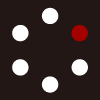
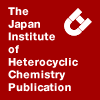
HETEROCYCLES
An International Journal for Reviews and Communications in Heterocyclic ChemistryWeb Edition ISSN: 1881-0942
Published online by The Japan Institute of Heterocyclic Chemistry
e-Journal
Full Text HTML
Received, 22nd September, 2008, Accepted, 5th November, 2008, Published online, 6th November, 2008.
DOI: 10.3987/COM-08-S(D)27
■ Preparation and Electrochemical and Optical Properties of Tetrakis(dithiaselenolo)phthalocyanine
Takeshi Kimura,* Toshiharu Namauo, Akio Yamakawa, and Yutaka Takaguchi
Center for Instrumental Analysis, Iwate University, 4-3-5 Ueda, Morioka, Iwate 020-8551, Japan
Abstract
Four o-xylylene groups of phthalocyanine (2) were removed by Birch reduction and the generated octathiolate anions were then reacted with Se to give tetrakis(dithiaselenolo)phthalocyanine (3). The dication generated from 3 in CHCl3/TFA was determined with UV-vis and NMR spectroscopy.Phthalocyanines have attracted much attention for their actual and/or potential applications in a variety of fields.1,2 New types of phthalocyanines have been sought after for developing functional materials, which has stimulated study of the compounds and related areas. One method for the derivation of phthalocyanines is to introduce heteroatoms at the peripheral positions as substituents or in its fused ring systems.3-5 We recently reported the preparation of tetrakis(trithiolo)phthalocyanine (PcTTT) by treatment of tetrakis(o-xylylenedithio)phthalocyanine (2) with Li/THF/NH3 and then with S8.5 PcTTT could be protonated with TFA to generate a charged species, which was determined by UV-vis and NMR spectroscopy. If Se atoms could be substituted on the five-membered rings of PcTTT, it would be possible to obtain information regarding the electronic state of the dithiaselenole rings by using the NMR technique. This paper reports the preparation and structure determination of tetrakis(dithiaselenolo)-phthalocyanine (3). In addition, the cationic species, generated by treatment of 3 with TFA in CHCl3, was examined with UV-vis and NMR spectroscopy.
Phthalocyanine (2) was prepared from phthalonitrile (1) by the method previously reported (Scheme 1).5a The S-C bond of the xylylenedithio groups of 2 could be cleaved by Birch reduction,4-6 and the generated thiolate anions were functionalized by treatment with Se.
Compound (2) was treated with Li in THF and NH3 at –70 °C under Ar for 1 h. After evaporation of NH3 under a nitrogen gas stream, deaerated MeOH was added to the reddish-brown solid. NH4Cl and Se were added and the solution was stirred for 5 h at rt. The reaction mixture was then treated with NaBH4 and K2CO3 and the solution was stirred for 15 h. After workup and purification, phthalocyanine (3) was obtained in 27% yield as a dark-brown powder. The product was stable in air and the solubility in CHCl3 was high. The Q band absorption of 3 in the UV-vis spectrum was observed at λmax = 777 nm, log ε = 5.26 (Figure 1a), which is a lower energy region than that of PcTTT (λmax = 769 nm). In the 1H NMR spectrum, it was possible to confirm the disappearance of the signal of the xylylene groups. 77Se NMR showed a signal at δ = 709.4 ppm, which is a chemical shift similar to that of benzodithiaselenole.7 Compound (3) was finally measured by MALDI-TOF-MS and the molecular ion peak was observed at 1979.264 [MH+]. These results suggest that the dithiaselenole rings are constructed on the phthalocyanine skeleton.
To generate a charged species, 3 was treated with CHCl3 containing 5 vol% of TFA, and the UV-vis spectrum was measured in the solvent (Figure 1a).8,9 In the spectrum, the Q-band absorption of 3 was observed at λmax = 925 nm, suggesting that the cationic species of 3 was generated by protonation of the nitrogen atoms and the positive charge strongly affects the π-electronic system of phthalocyanine. The Q-band absorption of 3 is longer in wavelength in the solvent than is that of PcTTT by about 10 nm.
1H, 13C, and 77Se NMR spectra of 3 were then measured using CDCl3/TFA-d (5 vol%) as the solvent.9 When the 1H NMR spectrum of 3 was measured in the solvent, the signals of the methylene groups connected to phthalocyanine and of the next methylene groups were observed as two split signals (δ = 1.58–1.71 and 1.71–1.86, and 3.43–3.56 and 4.19-4.32), and appeared at a higher magnetic field than was the spectrum measured in CDCl3 (Figure 2-i and ii). The other signals of the octyl group were observed at a lower field. The splitting of the methylene signal could be caused by the hindered rotation of the octyl group due to steric congestion with the dithiaselenole rings or by the conformation change of phthalocyanine from a planar form to a non-planar saddle conformation.10
The 13C NMR spectrum showed the four signals for the aromatic carbon at δ = 126.0, 138.0, 143.9, and 152.2, demonstrating that 3 had a completely symmetric structure and the macrocycle is deuterated with TFA-d on the inner pyrrolic nitrogen atoms.5b It was reported that one or two signals for the pyrrole carbons of free base phthalocyanine could not be observed in the 13C NMR spectrum at around rt due to the effect of proton alternation between the four central nitrogen atoms.11 Therefore, the species generated in the solution should be the dication of 3 with deuteration on the two pyrrolic nitrogen atoms. The signal of the 77Se atoms was observed at δ = 707.2 ppm and the chemical shift is similar to that of 3 measured in CDCl3. This result suggests that the dithiaselenole rings may not be strongly affected by the protonation of phthalocyanine.
In contrast, in the 1H NMR spectrum, the signal of the methylene groups slowly became broad and the two split signals changed to two broad signals after 15 h (Figure 2-iii). The signal of 77Se atoms, observed initially, disappeared in the NMR spectrum, which could have been caused by the broadening of this signal. In the case of PcTTT, no change of the 1H NMR spectrum was observed. To identify the compound in the solution, the solvent was evaporated and 1H NMR of the residue was measured in CDCl3. In the spectrum, the signal of 3 could be observed together with one decomposed product in a 7:1 integral ratio. After purification, the structure of the new product could be identified as 2,5-dioctyl-3,4-dithiaselenolophthalimide (4). It seems that 3 is gradually hydrolyzed in CDCl3/TFA-d. Since 4 was not the major product, it is thought that the changes of the 1H and 77Se NMR spectra occurred by the partial deuteration of the four outer nitrogen atoms of phthalocyanine and the rate of the deuterium exchange was similar to the NMR time scale.
The electrochemical property of 3 was determined by cyclic voltammetry using Ag/AgNO3 as a reference electrode (Figure 1b). Compound (3) shows three quasi-reversible oxidation couples (E1/2 = 0.54, 0.77, and 1.10 V) and one irreversible reduction potential (Ep = –1.14 V). In contrast, PcTTT showed two reversible oxidation potentials (E1/2 = 0.56 and 0.82 V) and one quasi-reversible reduction potential (E1/2 = –0.96 V).5a It is expected that the first and second oxidation potentials originate from the oxidation of phthalocyanine and the last one is caused by the oxidation of the four dithiaselenole rings. It appeared that the first and second oxidation potentials of 3 are slightly lower than are those of PcTTT, suggesting that the difference of oxidation potential between 3 and PcTTT might determine the protonation of the outer nitrogen atoms of 3 in CDCl3/TFA-d.
EXPERIMENTAL
General
NMR spectra were measured with a Bruker AC-400 spectrometer. Mass spectra were obtained using a JEOL JMS-700 mass spectrometer and a PerSeptive Biosystems Voyager RP-DE mass spectrometer. UV-vis spectra were recorded with a JASCO Ubest V-570 spectrometer. A Hokuto Denko Co. Model HAB-151 apparatus was applied to measurements of redox potentials. Bio-beads (SX-1) for column chromatography was purchased from Nippon Bio-Rad Laboratories.
Oxidation potential
All measurements were performed by cyclic voltammetry, using Ag/AgNO3 (0.01 mol/l) as a reference electrode (scan rate: 200 mV/s). A solution of n-Bu4NClO4 in CH2Cl2 (0.1 mol/l) was used as an electrolyte. The oxidation potential of ferrocene was observed at E1/2 = 0.09 V by the apparatus without any correction.
Tetrakis(o-xylylenedithio)phthalocyanine (2).
Compound (2) was prepared using a method described previously.5a
Deprotection of 2 and Cyclization of Lithium Octathiolate with Se
Compound (2) (110.4 mg, 0.53 mmol) and Li (38 mg, 5.5 mmol) were placed in a glass reactor. THF (4 mL) was added under Ar, and the solution was cooled to –78 °C. NH3 (30 mL) was introduced into the reactor and was condensed. The solution was stirred for 1 h at this temperature, and gradually warmed to rt. NH3 gas was evaporated under the N2 gas stream. After evaporation of NH3 was over, deaerated MeOH was added to the reddish-brown solid and NH4Cl and Se were added to the solution and the solution was stirred for 5 h at rt. The reaction mixture was then treated with NaBH4 and K2CO3 and the solution was stirred for 15 h. After workup and purification with column chromatography (Wakogel C-300HG, n-hexane and Bio-beads SX-1, CHCl3), phthalocyanine (3) was obtained in 27% yield (28.2 mg); 3: dark-brown powder; mp 300 °C >; 1H NMR (400 MHz, CDCl3) δ = 0.76 (t, J = 6.7 Hz, 24H), 1.03–1.31 (m, 64H), 1.51 (quint, J = 7.5 Hz, 16H), 1.83 (quint, J = 7.5 Hz, 16H), 4.49 (br, 16H); 77Se NMR (76 MHz, CDCl3) δ = 709.4; MALDI-TOF-MS (m/z) 1979.264 (MH+). The protons on the nitrogen atoms of the pyrrole rings could not be observed in the spectra under the measurement condition.
NMR Spectra Measured in CDCl3/TFA-d
1H, 13C, and 77Se NMR spectra of 3 were measured in CDCl3/TFA-d; 1H NMR (400 MHz) δ = 0.87 (t, J = 6.4 Hz, 24H), 1.15–1.40 (m, 64H), 1.47–1.58 (m, 16H), 1.58¬–1.71 (m, 8H), 1.71–1.86 (m, 8H), 3.43–3.56 (m, 8H), 4.19-4.32 (m, 8H); 13C NMR (101 MHz) δ = 14.0, 22.7, 29.2(9), 29.3(4), 29.5, 30.0, 31.9, 34.0, 126.0, 138.0, 143.9, 152.2; 77Se NMR (76 MHz) δ = 707.2. After the NMR measurement in CDCl3/TFA-d, the solvent was evaporated and the residue was purified by column chromatography to produce 3 and 4; 4: 1H NMR (400 MHz, CDCl3) δ = 0.88 (t, J = 6.9 Hz, 6H), 1.20–1.65 (m, 24H), 3.15 (t, J = 7.9 Hz, 4H), 7.41 (br, 1H); HRFAB Calcd for C24H35NO2S2Se, 513.1275. Found, (m/z) 513.1290 (M+).
References
1. a) ‘The Porphyrin Handbook’, Vols. 15-20, ed. by K. M. Kadish, K. M. Smith, and R. Guilard, Academic Press, San Diego, 2003; b) G. de la Torre, C. G. Claessens, and T. Torres, Chem. Commun., 2007, 2000; c) ‘Phthalocyanines–Chemistry and Functions’, ed. by H. Shirai and N. Kobayashi, IPC, Tokyo, 1997 (in Japanese).
2. a) ‘Phthalocyanines: Properties and Applications’, Vols. 1-4, ed. by C. C. Leznoff and A. B. P. Lever, VCH, New York, 1989-1996; b) D. Wöhrle, O. Suvorova, R. Gerdes, O. Bartels, L. Lapok, N. Baziakina, S. Makarov, and A. Slodek, J. Porphyrins Phthalocyanines, 2004, 8, 1020; CrossRef c) G. de la Torre, P. Vázquez, F. Agulló-López, and T. Torres, Chem. Rev., 2004, 104, 3723. CrossRef
3. a) P. A. Stuzhin, E. M. Bauer, and C. Ercolani, Inorg. Chem., 1998, 38, 1533; CrossRef b) E. M. Bauer, C. Ercolani, P. Galli, I. A. Popkova, and P. A. Stuzhin, J. Porphyrins Phthalocyanines, 1999, 3, 371; CrossRef c) S. M. Baum, A. A. Trabanco, A. G. Montalban, A. S. Micallef, C. Zhong, H. G. Meunier, K. Suhling, A. J. P. Phillips, A. J. P. White, D. J. Williams, A. G. M. Barrett, and B. M. Hoffman, J. Org. Chem., 2003, 68, 1665. CrossRef
4. T. Kimura, A. Yomogita, T. Matsutani, T. Suzuki, I. Tanaka, Y. Kawai, Y. Takaguchi, T. Wakahara, and T. Akasaka, J. Org. Chem., 2004, 69, 4716. CrossRef
5. a) T. Kimura, T. Suzuki, Y. Takaguchi, A. Yomogita, T. Wakahara, and T. Akasaka, Eur. J. Org. Chem., 2006, 1262; CrossRef b) T. Kimura, N. Kanota, K. Matsui, I. Tanaka, T. Tsuboi, Y. Takaguchi, A. Yomogita, T. Wakahara, S. Kuwahara, F. Nagatsugi, and T. Akasaka, Inorg. Chem., 2008, 47, 3577. CrossRef
6. a) C. S. Velázquez, W. E. Broderick, M. Sabat, A. G. M. Barrett, and B. M. Hoffman, J. Am. Chem. Soc., 1990, 112, 7408; CrossRef b) C. S. Velázquez, G. A. Fox, W. E. Broderick, K. A. Anderson, O. P. Anderson, A. G. M. Barrett, and B. M. Hoffman, J. Am. Chem. Soc., 1992, 114, 7416. CrossRef
7. S. Ogawa, T. Ohmiya, T. Kikuchi, A. Kawaguchi, S. Saito, A. Sai, N. Ohyama, Y. Kawai, S. Niizuma, S. Nakajo, T. Kimura, and R. Sato, J. Organomet. Chem., 2000, 611, 136. CrossRef
8. a) P. A. Bernstein and A. B. P. Lever, Inorg. Chem. Acta, 1992, 198-200, 543; CrossRef b) P. A. Stuzhin, J. Porphyrins Phthalocyanines, 1999, 3, 500. CrossRef
9. The effect of the positive charge on the Q-band of tetrazaporphyrine derivative was reported; N. Kobayashi, T. Nonomura, and K. Nakai, Angew. Chem. Int. Ed., 2001, 40, 1300. CrossRef
10. C. Liu, D.-M. Shen, and Q.-Y. Chen, J. Am. Chem. Soc., 2007, 129, 5814. CrossRef
11. It has been reported that the tautomerization of inner protons affects the signals of 1H NMR and 13C NMR spectra; a) R. J. Abraham, G. E. Hawkes, and K. M. Smith, Tetrahedron Lett., 1974, 1483; CrossRef b) R. J. Abraham, G. E. Hawkes, M. F. Hudson, and K. M. Smith, J. Chem. Soc., Perkin Trans. 2, 1975, 204; CrossRef M. J. Cook, S. J. Cracknell, G. R. Moore, M. J. Osborne, and D. J. Williamson, Magn. Reson. Chem., 1991, 29, 1053. CrossRef