (rel int): 397 (64, (MH+)-18), 415 (100, MH+); HRMS m/z calcd for C25H22N2O4 414.1580, found 414.1579 ± 0.0001.
1-{(2,3-Dihydro-7-morpholino-1,3-dioxo-2-phenyl)-1H-isoindolin-4-yl}but-3-en-1-ol (9)
Following general procedure of cycloaddition, 5a and N-phenylmaleimide led to 52.9 mg (35%) of desired product 9 after flash chromatography (petroleum ether:EtOAc 8:2 to 6:4) as yellow oil. 1H NMR (CDCl3, 300 MHz): δ 2.56–2.62 (m, 2H, CHCH2CH=CH2), 3.30–3.35 (m, 4H, NCH2), 3.90–3.93 (m, 4H, OCH2), 5.10–5.25 (m, 3H, CHCH2CH=CH2 + OHCHCH2CH=CH2), 5.78–5.92 (m, 1H, CHCH2CH=CH2), 7.20 (d, 3J = 8.7 Hz, 1H, CH=CHCN), 7.38–7.43 (m, 3H, Ph), 7.48–7.51 (m, 2H, Ph), 7.67 (d, 3J = 8.7 Hz, 1H, CH=CHCCHOH); 13C NMR (CDCl3, 75 MHz): δ 42.8 (CHCH2CH=CH2), 51.6 (2C, NCH2), 66.9 (OCH2), 70.6 (CHCH2CH=CH2), 118.3 (CHCH2CH=CH2), 123.4 (CH=CHCN), 126.9 (CH, Ph), 128.3 (CH, Ph), 129.1 (CH, Ph), 131.4 (C), 134.4 (CH, CHCH2CH=CH2), 134.6 (CH, CH=CHCCHOH), 137.2 (C), 149.7 (C), 166.37 (C, NCO), 168.51 (C, NCO).
3-Hydroxy-6-morpholino-1,2-benzenedicarboxylic acid dimethyl ester (10)
Following general procedure for cycloaddition, 2 and DMAD led to 35 mg (45%) of the desired product 10 after flash chromatography (cyclohexane:EtOAc 8:2) as a colorless solid. 1H NMR (CDCl3, 300 MHz): δ 2.83–2.86 (m, 4H, NCH2), 3.71–3.75 (m, 4H, OCH2), 3.89 (s, 3H, CO2CH3CN), 3.91 (s, 3H, CO2CH3CCOH), 7.04 (d, 3J = 9.1 Hz, 1H, CH=CHCN), 7.43 (d, 3J = 8.9 Hz, 1H, CH=CHCCOH), 10.9 (s, 1H, OH); 13C NMR (CDCl3, 75 MHz): δ 52.2 (CO2CH3CN), 52.9 (CO2CH3CCOH), 53.5 (2C, NCH2), 67.6 (2C, OCH2), 108.9 (C), 119.7 (CH, CH=CHCN), 130.3 (CH, CH=CHCCOH), 134.6 (C), 142 (C), 159.4 (C), 168.5 (C, CO2CCN), 169.1 (C, CO2CCOH); CI-MS NH3 m/z (rel int): 296 (100, MH+); HRMS m/z calcd for C14H17NO6 295.1056, found 295.1055 ± 0.0001; Anal. Calcd for 3(C14H17NO6).H2O: C 55.81, H 5.91, N 4.65. Found: C 55.92, H 5.82, N 4.52.
3-Hydroxy-6-(N-4-methoxyphenyl-N-methylamino)-1,2-benzenedicarboxylic acid dimethyl ester (11)
Following general procedure for cycloaddition, 3c and DMAD led to 50.9 mg (45%) of the desired product 11 after flash chromatography (petroleum ether:EtOAc 8:2) as a colorless solid.1H NMR (CDCl3, 300 MHz): δ 3.07 (s, 3H, NCH3), 3.74 (s, 6H, CO2CH3), 3.93 (s, OCH3), 6.59 (d, 3J = 9.0 Hz, 2H, PhOMe), 6.76 (d, 3J = 9.1 Hz, 2H, PhOMe), 7.06 (d, 3J = 9.1 Hz, 1H, CH=CHCN), 7.24 (d, 3J = 9.0 Hz, 1H, CH=CHCCOH), 11.05 (s, 1H, OH); 13C NMR (CDCl3, 75 MHz): δ 40.8 (NCH3), 52.3 (CO2CH3CN), 53 (CO2CH3CCOH), 55.7 (OCH3), 109.3 (C), 114.3 (CH, NPhOMe), 116.1 (CH, NPhOMe), 120.9 (CH, CH=CHCN), 136.4 (CH, CH=CHCOH), 139.5 (C), 144.2 (C), 152.7 (C), 160.3 (C), 168.1 (C, CO2), 169.1 (C, CO2); Anal. Calcd for C18H19NO6: C 62.60, H 5.55, N 4.06. Found: C 62.09, H 5.61, N 4.01.
1,3-Dihydro-5-(4-morpholinyl)-3-oxo-4-isobenzofurancarboxylic acid ethyl ester (12)
Following general procedure for cycloaddition, 2 and diethyl maleate led to 20 mg (25%) of the desired product 12 after flash chromatography (cyclohexane:EtOAc 9:1 to 7.5:2.5) as a colorless oil. 1H NMR (CDCl3, 300 MHz): δ 1.34–1.40 (m, 3H, CO2CH2CH3), 3.04–3.07 (m, 4H, NCH2), 3.76–3.81 (m, 4H, OCH2), 4.45–4.52 (q, 3J = 7.5 Hz, 2H, CO2CH2CH3), 5.25 (s, 2H, CH2CO2CH2CH3), 7.30 (d, 3J = 8.3 Hz, 1H, CH=CHCN), 7.48 (d, 3J = 8.5 Hz, 1H, CH=CHCCH2O); 13C NMR (CDCl3, 75 MHz): δ 14.1 (CO2CH2CH3), 53.1 (2C, NCH2), 61.4 (OCH2), 62.1 (CO2CH2CH3), 67.2 (2C, OCH2), 123.7 (CH, CH=CHCN), 124.4 (C), 126.7 (CH=CHCCMe), 130.3 (C), 141.4 (C), 150.7 (C), 167.6 (C, CO), 168.0 (C, CO2Et); CI-MS NH3 m/z (rel int): 292 (100, M+H+).
1,3-Dihydro-1-methyl-5-(4-morpholinyl)-3-oxo-4-isobenzofurancarboxylic acid ethyl ester (13)
Following general procedure for cycloaddition, 3a and diethyl maleate led to 8 mg (10%) of the desired product 13 after flash chromatography (cyclohexane:EtOAc 7:3 to 1:1) as a colorless oil. 1H NMR (CDCl3, 300 MHz): δ 1.39–1.44 (t, 3J = 7.1 Hz, 3H, CO2CH2CH3), 1.62 (d, 3J = 6.6 Hz, 3H, CHCH3), 3.04–3.07 (m, 4H, NCH2), 3.78–3.82 (m, 4H, OCH2), 4.44–4.56 (q, 3J = 7.1 Hz, 2H, CO2CH2CH3), 5.46–5.54 (q, 1H, CHCH3), 7.40–7.46 (m, 2H, CH=CHCN + CH=CHCCMe); 13C NMR (CDCl3, 75 MHz): δ 14.2 (CO2CH2CH3), 20.3 (CHCH3), 53.2 (2C, NCH2), 62.1 (CO2CH2CH3), 67.6 (2C, OCH2), 77.2 (CH, CHCH3), 123.2 (CH, CH=CHCN), 124.3 (C), 126.7 (CH=CHCCMe), 128.8 (C), 146.2 (C), 150.7 (C), 166.3 (C, CO), 168.0 (C, CO2Et); CI-MS NH3 m/z (rel int): 306 (100, M+H+); HRMS m/z calcd for C16H19NO5 305.1263, found 305.1254 ± 0.0001; Anal. Calcd for C16H19NO5: C 62.94, H 6.27, N 4.59. Found: C 62.61, H 6.46, N 4.17.
1,3-Dihydro-5-(4-morpholinyl)-3-oxo-1-phenyl-4-isobenzofurancarboxylic acid ethyl ester (14)
Following general procedure for cycloaddition, 4 and diethyl maleate led to 14 mg (15%) of the desired product 14 after flash chromatography (cyclohexane:EtOAc 7:3 to 1:1) as a colorless oil. 1H NMR (CDCl3, 300 MHz): δ 1.42–146 (t, 3J = 7.1 Hz, 3H, CO2CH2CH3), 3.05–3.08 (m, 4H, NCH2), 3.79–3.82 (m, 4H, OCH2), 4.48–4.56 (q, 3J = 7.1 Hz, 2H, CO2CH2CH3), 6.34 (s, 1H, CHPh), 7.27–7.32 (m, 5H, Ph), 7.37–7.41 (m, 2H, CH=CHCN + CH=CHCCPh); 13C NMR (CDCl3, 75 MHz): δ 14.2 (CO2CH2CH3), 53.1 (2C, NCH2), 62.2 (CO2CH2CH3), 67.2 (2C, OCH2), 82.2 (CHPh), 123.7 (C), 124.5 (CH, Ph), 126.9 (CH, Ph), 127 (CH, CH=CHCN), 128.4 (C), 129.0 (CH=CHCCPh), 129.1 (CH, Ph), 136.1 (C), 144.5 (C), 150.9 (C), 166.2 (C, CO), 168.1 (C, CO2Et); HR-MS m/z calcd for C21H21NO5 367.1420, found 367.1421 ± 0.0001, Anal. Calcd for C21H21NO5.H2O: C 65.44, H 6.02, N 3.63. Found: C 65.38, H 5.91, N 3.51.
ACKNOWLEDGMENTS
Authors are highly grateful to the Université de Versailles-Saint-Quentin-en Yvelines for material support and to CNRS for financial support, especially for a grant (R. M.) and ANR program (ANR–07–CP2D–14–01).
References
1. O. C. Kappe, S. S. Murphree, and A. Padwa, Tetrahedron, 1997, 53, 14179. CrossRef
2. For recent elegant examples see: Y. W. Goh, B. R. Pool, and J. M. White, J. Org. Chem., 2008, 73, 151; CrossRef R. N. Ram and N. Kumar, Tetrahedron Lett., 2008, 49, 799. CrossRef
3. A. Padwa, K. R. Crawford, P. Rashatasakhon, and M. Rose, J. Org. Chem., 2003, 68, 2609; CrossRef A. Padwa, M. A. Brodney, K. Satake, and C. S. Straub, J. Org. Chem., 1999, 64, 4617; CrossRef A. Padwa, M. Dimitroff, and A. G. Waterson, J. Org. Chem., 1997, 62, 4088. CrossRef
4. J. E. Cochran and O. Sterner, Tetrahedron Lett., 1996, 37, 2903; CrossRef N. Chatani and T. Hanafusa, J. Org. Chem., 1987, 52, 4408; CrossRef M. F. Semmelhack and J. Park, Organometallics, 1986, 5, 2550; CrossRef G. V. Boyd and K. Heatherington, J. Chem. Soc., Perkin Trans. 1, 1973, 2523. CrossRef
5. E. Migianu, D. Prim, and G. Kirsch, Synlett, 2000, 459; D. Prim and G. Kirsch, Tetrahedron, 1999, 55, 6511; CrossRef D. Prim, G. Kirsch, and J.-F. Nicoud, Synlett, 1998, 383. CrossRef
6. R. Medimagh, S. Marque, D. Prim, S. Chatti, and H. Zarrouk, J. Org. Chem., 2008, 73, 2191. CrossRef
7. M. E. Farkas, E. Rodriguez, C. Longo, M. Monasterios, M. C. Ortega, A. B. Rivas, A. J. Pardey, R. Lopez, and S.A. Moya, J. Chil. Chem. Soc., 2006, 51, 829. Sole 5-amino-2-furylmethanol is mentioned on the text, however, nor isolation neither spectroscopic data were available.
8. For recent example of rearrangments with loss of methylene group see: S. D. Carter, D. Thetford, P. G. Sammes, and M. Voyk, J. Chem. Soc., Perkin Trans. 1, 1990, 1231. CrossRef