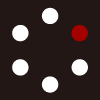
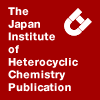
HETEROCYCLES
An International Journal for Reviews and Communications in Heterocyclic ChemistryWeb Edition ISSN: 1881-0942
Published online by The Japan Institute of Heterocyclic Chemistry
e-Journal
Full Text HTML
Received, 25th September, 2008, Accepted, 23rd October, 2008, Published online, 31st October, 2008.
DOI: 10.3987/COM-08-S(D)30
■ Effective Synthesis of Optically Active 3-Phenyl-3-(3-trifluoromethyl)diazirinyl Bishomophenylalanine Derivatives
Yuta Murai, Yasumaru Hatanaka, Yuichi Kanaoka, and Makoto Hashimoto*
Obihiro University of Agriculture and Veterinary Medicine, Inada-cho, Obihiro, Hokkaido 080-8555, Japan
Abstract
Effective incorporation of phenyldiazirine moiety on the acyl residue of L- and D- glutamic acid by Friedel-Crafts reactions with triflic acid developed simple preparation of bishomophenylalanine (bhPhe) for aromatics, which added a versatile and a reliable access to photoreactive peptide probes.Photoaffinity labeling is a useful biochemical method to reveal structural and functional relationships between low molecular weight bioactive compounds and biomolecules1; and for this, various photophores, such as phenyldiazirine, arylazide and benzophenone, are used. Although comparative irradiation studies of these three photophores in living cells indicated that a carbene precursor 3-phenyl-3-(3-trifluoromethyl)-3H-diazirine is the most promising photophore,1c the many steps for the synthesis of the 3-phenyl-3-(3-trifluoromethyl)diazirinyl ring has resulted in fewer applications in biomolecular studies than other photophores.2 Elucidations of side chain effects in amino acid are attractive subjects in peptide chemistry and photoaffinity labeling is one of the suitable methods for the purpose. Bishomophenylalanine (bhPhe, 2-Amino-5-phenylpentanoic acid) elongates two carbon atoms in a side chain of phenylalanine (Phe). Although a few of these 3-phenyl-3-(3-trifluoromethyl)diazirinyl bhPhe has been reported, the synthetic routes require condensation of corresponding photoreactive bromide and acetoamidemalonate, followed by an enzymatic resolution to isolate L-form in low yield.3
Asymmetric synthesis of a bhPhe derivative has been reported using several methodologies including hydrogenation of cyclic dehydrodipeptides,4 Suzuki-coupling,5 diastereoselective substitution6 and enzymatic reductive amination.7 These methods require the preparation of special reagents or precursors for the asymmetric synthesis of both enantiomers. Glutamic acid is one of the precursors for the effective synthesis of bhPhe, because Friedel-Crafts (F-C) acylation with γ-carboxyl, followed by reduction of introduced carbonyl moiety is one of the techniques used for retrosynthesis. It has been reported that L- N-phthaloyl glutamic anhydride was reacted F-C reaction in excess benzene (acyl acceptor) at 80 °C under AlCl3.8 It is too hard to apply the synthetic condition for precious and heat sensitive aromatics. In this paper we describe the synthesis of bhPhe derivatives including 3-trifluoromethyldiazirinyl moiety via asymmetric F-C reaction with stoichiometric amounts of glutamic acid analogues and aromatics. Only a few studies have reported F-C reactions with various aromatics and a side chain of glutamic acid derivatives, because many α-amino acids were less soluble in many organic solvent. For example, a F-C reaction of aspartic acid derivative, with a shorter side chain of glutamic acid and aromatics, was performed in excess aromatics as a solvent at high temperatures during long reaction times.9 The synthetic strategies were not applied to 3-phenyl-3-(3-trifluoromethyl)diazirinyl compounds, which are not stable in the presence of Lewis acid at temperatures above rt.10 Triflic acid was one of the good solvents for the α-amino acid and a promoter for F-C reactions. We have already reported catalytic amounts of triflic acid did not affect 3-trifluoromethyldiazirinyl moiety and promote F-C alkylation to a benzene ring with dichloromethyl methyl ether at low temperatures.2e Furthermore, it is preferable that the reaction proceed under the condition of stoichiometric amounts of glutamic acid derivatives and aromatics, and produce a homogeneous mixture. It is appropriate that triflic acid was used as a catalyst for the F-C reactions and a solvent for glutamic acid derivatives, as in accordance with previous information. N-TFA-Glu(Cl)-OMe 411 (L- or D- form) was selected as an acyl donor, because the compound had only one acylation site and both protective groups acquired a tolerance at the F-C condition. Several aromatics were subjected to the F-C reaction (Scheme 1). Triflic acid was added to the mixture of stoichiometric amounts of benzene 1 and 4 at 0 °C. The reaction mixture immediately became a clear solution and was stirred for an hour at 0 °C and then poured into ice-water and ethyl acetate to afford F-C products 5a over 90% yield. Further purification was not needed by 1H-NMR analysis after the reaction mixture was subjected to partition (1N HCl, saturated NaHCO3. and saturated NaCl). Toluene 2 afforded only p-substituted product 6a, however, anisole 3 afforded both p- (7a) and o- substituted (8a) products (p- : o- = 9 : 1) in high yield. These F-C products were subjected to hydrogenation of benzyl carbonyl group with Pd/C in acetic acid (5b-7b, 95-98%), followed by deprotection of trifluoroacetamide and methyl ester with 6N HCl at 80 °C in good yield to afford L- or D- bhPhe analogues (5c-7c, 98-99%). The stereochemistry of these compounds was determined [α]D12 and chiral HPLC (CHIROBIOTIC T; eluted with 10% EtOH – H2O; flow rate 1.0 ml/min; UV detection at 210 nm) for both enantiomers. Enantiomeric excess of all of the deprotected compounds was calculated >99% in order to succeed with asymmetric synthesis of bhPhe derivatives. The stereochemistry of the starting glutamic acid was retained for the each bhPhe (5c-7c) as expected. The synthetic routes were very useful because overall yields from F-C acylation to deprotection were very high.
The synthetic route applied to 3-phenyl-3-(3-trifluoromethyl)-3H-diazirine derivatives (Scheme 2). 3-Phenyl-3-(3-trifluoromethyl)-3H-diazirine was less reactive for nucleophilic substitutions than benzene, because quaternary carbon in 3-trifluoromethyldiazirine was polarizes slightly positive by two nitrogen and trifluoromethyl groups.13 3-Phenyl-3-(3-trifluoromethyl)-3H-diazirine, which has no substituent on the benzene ring, did not react with L- or D- 4 in TfOH at 0°C and the decomposed diazirinyl ring at higher temperatures. But we have already reported that 3-phenyl-3-(3-trifluoromethyl)-3H-diazirine is able to react to F-C alkylation in TfOH with dichloromethyl methyl ether in moderate yield.2e The results indicated that the reactivity of the acyl donor is also plays a critical role. 3-(3-Methoxyphenyl)-3-(3-trifluoromethyl)-3H-diazirine 914 will be a good acyl acceptor because it has a methoxy substituent.15
The mixture of stoichiometric amounts of 4 and 9 was dissolved in TfOH at 0 °C and maintained at the same temperature for 1h to afford only one F-C acylated product 1016 in moderate yield (76%). NOESY measurement of a methoxy group and an aromatic proton in 10 revealed that the F-C reaction proceeded at o-position against the methoxy substituent specifically, because steric hindrance between the diazirinyl ring and the substitute at p-position against the methoxy substituent may have contributed to the observation. It has already been reported that the diazirinyl N=N double bond was unstable under H2-Pd/C conditions.17 Specific reduction of benzyl carbonyl to methylene in a diazirinyl compound was performed with triethylsilane - TFA in good yield.18 The reduction of 10 with the condition for an hour at rt afforded 1119 in good yield. No decomposition of the diazirinyl ring was observed during the reaction. Deprotection of both protecting groups for 11 was performed using an alkaline condition to afford diazirinyl bhPhe 1220 in good yield (90%). Deprotection of 11 with an acidic condition (6N HCl at 80 °C in 6 h) afforded byproducts, in which diazirinyl moiety was broken, slightly. The stereochemistry of compound 12 was determined to be chiral HPLC (CHIROBIOTIC T; eluted with 10% EtOH – H2O; flow rate 1.0 ml/min; UV detection at 210 nm) for both enantiomers and the stereochemistry of the starting glutamic acid was retained for the each of the compounds.
We have demonstrated effective asymmetric synthesis of bhPhe from enantiopure glutamic acid derivative and aromatics in TfOH at low temperature, followed by reduction and deprotection in high yield. The protocol was applied to 3-(3-methoxyphenyl)-3-(3-trifluoromethyl)-3H-diazirine, which is one of the most useful photophores for photoaffinity labeling and is reported as unstable compounds for the F-C reaction at high temperatures. The results will be contributed to a better understanding of structure-activity relationships for the side chain of aromatic α-amino acids.
ACKNOWLEDGEMENTS
This research was partially supported by a Ministry of Education, Science, Sports and Culture Grant-in-Aid for Scientific Research on a Priority Area, 18032007 and for Scientific Research (C), 19510210. The authors would also like to thank Professor Masaru Hashimoto at Hirosaki University for the mass spectrometry measurements.
References
1. a) Y. Hatanaka, H. Nakayama, and Y. Kanaoka, Rev. Heteroatom Chem., 1996, 14, 213; b) A. K. Gillingham, F. Koumanov, M. Hashimoto, and G. D. Holman, ‘Membrane Transport : A Practical Approach’, ed. by S. A. Baldwin, Oxford University Press, Oxford, 2000, pp. 193-207; c) M. Hashimoto and Y. Hatanaka, Eur. J. Org. Chem., 2008, 2513. CrossRef
2. a) M. Nassal, J. Am. Chem. Soc., 1984, 106, 7540; CrossRef b) L. Beewen and H. Bayley, Anal. Biochem., 1985, 144, 132; CrossRef c) C. W. G. Fishwick, J. M. Sanderson, and J. B. C. Findlay, Tetrahedron Lett., 1994, 35, 4611; CrossRef d) M. Hashimoto, Y. Hatanaka, Y. Sadakane, and K. Nabeta, Bioorg. Med. Chem. Lett., 2002, 12, 2507; CrossRef e) H. Nakashima, M. Hashimoto, Y. Sadakane, T. Tomohiro, and Y. Hatanaka, J. Am. Chem. Soc., 2006, 128, 15092. CrossRef
3. a) K. Hashimoto, T. Yoshioka, C. Morita, M. Sakai, T. Okuno, and H. Shirahama, Chem. Lett., 1998, 203; CrossRef b) C. Morita, T. Okuno, K. Hashimoto, and H. Shirahama, Heterocycles, 2001, 54, 917. CrossRef
4. a) T. Kanmera, S. Lee, H. Aoyagi, and N. Izumiya, Tetrahedron Lett., 1979, 4483; CrossRef b) Y. Hashimoto, H. Aoyagi, M. Waki, T. Kato, and N. Izumiya, Int. J. Pept. Prot. Res., 1983, 21, 11.
5. P. N. Collier, A. D. Campbell, I. Patel, and R. J. K. Taylor, Tetrahedron Lett., 2000, 41, 7115. CrossRef
6. a) N. R. Treweeke, P. B. Hitchcock, D. A. Pardoe, and S. Caddick, Chem. Commun., 2005, 1868; CrossRef b) M. Yamada, N. Nagashima, J. Hasegawa, and S. Takahashi, Tetrahedron Lett., 1998, 39, 9019. CrossRef
7. Y. Asano, A. Yamada, Y. Kato, K. Yamaguchi, Y. Hibino, K. Hirai, and K. Kondo, J. Org. Chem., 1990, 55, 5567. CrossRef
8. G. Mohr, A. W. Frahm, and F. Zymalkowski, Liebigs Ann. Chem., 1972, 756, 103. CrossRef
9. a) D. G. Melillo, R. D. Larsen, D. J. Mathre, W. F. Shukis, A. W. Wood, and J. R. Colleluori, J. Org. Chem., 1987, 52, 5143; CrossRef b) A. G. Griesbeck, and H. Heckroth, Synlett, 1997, 11, 1243; CrossRef c) Q. Xu, G. Wang, X. Wang, T. Wu, X. Pan, A. S. C. Chan, and T. Yang, Tetrahedron: Asymmetry, 2000, 11, 2309. CrossRef
10. R. A. Moss, J.-M. Fede, and S. Yan, Org. Lett., 2001, 3, 2305. CrossRef
11. B. Badet, P. Vermoote, P.-Y. Haumont, F. Lederer, and F. Le Goffic, Biochemistry, 1987, 26, 1940. CrossRef
12. Y. Shimohigashi, S. Lee, and N. Izumiya, Bull. Chem. Soc. Jpn., 1976, 49, 3280. CrossRef
13. M. Hashimoto, Y. Kato, and Y. Hatanaka, Tetrahedron Lett., 2006, 47, 3391. CrossRef
14. Y. Hatanaka, M. Hashimoto, H. Kurihara, H. Nakayama, and Y. Kanaoka, J. Org. Chem., 1994, 59, 383. CrossRef
15. M. Hashimoto, Y. Kanaoka, and Y. Hatanaka, Heterocycles, 1997, 46, 119. CrossRef
16. 10 1H -NMR (CDCl3) δ:7.69 (1H, d, J = 8.0 Hz), 6.80 (1H, d, J = 8.0 Hz), 6.64 (1H, s), 4.60 (1H, td, J = 8.0, 4.6 Hz), 3.87 (3H, s), 3.74 (3H, s), 3.14-3.01 (2H, m), 2.36-2.29 (1H, m), 2.17-2.13 (1H, m). 13C-NMR (CDCl3) δ: 200.10, 170.96, 158.59, 157.06 (q, 2JCF = 37.6 Hz), 134.86, 130.94, 128.09, 121.73 (q, 1JCF = 273.9 Hz), 118.75, 115.60 (q, 1JCF = 288.3 Hz), 109.47, 55.60, 52.80, 52.41, 39.67, 28.33 (q, 2JCF = 40.8 Hz), 25.77. ESI-MS 456 (MH+). [α]D - +25.00 (c 2.0 CHCl3), [α]D - 26.50 (c 2.0 CHCl3).
17. Y. Ambroise, C. Miskowski, G. Djega-Mariadassou, and B. Rousseau, J. Org. Chem., 2000, 65, 7183. CrossRef
18. M. Hashimoto, Y. Hatanaka, and K. Nabeta, Heterocycles, 2003, 59, 395. CrossRef
19. 11 1H -NMR (CDCl3) δ: 7.08 (1H, d, J = 8.0 Hz), 6.71 (1H, d, J = 8.0 Hz), 6.57 (1H, s), 4.65 (1H, td, J = 7.7, 5.3 Hz), 3.78 (3H, s), 3.74 (3H, s), 2.65-2.56 (2H, m), 1.96-1.92 (1H, m), 1.76-1.73 (1H, m). 1.61-1.56 (2H,m). 13C-NMR (CDCl3) δ: 171.58, 157.72, 156.97 (q, 2JCF = 37.6 Hz), 131.86, 130.33, 128.35, 122.31 (q, 1JCF = 274.7 Hz), 118.89, 115.79 (q, 1JCF = 287.5 Hz), 108.16, 55.32, 52.90, 52.55, 31.60, 29.45, 28.60 (q, 2JCF = 40.4 Hz), 25.04. ESI-MS 442 (MH+). [α]D -+37.00 (c 1.0 CHCl3), [α]D - 37.00 (c 1.0 CHCl3).
20. 12 1H-NMR (CD3OD) δ: 7.21 (1H, d, J = 8.6 Hz), 6.75 (1H, d, J = 6.9 Hz), 6.64 (1H, s), 3.79 (3H, s), 3.52 (1H, dd, J = 6.6, 5.4 Hz), 2.65 (2H, t, J = 7.2 Hz), 1.89-1.64 (4H, m). 13C-NMR (CD3OD) δ: 174.54, 159.26, 133.75, 131.68, 128.94, 123.62 (q, 1JCF = 273.1 Hz), 119.78, 109.04, 56.13, 55.92, 32.08, 30.52, 29.54 (q, 2JCF = 40.0 Hz), 26.24. ESI-MS 332 (MH+). [α]D --6.0 (c 1.0 MeOH), [α]D -+5.5 (c 1.0 MeOH).