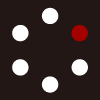
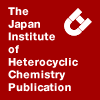
HETEROCYCLES
An International Journal for Reviews and Communications in Heterocyclic ChemistryWeb Edition ISSN: 1881-0942
Published online by The Japan Institute of Heterocyclic Chemistry
e-Journal
Full Text HTML
Received, 24th September, 2008, Accepted, 23rd October, 2008, Published online, 27th October, 2008.
DOI: 10.3987/COM-08-S(F)118
■ Anomeric Samarium(III) Intermediates of N-Acetylneuraminic Acid from Anomeric 2-Pyridylsulfides
Adeline Malapelle, Zouleika Abdallah, Gilles Doisneau, and Jean-Marie Beau*
Laboratoire de Synthese de Biomolecules, Institut de Chimie Moleculaire et Materiaux associe au CNRS, Université de Paris-Sud 11, Batiment 430, F-91405 Orsay Cedex, France
Abstract
Treatment of the anomeric 2-thiopyridyl derivative of N-acetylneuraminic acid (sialic acid or Neu5Ac) with samarium diiodide in the presence of aldehydes or ketones provides the corresponding C-sialylated derivatives in excellent yields. The efficiency of the reductive samariation of the anomeric 2-pyridylsulfide moiety in the Neu5Ac series does not require any cosolvent or additive. This samarium Reformatsky-like reaction from anomeric 2-pyridylsulfide derivatives of Neu5Ac provides an efficient and highly stereoselective access to α-2,6-C-disaccharides.N-Acetylneuraminic acid (Neu5Ac) is the more common nine-carbon member of the ulosonic acids family, that are important constituents of numerous oligosaccharides and glycoconjugates.1 The terminal position of the Neu5Ac residues in these conjugates confer a major role to these structures in many biological processes.2,3 The α-glycosidic linkage of this important structural unit exhibits however poor chemical and enzymatic hydrolytic stability thus providing unstable tools for biological research. The replacement of the interglycosidic oxygen by a carbon atom provides carbon-linked analogues (C-glycosides) that are stable to hydrolysis.4 Based on our previous work on neutral aldoses5 and 2-acetamido-2-deoxy-sugars,6 Linhardt and coworkers7 have reported, over the past years, efficient and stereoselective access to C-glycosides of Neu5Ac based on the reductive metallation of anomeric aryl sulfones and chloride. In an effort to further simplify the preparation of the anomeric organometallic precursor, our laboratory previously reported procedures that rely on the reductive samariation of standard peracetylated intermediates8 and subsequent coupling with carbonyl electrophiles. Along these lines, and during the course of a synthesis of a carbon-linked mimic of the disaccharidic unit of the tumor-related sialyl-Tn antigen, we also showed that the use of 2-pyridyl sulfide of N-acetyl neuraminic acid derivative 3 as the anionic precursor in a samarium-Reformatsky procedure was remarkably efficient.9 This prompted us to study this coupling reaction with diverse substrates and we report here the details and the scope of the reaction.
The crystalline sulfide 3 was prepared from commercial Neu5Ac 1 according to the reactions described in Scheme 1. Commercial Neu5Ac 1 was quantitatively esterified in a methanolic acid solution and the methyl ester thus obtained was subsequently treated by a 1/1 mixture of acetic acid and acetyl chloride saturated at 0°C with hydrogen chloride to furnish the chloride 2.10 With 2-thiopyridine as nucleophile (3.7 equivalents) in biphasic reaction conditions, the anomeric chloride 2 gave 3 in 60% yield. The same reaction using thiophenol as a nucleophile gave the corresponding anomeric thiophenyl derivative 4 in 68% yield.
We started our study on the reductive samariation of 3 in the presence of reactive cyclic ketones (2 equivalents). The coupling reaction with cyclobutanone gave the C-sialyl derivative 5 in 96% yield. As expected and in agreement with previous observations on the reductive coupling from other anomeric derivatives,7,8 only one diastereomer with an equatorial orientation of the new C-C bond could be detected by 1H-NMR analysis of the crude product. Reactions with cyclopentanone and cyclohexanone gave with equal efficiency the corresponding coupling products 6 and 7 in 94% and 82% yields, respectively (Scheme 2).
We were pleased to observe that the Sm(II) reduction of the anomeric 2-thiopyridyl group of the substrate was instantaneous at room temperature as indicated by the rapid discoloration of the dark blue THF-SmI2 solution. The first electron transfer from Sm(II) to the 2-thiopyridyl group was fast enough to avoid the addition of cosolvants like HMPA,11 DMPU,12 or other chelating agents.13,14
The reaction leading to α-C-sialosides was then examined with several simple electrophiles including acyclic ketones or aliphatic aldehydes. The coupling reactions from 3 always proceeded in high yields with total selectivity in favor of a new equatorial C-C bond. Table 1 lists the results of these reactions. The products obtained from the coupling with an unsymmetrical ketone (entry 1, Table 1) or from aldehydes (entries 3-5, Table 1) were isolated as a 1/1 mixture of two diastereoisomers at the exocyclic asymmetric center. The selective formation of monomeric or dimeric structures could be achieved using 1.4 or 0.45 equivalent of 1,4-cyclohexandione (entries 7-8, Table 1), providing adducts 14 and 15, respectively. Reactions were strictly conducted under Barbier conditions, due to the instability of the intermediate organosamarium species, and under an argon atmosphere as samarium diiodide is highly oxygen sensitive.
The high efficiency and selectivity of this reaction was exemplified by the quantitative formation of the C-sialoside 13 issued from the coupling of 3 with 4-tertbutylcyclohexanone. We assume that this Reformatsky-like reaction goes through the formation of a samarium enolate which reacts with electrophiles from its less hindered α-face, leading to the formation of an equatorial carbon-carbon bond. The two diastereotopic faces of the 4-tert-butylcyclohexanone are also highly discriminated by the organosamarium intermediate which reacts at its more accessible one leading to a single diastereomer (Scheme 3).
A possible explanation for the formation of a samarium(III) enolate is given in Scheme 4, although no detailed mechanistic study was undertaken. The reaction proceeds through two successive electron transfers. With the 2-pyridyl sulfide group, activation of the carbon-nitrogen double bond through a pre-complexation of the samarium(II) reagent as in complex I (Scheme 4) would precede the first electron transfer into the pyridyl nucleus. Carbon-sulfur bond cleavage that follows is fast because of the formation of an anomeric radical III further stabilized by the adjacent methyl ester group. The geometry of this enolate could be the one shown in V with a coordination of the oxophilic Sm(III) to the adjacent endocyclic oxygen, leading to the cyclic structure.
Under the same reaction conditions, the anomeric phenyl sulfide 4 does not react with samarium diiodide, and only 25% of the coupling product can be isolated from the reaction with cyclopentanone in the presence of 4 equivalents of HMPA (results not shown). This demonstrates the crucial role of the 2-pyridyl moiety in 3 that may act as an external ligand like HMPA, inducing a faster internal electron transfer and formation of the anomeric radical (Scheme 4). As we suggested earlier,4a the initial homolytic cleavage of the C-S bond is faster as it leads to a stabilized captodative radical.15 With this very reactive sulfide derivative, the use of only 2.2 equivalents of samarium diiodide is sufficient enough to achieve the complete conversion of the starting material.
We then focused on the reaction with more elaborated electrophiles derived from monosaccharides in order to prepare 2,6-C-disaccharidic derivatives of Neu5Ac. The coupling reactions of 3 with the sugar aldehyde derivatives 16,16 17,5f and 18 9 gave the corresponding C-dimers 19-21 in high yields, each isolated as an equimolar mixture of diastereomers (Table 2).
One should take notice of the remarkable efficiency of the reaction even for the coupling with the aldehyde derived from a GalNAc unit 18 in which the electrophile also possesses an acidic proton (NHAc), considering that these coupling reactions process through sensitive organometallic species.8a
In conclusion, the reductive samariation of the acetylated pyridyl sulfide of methyl N-acetyl-neuraminate in the presence of a variety of carbonyl compounds provides a useful and high yielding approach for stereoselective α-C-sialylation. The reactions are very efficient even for the challenging preparation of C-disaccharides. This procedure complements the use of the corresponding peracetylated derivatives that react in excellent yields only with simple and more reactive cyclic carbonyl compounds8 but that are not efficient enough for the coupling with the more demanding sugar-derived aldehydes.
EXPERIMENTAL
For the general conditions see reference 8b.
Preparation of a 0.1M THF solution of samarium diiodide
Samarium powder (0.190 g, 1.26 mmol) was placed in a dry flask under argon. After stirring for 15 min., 1,2-diiodoethane (0.280 g, 1.00 mmol) was added and the mixture was stirred for 10 min. Freshly distilled THF (10 mL) was slowly added and the content of the flask was magnetically stirred for at least 5 h at room temperature to give a deep blue solution.
Two representative procedures for the SmI2 coupling reactions:
C-Dimer 15
A 0.1M solution of SmI2 in THF (4.5 mL, 0.45 mmol of SmI2) was added to a stirred THF (1 mL) solution of pyridyl sulfide 3 (114 mg, 0.19 mmol) and 1,4-cyclohexanedione (9.6 mg, 0.085 mmol, 0.45 eq) at 20 °C under Ar. After stirring the mixture for 10 min, saturated aqueous NH4Cl was added and the reaction mixture was extracted 3 times with CH2Cl2. The combined organic phases were washed twice with water, dried with Na2SO4, evaporated and co-evaporated with toluene. The crude product was purified by flash chromatography on silica gel (toluene/acetone, 1/1) to yield 15 (88 mg, 0.083 mmol, 98%). Mp 243 °C (toluene/acetone); 1H NMR (CDCl3, 360 MHz): δ (ppm) = 5.42 (ddd, 2 H, J8,7 = 8.2 Hz, J8,9b = 6.6 Hz, J8,9a = 2.6 Hz, H-8), 5.29 (dd, 2 H, J7,8 = 8.0 Hz, J7,6 = 1.8 Hz, H-7), 5.13 (d, 2 H, JNH,5 = 9.6 Hz, NH), 4.72 (ddd, 2 H, J4,3ax = 11.9 Hz, J4,5 = 9.7 Hz, J4,3eq = 4.6 Hz, H-4), 4.32 (dd, 2 H, J9a,9b = 12.4 Hz, J9a,8 = 2.6 Hz, H-9a), 4.05-3.95 (m, 6 H, H-6, H-5, H-9b), 3.75 (s, 6H, CO2CH3), 2.77 (br s, 2 H, OH), 2.45 (dd, 2 H, J3eq,3ax = 12.8 Hz, J3eq,4 = 4.6 Hz, H-3eq), 2.16, 2.13, 2.04, 2.00 (4s, 24H, 8 OAc), 1.92 (t, 2 H, , J3ax,3eq = 12.8 Hz, J3ax,4 = 11.9 Hz, H-3ax), 1.85 (s, 6H, NAc), 1.75-1.58 (m, 8 H, CH2 of cyclohexyl); 13C NMR (CDCl3, 90 MHz): δ (ppm) = 171.1, 170.8, 170.2, 170.1, 169.9, and 169.8 (8 OCOCH3, 2 NCOCH3, 2 COOCH3), 86.0 (C-2), 74.6 (COH), 73.2, 70.4, 68.6, 67.9 (C-4, C-6, C-7, C-8), 62.8 (C-9), 52.4 (CH3O), 49.4 (C-5), 33.1 (C-3), 27.9-26.6 (4 CH2 of cyclohexyl), 23.2, 21.4, 20.9, 20.8, and 20.7 (8CH3OCO, 2CH3CON); MS (ESI): m/z = 1085.5 [M+Na]+; HR-MS (ES) for C46H66O26N2Na; Calcd: 1085.3796; Found: 1085.3819. Anal. Calcd for C46H66O26N2: C, 51.97; H, 6.26; N, 2.64; Found: C 51.64; H 6.22; N 2.31.
C-Disaccharide 20
A 0.1M solution of SmI2 in THF (1.12 mL, 0.112 mmol of SmI2) was added to a stirred mixture of pyridyl sulfide 3 (30 mg, 0.051 mmol) and freshly prepared aldehyde 17 (49 mg, 0.103 mmol) at 20 °C under Ar. After treatment of this reaction mixture as described above, a flash chromatography (toluene/acetone, 3/1) gave 20 (38.8 mg, 80%) as a 1/1 mixture of isomers. Selected data for (R/S) mixture: 1H NMR (CDCl3, 360 MHz, atom numbering of the dimer): δ (ppm) = 7.40-7.25 (m, 30 H, Ph), 5.45 (ddd, 2 H, J8,7 = 7.4 Hz, J8,9b= 6.4 Hz, J8,9a = 2.2 Hz, H-8), 5.33-5.29 (m, 2 H, H-7), 4.94 (dd, 1 H, J = 10.8 Hz, J = 1.8 Hz, H-2’), 4.82-4.55 (d, 12 H, J = 11.2 Hz, CH2Ph), 4.31-3.57 (m, 22 H, H-3’, H-4’, H-5’, 2 H-6’, H-4, H-5, H-6, H-9a, H-9b, H-7’), 3.73 (s, 3H, COOMe), 3.65 (s, 3H, COOMe), 3.23 (s, 6 H, OMe), 2.34 (dd, 2 H, J3eq,3ax = 13.0 Hz, J3eq,4 = 4.8 Hz, H-3eq), 2.12, 2.08, 2.02, 2.01, 2.00, 1.97 (6s, 24 H, OAc), 1.86 (t, 2 H, J3ax,3eq = 13.0 Hz, J3ax,4 = 12.5 Hz, H-3ax), 1.85 (s, 3 H, NAc), 1.84 (s, 3 H, NAc). MS (ES): m/z = 974 [M+Na]+; HR-MS (ES) for C49H61O18NNa; Calcd: 974.3786; Found: 974.3798.
ACKNOWLEDGEMENTS
We are grateful to the Ministère de la Recherche for Ph.D grants to A. M. and Z. A. and for the financial support of this study.
This paper is dedicated to Professor Emeritus Keiichiro Fukumoto on the occasion of his 75th birthday.
References
1. R. Schauer, ‘Sialic Acids: Chemistry, Metabolism and Function’, Springer-Verlag: Vienna, 1982, Cell Biology Monograph series, Vol. 10; R. Schauer, Adv. Carbohydr. Chem. Biochem., 1982, 40, 131; CrossRef M. J. Kiefel and M. von Itzstein, Chem. Rev., 2002, 102, 471. CrossRef
2. A. Rosenberg, ‘Biology of Sialic Acids’, A. Plenum Press: New York, London, 1995.
3. R. Schauer, ‘Carbohydrates in Chemistry and Biology’ Vol. 3, ed. by B. Ernst, G. W. Hart, and P. Sinaÿ, Wiley-VCH: Weinheim, 2000, pp. 227-243; CrossRef T. Angataa and A. Varki, Chem. Rev., 2002, 102, 439. CrossRef
4. Reviews: a) J.-M. Beau and T. Gallagher, Topics Curr. Chem., 1997, 187, 1; CrossRef b) Y. Du, R. J. Linhardt, and I. R. Vlahov, Tetrahedron, 1998, 54, 9913; CrossRef c) A. Dondoni and A. Marra, Chem. Rev., 2000, 100, 4395; CrossRef d) L. Somsák, Chem. Rev., 2001, 101, 81. CrossRef
5. Neutral hexoses: a) D. Mazéas, T. Skrydstrup, O. Doumeix, and J.-M. Beau, Angew. Chem., Int. Ed. Engl., 1994, 33, 1383; CrossRef b) D. Mazéas, T. Skrydstrup, and J.-M. Beau, Angew. Chem., Int. Ed. Engl., 1995, 34, 909; CrossRef c) T. Skrydstrup, D. Mazéas, M. Elmouchir, G. Doisneau, C. Riche, A. Chiaroni, and J.-M. Beau, Chem. Eur. J., 1997, 3, 1342; CrossRef d) T. Skrydstrup, O. Jarreton, D. Mazéas, D. Urban, and J.-M. Beau, Chem. Eur. J., 1998, 4, 655; CrossRef e) O. Jarreton, T. Skrydstrup, J.-F. Espinosa, J. Jiménez-Barbero, and J.-M. Beau, Chem. Eur. J., 1999, 5, 430 ; CrossRef f) N. Miquel, G. Doisneau, and J.-M. Beau, Angew. Chem. Int. Ed., 2000, 39, 4111. CrossRef
6. a) D. Urban, T. Skrydstrup, C. Riche, A. Chiaroni, and J.-M. Beau, Chem. Commun., 1996, 1883; CrossRef b) D. Urban, T. Skrydstrup, and J.-M. Beau, J. Org. Chem., 1998, 63, 2507; CrossRef c) L. Andersen, L. M. Mikkelsen, J.-M. Beau, and T. Skrydstrup, Synlett, 1998, 1393; CrossRef d) S. Palmier, B. Vauzeilles, and J.-M. Beau, Org. Biomol. Chem., 2003, 1, 1097. CrossRef
7. a) I. R. Vlahov, P. I. Vlahova, and R. J. Linhardt, J. Am. Chem. Soc., 1997, 119, 1480; CrossRef b) T. Polat, Y. Du, and R. J. Linhardt, Synlett, 1998, 1195; CrossRef c) B. Kuberan, S. A. Sikkander, H. Tomiyama, and R. J. Linhardt, Angew. Chem. Int. Ed., 2003, 42, 207. CrossRef
8. a) A Malapelle, Z. Abdallah, G. Doisneau, and J.-M. Beau, Angew. Chem. Int. Ed., 2006, 45, 6016; CrossRef b) A Malapelle, A. Coslovi, G. Doisneau, and J.-M. Beau, Eur. J. Org. Chem., 2007, 3145. CrossRef
9. Z. Abdallah, G. Doisneau, and J.-M. Beau, Angew. Chem. Int. Ed., 2003, 42, 5209. CrossRef
10. A. Lubineau and J. Le Gallic, J. Carbohydr. Chem., 1991, 10, 263. CrossRef
11. J. Inanaga, M. Ishikawa, and M. Yamaguchi, Chem. Lett., 1987, 1485. CrossRef
12. E. Hasegawa and D. P. Curran, J. Org. Chem., 1993, 58, 5008. CrossRef
13. A. Dahlén and G. Hilmersson, Eur. J. Inorg. Chem., 2004, 3393. CrossRef
14. These additives are often delicate to use in our experiments because they also accelerate a concomitant electron transfer into the carbonyl group of the electrophilic partner.
15. For a related reductive samariation of glycyl sulfide derivatives see M. Ricci, L. Madariaga, and T. Skrydstrup, Angew. Chem. Int. Ed., 2000, 39, 242; CrossRef M. Ricci, P. Blakskjaer, and T. Skrydstrup, J. Am. Chem. Soc., 2000, 122, 12413. CrossRef
16. M. Koketsu, B. Kuberan, and R. J. Linhardt, Org. Let., 2000, 2, 3361; CrossRef J. M. Benito, C. O. Mellet, and J. M. G. Fernández, Carbohydr. Res., 2000, 323, 218. CrossRef