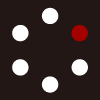
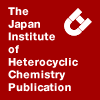
HETEROCYCLES
An International Journal for Reviews and Communications in Heterocyclic ChemistryWeb Edition ISSN: 1881-0942
Published online by The Japan Institute of Heterocyclic Chemistry
e-Journal
Full Text HTML
Received, 26th September, 2008, Accepted, 19th November, 2008, Published online, 21st November, 2008.
DOI: 10.3987/COM-08-S(D)35
■ Facile Syntheses of Three Ahp-Type Building Blocks with Complementary Reactivity
Wen Chen, Xiao Zheng, Yuan-Ping Ruan, and Pei-Qiang Huang*
Department of Chemistry and Key Laboratory for Chemical Biology of Fujian Province, College of Chemistry and Chemical Engineering, Xiamen University, Xiamen, Fujian 361005, China
Abstract
Two protected 3-amino-6-hydroxy-2-piperidone derivatives (Ahp, 12 and 6), as well as a thioether analogue (7) were synthesized starting from L-glutamic acid in four, five, and six steps respectively. The Ahp derivatives 12 and 6 are not only the structural feature found in many naturally occurring bioactive depsipeptides, but also precursors of the N-acyliminium such as D; while the (S)-thioether 7 was shown to be an effective synthetic equivalent of the novel 2-piperidone N-α-carbanion E via the lithium-naphthalenide (LN)-mediated reductive lithiation, capable of reacting with carbonyl compounds without an acidic α–position to give the α-hydroxyalkylation products.INTRODUCTION
6-Substituted 3-amino-2-piperidones are substructures found in many bioactive compounds. Among them, a number of 3-amino-6-hydroxy-2-piperidone (Ahp, 1)-containing depsipeptides were isolated from cyanobacteria (blue-green algae),1, 2 a prolific source of bioactive natural products.3 For example, micropeptin T-20 (Figure 1, 2), isolated from a cyanobacterium Microcystis aeruginosa,1e strongly inhibits chymotrypsin with an IC50 of 2.5 nM, but weakly inhibits tyrosinase with an IC50 of 5 mM;2c cyanopeptolin 954, isolated from the freshwater cyanobacterium Microcystis aeruginosa NIVA Cya 43, inhibits chymotrypsin with an IC50 value of 45 nM;1k nostopeptin BN920, isolated from the cyanobacterium Nostoc, inhibits chymotrypsin with an IC50 value of 31 nM.1k On the other hand, azabicyclo[X.Y.0]alkane amino acids4 such as 3-amino-2-piperidinone-6-carboxylic acid residue (Acp, 3),5 indolizidinone amino acid 4a,6a oxazolopiperidones 4b,6b and thiazolopiperidones 4c,6c are important peptide mimetic systems having restrained backbones, which could mimic the natural β-turn, arrange the correct side-chain functionalities for efficient interaction with the receptors. Due to the potential applicability for drug discovery, their synthesis have attracted much attention.6 In addition, (−)-slaframine7 (5) is an alkaloid produced by the mold Rhizoctonia leguminicola, which may be clinically useful for the treatment of disease arising from chlolinergic dysfunctions.8
Among the known methods2, 4, 6, 9, 10 for the construction of the structure motifs showed in Figure 1, those using L-glutamic acid as a chiron are attractive due to the high enantioselectivity.2, 6, 9, 10a In view of synthesizing the 2-piperidones with generic structure A according to Scheme 1,11 we now report the syntheses of novel chiral building blocks 6/7, and the preliminary study on the reductive α-hydroxyalkylation of 7.
RESULTS AND DISCUSSION
The synthesis of the chiral Ahp derivatives 6/7 is displayed in Scheme 2. L-Glutamic acid was converted to tetra-benzylated product (S)-8 by a known procedure (BnBr, Na2CO3/NaOH, H2O, reflux).12 Observation of the formation of a large amount of benzyl alcohol led us to use Na2CO3 as the only base, and add an additional amount of BnBr at the later period of the reaction, which improved the yield from 56% to 77% (Scheme 2). To check the optical purity of the product, a racemic sample (±)-8 was prepared in the same manner. Comparing the two products by HPLC analysis on a chiral column allowed concluding that the e.e. of (S)-8 is 100%, and no racemization occurred under the reaction conditions. Treatment of the glutamate 8 with diisobutylaluminum hydride (DIBAL-H) at 0 °C for 5 min. followed by careful work-up with 1N HCl allowed to reduce the less hindered ester group and gave the known alcohol (S)-912a, 12c in 85% yield.
For the lactamization, direct treatment of ester 9 with allylamine in refluxing THF was unsuccessful even in the presence of a large excess of allylamine. We next tried using allylamine-DIBAL-H complex for the amidation.13 After several trials, it was established that an 80% yield of 10 could be obtained by running the reaction at 45 °C for 5 hours. For the selective oxidation of the primary alcohol to aldehyde, PDC and Dess-Martin periodinane were tried as the oxidants. However, only 20-30% and 50-60% yields of 11 were obtained respectively. The yield was improved to about 86% by Swern oxidation. The product is a mixture of two tautomers, namely, amido aldehyde 11 and N,O-acetal 12. The amido aldehyde 11 converted spontaneously and completely to cyclic N,O-acetal 12 upon standing in a refrigerator for 2-3 days. The latter was determined to be a 3: 7 mixture of two diastereomers by 1H NMR recording in CD3CN.
Although N,O-acetal 12 can serve as a precursor of an N-acyliminium intermediate14 similar to B, in view of the generation of umpoled 2-piperidone N-α-carbanion C, a transformation of dibenzylamino-2-piperidone 12 to the corresponding benzylamino-2-piperidone 6 become necessary to avoid side reactions (on 13) such as racemization and proton exchange15 under strongly basic conditions required for the reductive lithiation. Thus benzylamino-2-piperidone 12 was monodebenzylated to give benzylamino-2-piperidone 6 by treatment with CAN16 in a mixed solvent system (MeCN/ H2O, 5: 1 v/v) at rt for 20 min. The monodebenzylated product 6 was obtained in 76% yield as a 9:1 mixture of two diastereomers according to the 1H NMR analysis. For the conversion of the N,O-acetal 6 to thioether 7, the catalyst system PPTS-TsOH was inefficient. Fortunately, in the presence of boron trifluoride etherate, the desired thioether 7 was obtained in 56% yield. Although the Ahp derivative 6 and analogue 7 show the same diastereomeric ratio, it was recognized that thioether 7 was formed via the N-acyliminium ion intermediate14 D.
The facile formation and good stability of both the Ahp derivatives 12 and 6, and its analogue 7 is notable, because other Ahp systems were shown to be difficult to form under a variety of conditions, and were labile.10a The obtained results will be useful for the synthesis of Ahp-containing depsipeptide natural products.
With thioether 7 in hand, we then proceeded to test its reductive lithiation.17 Thus the secondary amine 7 was first deprotected with n-BuLi (1.5 mol. equiv.), and then treated with a freshly prepared 1.5 M solution of lithium-naphthalenide (LN) in THF (3.0 mol. equiv.) to generate the dianion E, which was confirmed by capture with methanol to give 3-benzylamino-2-piperidone 14. With the in situ generation of the dianion intermediate E secured we then investigated its reaction with other electrophiles. However attempted trapping of the dianion intermediate E with acetone led only to the reduced product 14 in 53% yield (Scheme 3). This result implicated that the dianion E behaved as a strong base, which abstracted easily acidic α-proton of acetone. Indeed, treatment of the dianion E with benzaldehyde gave the desired coupling products 15 in 50% yield, alongside with 10% of the reduced product 14. Compound 15 is an inseparable mixtures of four diastereomers. The ratios were determined by off-line HPLC-MS analysis to be 44:41:10:5. Reductive coupling with pivaldehyde gave the coupling products 16 (Yield: 34%) as an inseparable mixtures of four diastereomers in a ratio of 30:31:21:18, alongside with 7% of the reduced product 14. Because the diastereomers are difficult to separate, the stereochemistry of the diastereomers were not determined.
Although the diastereoselectivities of the reductive α–hydroxyalkylation of thioether 7 are only modest or mediocre, the successful reaction of the dianion E with aldehydes is worthy of note. It was reported that attempted reaction of the similar dianion F, generated in situ from the reductive lithiation of thioether 17 with 4.0 molar equivalents of LN (2 min), with benzaldehyde failed (Scheme 4).17e In that case benzyl alcohol formed due to rapid competitive reduction. The difference between the two cases may be attributed to, on one hand, that only 3.0 molar equivalents of LN were used in our case, and on the other hand, that the conditions we used (LN, 30 min) allows a clean formation of the dianion E. This means that at least for similar systems, one pot, but stepwise reductive lithiation-nucleophilic addition is a better procedure than a Barbier-like procedure for the reductive α-hydroxyalkylation. In addition, our system (E) allows overcoming the problem of proton exchange as that observed for the dianion G,15 allowing hence the desired C-6 reductive α-hydroxyalkylation of the Ahp derivative.
CONCLUSION
In summary, a practical approach to 3-amino-6-hydroxy-2-piperidone (Ahp) derivatives 12 and 6 from L-glutamic acid is disclosed. The building block 6 is not only a protected substructure (Ahp) found in several bioactive natural products, but can also serve as a precursor of the corresponding N-acyliminium D as demonstrated by the synthesis of thioether 7. The thioether 7 can serve as a synthetic equivalent of novel 2-piperidone N-α-carbanion E, via deprotonation and LN-mediated reductive lithiation. Conditions were established for the capture of the 2-piperidone dianion E with carbonyl compounds without an acidic α-proton, that constitutes a novel method for the α-hydroxyalkylation of 2-piperidone derivatives.
EXPERIMENTAL
General
Melting points were determined on a Yanaco MP-500 micromelting point apparatus and are uncorrected. Infrared spectra were measured with a Nicolet Avatar 360 FT-IR spectrometer using film KBr pellet technique. 1H NMR spectra were recorded in CDCl3 on a Bruker Avance DPX 400 MHz spectrometer with tetramethylsilane as an internal standard. Chemical shifts are expressed in δ (ppm) units downfield from TMS. Mass spectra were recorded by a Bruker Dalton Esquire 3000 plus liquid chromatography–mass spectrum (direct injection) and ABI 3200Q trap liquid chromatography–mass spectrum. Optical rotations were measured with a Perkin–Elmer 341 automatic polarimeter and Rudolph Autopol IV automatic polarimeter. Flash column chromatography was carried out with silica gel (300–400 mesh). THF was distilled over sodium. Dichloromethane was distilled over P2O5.
(S)-2-Dibenzylaminopentanedioic acid dibenzyl ester (8)
L-Glutamic acid (1.00 g, 6.80 mmol) was dissolved in water (13.6 mL) together with Na2CO3 (2.88 g, 27.2 mmol) in a two-necked flask equipped with a refluxing condenser and a dropping funnel. The mixture was refluxed and benzyl bromide (4.6 g, 27.2 mmol) was slowly added. After stirring for 1 h, an additional amount of benzyl bromide (1.2 g, 6.8 mmol) was added. The resulting mixture was stirred for another 30 min and then cooled to rt. The mixture was extracted with EtOAc (3 × 15 mL). The combined extracts were washed with brine, dried over anhydrous Na2SO4, filtered and concentrated in vacuum. Filtration of the residue through a short pad of column eluting with EtOAc/P.E. (v/v 1:15) yielded the known fully benzylated glutamate 812a,12c (2.62 g, yield: 77%) as a colorless oil. [α]D20 –70.8 (c 1.3, CHCl3); IR (film) 3085, 3062, 3030, 2945, 2846, 1731, 1602, 1585, 1495, 1454, 1415 cm-1; 1H NMR (400 MHz, CDCl3) δ 2.01-2.10 (m, 2H), 2.28-2.39 (m, 1H), 2.42-2.53 (m, 1H), 3.42 (t, J = 7.6 Hz, 1H, CHN), 3.50 (d, J = 13.7 Hz, 2H, PhCH2), 3.87 (d, J = 13.7 Hz, 2H, PhCH2), 4.92 (d, J = 12.4 Hz, 1H, PhCH2), 4.97 (d, J = 12.4 Hz, 1H, PhCH2), 5.12 (d, J = 12.2 Hz, 1H, PhCH2), 5.23 (d, J = 12.2 Hz, 1H, PhCH2), 7.10-7.40 (m, 20H, Ar-H) ppm; 13C NMR (100 MHz, CDCl3) δ 24.4, 30.8, 53.6, 54.6, 59.9, 66.27, 66.33, 127.2, 127.4, 128.3, 128.5, 128.5, 128.7, 128.7, 128.8, 129.1, 129.3, 136.1, 136.2, 139.4, 172.3, 172.9 ppm; MS (ESI) m/z 508 (M+H+, 100%), 530 (M+Na+, 90%).
(S)-2-Dibenzylamino-5-hydroxylpentanoic acid benzyl ester (9)
To a cooled (0 oC) solution of compound 8 (1.00 g, 1.97 mmol) in THF (10 mL) was slowly added a 1.2 M solution of DIBAL-H in hexane (4.9 mL, 5.91 mmol). The mixture was stirred for 10 min, and quenched with 1 N HCl (8 mL). The resulting mixture was extracted with EtOAc (3 × 10 mL). The combined extracts were washed with brine, dried over anhydrous Na2SO4, filtered and concentrated in vacuum. Filtration of the residue through a short pad of column eluting with EtOAc/P.E. (v/v 1:6) yielded the known compound 912a,12c (674 mg, yield: 85%) as a colorless oil. [α]D20 –81.4 (c 0.9, CHCl3); IR (film) 3406, 3085, 3062, 3029, 2948, 2867, 1728, 1494, 1453, 1372, 1210 cm-1; 1H NMR (400 MHz, CDCl3) δ 1.41-1.53 (m, 2H), 1.64-1.76 (m, 1H), 1.77-1.85 (m, 1H), 3.37 (t, J = 7.5 Hz, 1H, CHN), 3.43-3.47 (m, 2H, CH2OH), 3.50 (d, J = 13.8 Hz, 2H, PhCH2), 3.87 (d, J = 13.8 Hz, 2H, PhCH2), 5.15 (d, J = 12.2 Hz, 1H, PhCH2), 5.27 (d, J = 12.2 Hz, 1H, PhCH2), 7.15-7.45 (m, 15H, Ar-H) ppm; 13C NMR (100 MHz, CDCl3) δ 25.8, 29.3, 54.5, 60.5, 62.4, 66.1, 127.1, 128.3, 128.4, 128.6, 128.7, 128.9, 136.0, 139.4, 172.8 ppm; MS (ESI) m/z 404 (M+H+, 100%), 426 (M+Na+, 92%).
(S)-2-Dibenzylamino-5-hydroxylpentanoic acid N-allylamide (10)
A solution of DIBAL-H (1.2 M in hexane, 3.0 mL, 3.75 mmol) was added to a cooled (0-5 oC) solution of allylamine (0.3 mL, 4.1 mmol) in THF (1.5 mL) under nitrogen. The mixture was allowed to warm up and stirred at rt for 2 h. The concentration of the thus obtained DIBAL-H-H2NCH2CH=CH2 complex was about 0.75 M, and was used directly for aminolysis. To a solution of 9 (300 mg, 0.75 mmol) in THF (1.5 mL) was added the DIBAL-H-H2NCH2CH=CH2 complex (4.8 mL, 3.75 mmol) under nitrogen at rt. After being stirred at 45 oC for 5 h, the reaction was cooled to 0 oC, and then quenched with 1 N HCl (5 mL). The mixture was extracted with EtOAc (3 × 5 mL). The combined extracts were washed with brine, dried over anhydrous Na2SO4, filtered and concentrated in vacuum. Filtration of the residue through a short pad of column eluting with EtOAc/P.E. (v/v 1:2) yielded 10 (210 mg, yield: 80%) as a colorless oil. [α]D20 – 47.5 (c 0.2, CHCl3); IR (film) 3373, 3083, 3061, 3027, 2930, 1659, 1602, 1513, 1494, 1453, 1373 cm-1; 1H NMR (400 MHz, CDCl3) δ 1.59-1.78 (m, 2H), 1.83-1.92 (m, 1H), 1.92-2.03 (m, 1H), 2.80 (s, 1H, OH), 3.15 (dd, J = 3.5, 7.3 Hz, 1H, CHN), 3.50-3.66 (m, 2H, CH2OH), 3.54 (d, J = 13.5 Hz, 2H, PhCH2), 3.72 (d, J = 13.5 Hz, 2H, PhCH2), 3.80-3.95 (m, 2H, CH2NH), 5.10-5.18 (m, 2H, CH2=CH), 5.68-5.78 (m, 1H, CH2=CH), 7.20-7.38 (m, 10H, Ar-H) ppm; 13C NMR (100 MHz, CDCl3) δ 20.1, 31.5, 41.7, 54.5, 61.8, 61.8, 116.5, 127.4, 128.6, 128.7, 134.2, 138.8, 173.7 ppm; MS (ESI) m/z 353 (M+H+, 100%). HRMS calc for C22H29N2O2: 353.2229; found: 353.2236.
(3S,6R/S)-1-Allyl-3-dibenzylamino-6-hydroxypiperidin-2-one (12)
To a cooled solution of (–78 oC) oxalyl chloride (1.12 g, 8.87 mmol) in anhydrous CH2Cl2 (16 mL) was slowly added a solution of anhydrous DMSO (1.33 g, 17.05 mmol) in anhydrous CH2Cl2 (6 mL) under nitrogen. The mixture was stirred for 15 min, then a solution of 10 (2.5 g, 7.1 mmol) in anhydrous CH2Cl2 (6 mL) was slowly added. After stirring for 15 min., anhydrous Et3N (2 mL) was added and the mixture was stirred for 15 min at –78 oC. After addition of another 2 mL of Et3N, the flask was chilled with a ice-bath and the mixture stirred for 15 min. Water (5 mL) was added, and the mixture was extracted with CH2Cl2 (3 × 1 5 mL). The combined extracts were washed with brine, dried over anhydrous Na2SO4, filtered and concentrated in vacuum. Filtration of the residue through a short pad of column eluting with EtOAc/P.E. (v/v 1:5) yielded 11 (2.15 g, yield: 86%) as a pale yellow oil, which was converted into 12 as a 3:7 mixture of two inseparable diastereomers (determined by 1H NMR) upon standing in a refrigerator for 2-3 days. IR (film) 3381, 3083, 3061, 3027, 2926, 2852, 1621, 1585, 1493, 1453, 1414, 1370, 1326, 1266 cm-1; 1H NMR (400 MHz, CD3CN, data of the major diastereomer read from the mixture) δ 1.65-1.90 (m, 2H), 1.90-2.00 (m, 1H), 2.13-2.25 (m, 1H), 3.28 (t, J = 5.9, 11.1 Hz, 1H), 3.70 (d, J = 14.1 Hz, 2H), 3.80-3.88 (m, 1H), 3.98 (d, J = 14.1 Hz, 2H), 4.26-4.35 (m, 1H), 4.78-4.85 (m, 1H), 5.09-5.21 (m, 2H, CH2=CH), 5.75-5.88 (m, 1H, CH2=CH), 7.20-7.49 (m, 10H, Ar-H) ppm; 13C NMR (100 MHz, CD3CN) (major diastereomer) δ 22.6, 30.8, 44.3, 55.0, 58.6, 79.2, 117.4, 126.8, 128.2, 128.9, 134.4, 140.8, 171.1 ppm; MS (ESI) m/z 351 (M+H+, 100%). HRMS calc for C22H27N2O2: 351.2073; found: 351.2086.
(3S,6R/S)-1-Allyl-3-benzylamino-6-hydroxypiperidin-2-one (6)
Ceric ammonium nitrate (822 mg, 1.5 mmol) was added portion-wise to a stirring solution of 12 in MeCN-H2O (5:1). After stirring at rt for 30 min, the reaction was quenched by addition of a saturated aqueous NaHCO3 solution and stirred vigorously for 10 min. After extracting with Et2O, the combined extracts were washed with brine, dried over anhydrous Na2SO4, filtered and concentrated in vacuum. Filtration of the residue through a short pad of column eluting with EtOAc/P.E. (v/v 2:1) yielded 6 (140 mg, yield: 76%, pale yellow oil) as an inseparable 9:1 mixture of two diastereomers determined by 1H NMR. IR (film) 3280, 3062, 3027, 2925, 1656, 1519, 1495, 1452, 1420, 1334, 1251 cm-1; 1H NMR (400 MHz, CDCl3, data of the major diastereomer read from the mixture)δ 1.82-2.07 (m, 4H), 3.27 (t, J = 7.6 Hz, 1H), 3.72 (d, J = 13.1 Hz, 1H), 3.68-3.80 (m, 1H), 3.88 (d, J = 13.1 Hz, 1H), 4.34-4.43 (m, 1H), 4.87 (dd, J = 2.0, 3.6 Hz, 1H, HOCHN), 5.11-5.21 (m, 2H, CH2=CH), 5.73-5.86 (m, 1H, CH2=CH), 7.20-7.40 (m, 5H, Ar-H) ppm; 13C NMR (100 MHz, CDCl3, major diastereomer) δ 21.8, 28.6, 47.5, 51.1, 57.5, 79.1, 117.4, 127.3, 128.2, 128.3, 128.6, 133.3, 139.0, 170.8 ppm; MS (ESI) m/z 261 (M+H+, 100%). HRMS calc for C15H21N2O2: 261.1603; found: 261.1598.
(3S,6R/S)-1-Allyl-3-benzylamino-6-phenylsulfanylpiperidin-2-one (7)
To a solution of 6 (230 mg, 0.88 mmol), thiophenol (0.11 mL, 1.06 mmol), and CaCl2 (5 mg) in 11 mL of anhydrous CH2Cl2 was added BF3•Et2O (0.11 mL, 0.88 mmol) at 0 oC. After stirring for 1 h, the reaction was quenched with aqueous NaHCO3. The mixture was extracted with CH2Cl2 (3 × 15 mL). The combined extracts were washed with brine, dried over anhydrous Na2SO4, filtered and concentrated in vacuum. Filtration of the residue through a short pad of column eluting with EtOAc/P.E. (v/v 1:3) yielded 7 (175 mg, yield: 56%, pale yellow oil) as an inseparable 9: 1 mixture of two diastereomers as determined by 1H NMR. IR (film) 3321, 3059, 3026, 2948, 1651, 1582, 1494, 1439, 1412, 1352, 1319, 1252 cm-1; 1H NMR (400 MHz, CDCl3, data of the major diastereomer read from the mixture) δ 2.00-2.45 (m, 4H), 3.28 (dd, J = 6.2, 11.5 Hz, 1H), 3.72 (dd, J = 7.9, 15.3 Hz, 1H), 3.78 (d, J = 13.2 Hz, 1H, PhCH2), 3.88 (d, J = 13.2 Hz, 1H, PhCH2), 4.75-4.79 (m, 1H), 4.85-4.93 (m, 1H), 5.09-5.22 (m, 2H, CH2=CH), 5.70-5.82 (m, 1H, CH2=CH), 7.20-7.50 (m, 10H, Ar-H) ppm; 13C NMR (100 MHz, CDCl3) (major diastereomer) δ 23.0, 28.0, 47.5, 50.9, 57.6, 67.9, 118.2, 127.0, 128.2, 128.3, 128.5, 129.3, 132.6, 133.7, 133.9, 140.0, 170.9 ppm; MS (ESI) m/z 353 (M+H+, 100%). HRMS calc for C21H25N2OS: 353.1688; found: 353.1688.
General procedure for the one-pot synthesis of compounds 14-16 from 7
A solution of 7 (0.28 mmol) in 2.8 mL of dry THF at –78 oC was treated with n-BuLi (2.5 M solution in n-hexane, 0.42 mmol) and freshly prepared lithium naphthalenide (LN, 1.5 M solution in THF, 0.85 mmol). After stirring for 30 min at –78 oC, an electrophile (1.4 mmol) was added. The stirring continued at –78 oC for 1 h, and then was allowed to warm to 0 oC. The reaction was quenched with a saturated aqueous NH4Cl, and the mixture was extracted with CH2Cl2 (3 × 5 mL). The combined extracts were washed with brine, dried over anhydrous Na2SO4, filtered, and concentrated in vacuum. Filtration of the residue through a short pad of column eluting with EtOAc/P.E. (v/v 1:3) afforded the desired product.
(3S)-1-Allyl-3-benzylaminopiperidin-2-one (14)
Quenching agent: MeOH (0.1 mL); product: 14, 27 mg, yield: 56%, pale yellow oil. [α]D20 15.9 (c 0.8, CHCl3); IR (film) 3318, 3026, 2924, 2855, 1639, 1493, 1440, 1415, 1334, 1278, 1198 cm-1; 1H NMR (400 MHz, CDCl3) δ 1.60-1.83 (m, 2H), 1.89-2.00 (m, 1H), 2.11-2.21 (m, 1H), 2.60-2.75 (br s, 1H), 3.18-3.32 (m, 3H), 3.78 (d, J = 13.2 Hz, 1H, PhCH2), 3.90 (d, J = 13.2 Hz, 1H, PhCH2), 3.85-3.98 (m, 1H), 4.04 (dd, J = 5.9, 15.0 Hz, 1H, CH2CH=CH2), 5.10-5.20 (m, 2H, CH2=CH), 5.68-5.82 (m, 1H, CH2=CH), 7.20-7.40 (m, 5H, Ar-H) ppm; 13C NMR (100 MHz, CDCl3) δ 20.8, 27.2, 47.2, 49.6, 51.5, 57.4, 117.4, 127.0, 128.1, 128.4, 132.6, 139.8, 170.6 ppm; MS (ESI) m/z 245 (M+H+, 100%). Anal. Calcd for C15H20N2O: C, 73.74; H, 8.25; N, 11.47. Found: C, 73.56; H, 8.48; N, 11.68.
(3S,6R/S,1’R/S)-1-Allyl-3-benzylamino-6-(hydroxylphenylmethyl)piperidin-2-one (15)
Electrophile used: PhCHO (0.07 mL, 0.71 mmol); product 15: 25 mg, yield: 50%, colorless oil. HPLC-MS analysis showed that the product is a mixture of four diastereomers. The diastereomeric ratio was determined by HPLC analysis employing Shim-pack VP-ODS (150×4.6) (MeOH/H2O 60:40 (v/v), 1.0 mL/min, λ= 215 nm). The results are as follows: t1) 11.1 min (44%), t2) 13.9 min (41%), t3) 16.8 min (10%), t4) 18.5 min (5%). A pure sample of one diastereomer was obtained after repeated chromatographic separation. [α]D20 12.0 (c 0.1, CHCl3), IR (film) 3321, 3062, 3026, 2924, 2853, 1632, 1513, 1494, 1452, 1415, 1331, 1259, 1096 cm-1; 1H NMR (400 MHz, CDCl3) δ 1.40-1.50 (m, 2H), 1.78-1.90 (m, 1H), 2.04-2.15 (m, 1H), 3.20 (dd, J = 5.4, 10.6 Hz, 1H), 3.60-3.67 (m, 1H), 3.77 (d, J = 13.2 Hz, 1H, PhCH2), 3.78-3.85 (m, 1H), 3.88 (d, J = 13.2 Hz, 1H, PhCH2), 4.39-4.46 (m, 1H), 5.10 (d, J = 3.4 Hz, 1H), 5.23-5.32 (m, 2H, CH2=CH), 5.84-5.96 (m, 1H, CH2=CH), 7.20-7.40 (m, 10H, Ar-H) ppm; 13C NMR (100 MHz, CDCl3) δ 14.1, 19.5, 25.3, 47.4, 51.5, 57.1, 60.4, 62.1, 71.2, 117.8, 125.6, 127.1, 127.6, 128.2, 128.4, 128.4, 133.6, 173.4 ppm; MS (ESI) m/z 351 (M+H+, 100%). HRMS calc for C22H27N2O2: 351.2073; found: 351.2082.
(3S,6R/S,1’R/S)-1-Allyl-3-benzylamino-6-(1-hydroxyl-2,2-dimethylpropyl)piperidin-2-one (16)
Electrophile used: 2,2-dimethylpropionaldehyde (0.77 mL, 0.71 mmol); product: 16 (16 mg, yield: 34%), pale yellow oil. HPLC-MS analysis showed that the product is a mixture of four diastereomers. The diastereomeric ratio was determined by HPLC analysis employing Shim-pack VP-ODS (150×4.6) (MeOH/H2O 60:40 (v/v), 1.0 mL/min, λ= 215 nm). The results are as follows: t1) 15.3 min (30%), t2) 19.7 min (31%), t3) 22.1 min (21%), t4) 34.8 min (18%). A pure sample of one diastereomer was obtained after repeated chromatographic separation. [α]D20 2.2 (c 0.5, CHCl3); IR (film) 3400, 2953, 2869, 1632, 1479, 1453, 1360, 1255, 1219 cm-1; 1H NMR (400 MHz, CDCl3) δ 0.95 (s, 9H, C(CH3)3), 1.43-1.57 (m, 1H), 1.85-1.94 (m, 1H), 1.98-2.18 (m, 2H), 3.28 (dd, J = 4.6, 11.5 Hz, 1H), 3.50 (d, J = 1.1 Hz, 1H), 3.60-3.70 (m, 2H), 3.80 (d, J = 13.2 Hz, 1H, PhCH2), 3.87 (d, J = 13.2 Hz, 1H, PhCH2), 4.34-4.43 (m, 1H), 5.13-5.21 (m, 2H, CH2=CH), 5.75-5.87 (m, 1H, CH2=CH), 7.20-7.40 (m, 5H, Ar-H); 13C NMR (100 MHz, CDCl3) δ 20.5, 26.5, 27.1, 34.6, 47.5, 51.9, 57.2, 57.8, 77.6, 117.4, 126.9, 128.2, 128.4, 133.6, 173.9; MS (ESI) m/z 331 (M+H+, 100%). HRMS calc for C20H31N2O2: 331.2386; found: 331.2382.
ACKNOWLEDGEMENTS
The authors are grateful to the NSFC (20572088, 20832005) and the program for Innovative Research Team in Science & Technology (University) in Fujian Province for financial support. We thank Professor Y. F. Zhao for the use of her Bruker Dalton Esquire 3000 plus LC-MS apparatus.
References
1. For selected examples of the isolation of the Ahp-containing peptides, see: (a) G. R. Pettit, Y. Kamano, C. L. Herald, C. Dufresne, R. L. Cerny, D. L. Herald, J. M. Schmidt and H. Kizu, J. Am. Chem. Soc., 1989, 111, 5015; CrossRef (b) K. Ishida, M. Murakami, H. Matsuda and K. Yamaguchi, Tetrahedron Lett., 1995, 36, 3535; CrossRef (c) H. J. Shin, M. Murakami, H. Matsuda, K. Ishida and K. Yamaguchi, Tetrahedron Lett., 1995, 36, 5235; CrossRef (d) K. Kaya, T. Sano, K. A. Beattie and G. A. Codd, Tetrahedron Lett., 1996, 37, 6725; CrossRef (e) T. Okano, T. Sano and K. Kaya, Tetrahedron Lett., 1999, 40, 2379; CrossRef (f) Y. Itou, K. Ishida, H. J. Shin and M. Murakami, Tetrahedron, 1999, 55, 6871, and references cited therein; CrossRef (g) G. G. Harrigan, H. Luesch, W. Y. Yoshida, R. E. Moore, D. G. Nagle and V. J. Paul,, J. Nat. Prod., 1999, 62, 655; CrossRef (h) K. Fujii, K. Sivonen, E. Naganawa and K. I. Harada, Tetrahedron, 2000, 56, 725; CrossRef (i) A. M. Burja, B. Banaigs, E. Abou-Mansour, J. G. Burgess and P. C. Wright, Tetrahedron, 2001, 57, 9347; CrossRef (j) B. Bister, S. Keller, H. I. Baumann, G. Nicholson, S. Weist, G. N. Jung, R. D. Süssmuth and F. Jüttner, J. Nat. Prod., 2004, 67, 1755; CrossRef (k) E. Elert, L. Oberer, P. Merkel, T. Huhn and J. F. Blom, J. Nat. Prod., 2005, 68, 1324; CrossRef (l) A. Plaza and C. A. Bewley, J. Org. Chem., 2006, 71, 6898. CrossRef
2. For synthetic studies on the 3-amino-6-hydroxy-2-piperidone-containing depsipeptides, see: (a) F. Yokokawa, A. Inaizumi and T. Shioiri, Tetrahedron Lett., 2001, 42, 5903; CrossRef (b) F. Yokokawa and T. Shioiri, Tetrahedron Lett., 2002, 43, 8673; CrossRef (c) F. Yokokawa, A. Inaizumi and T. Shioiri, Tetrahedron, 2005, 61, 1459; CrossRef (d) After the total synthesis of the proposed structure of micropeptin T-20 (Figure 1, 2), the structure of the natural micropeptin T-20 was questioned.
3. For a review, see: V. Reshef and S. Carmeli,, Tetrahedron, 2001, 57, 2885. CrossRef
4. For reviews, see: (a) H. G. Lombart and W. D. Lubell, ‘Peptides: Chemistry, Structure and Biology’, ed. by P. T. P. Kaumaya, R. S. Hodges, ESCOM Sci. Pub. B. V., Leiden, 1996, pp. 695; (b) S. Hanessian, G. McNaughton-Smith, H. G. Lombart and W. D. Lubell, Tetrahedron, 1997, 53, 12789; CrossRef (c) W. Maison and A. H. G. P. Prenzel, Synthesis, 2005, 1031. CrossRef
5. D. S. Kemp and P. E. McNamara, J. Org. Chem., 1984, 49, 2286. CrossRef
6. (a) H. G. Lombart and W. D. Lubell, J. Org. Chem., 1996, 61, 9437; CrossRef (b) M. A. Estiarte, M. Rubiralta, A. Diez, M. Thormann and E. Giralt, J. Org. Chem., 2000, 65, 6992; CrossRef (c) U. Nagai and K. Sato, Tetrahedron Lett., 1985, 26, 647; CrossRef For reviews, see: (d) J. A. Robinson, Synlett, 2000, 429; CrossRef (e) W. Maison and A. H. G. P. Prenzel, Synthesis, 2005, 1031. CrossRef
7. (a) S. D. Aust, H. P. Broquist and K. L. Rinehart Jr., J. Am. Chem. Soc., 1966, 88, 2879; CrossRef (b) R. A. Gardiner, K. L. Rinehart Jr., J. J. Snyder and H. P. Broquist, J. Am. Chem. Soc., 1968, 90, 5639. CrossRef
8. W. J. Croom, W. M. Hagler, M. A. Froetschel and A. D. Johnson, J. Anim. Sci., 1995, 73, 1499.
9. For recent reviews on the piperidines and piperidine alkaloids, see: (a) M. Rubiralta, E. Giralt and A. Diez, ‘Piperidines Structure, Preparation, Reactivity, and Synthetic Applications of Piperidines and Its Derivatives’, Elsevier: Amsterdam, 1991, ; (b) M. Schneider, ‘Pyridine and Piperidine Alkaloids: An Update in Alkaloids: Chemical and Biochemical Perspectives’, Vol. 10, ed. by S. W. Pelletier, Elsevier Science, Oxford, 1996, pp. 155-299; (c) S. Laschat and T. Dickner, Synthesis, 2000, 1781; CrossRef (d) P. M. Weintraub, J. S. Sabol, J. M. Kane and D. R. Borcherding, Tetrahedron, 2003, 59, 2953. CrossRef
10. (a) M. A. Estiarte, M. V. N. Souza, X. Rio, R. H. Dodd, M. Rubiralta and A. Diez, Tetrahedron, 1999, 55, 10173; CrossRef (b) R. J. B. H. N. van. den. Berg, D. Noort, E. S. Milder-Enacache, G. A. van. den. Marel, J. H. v. Boom and H. P. Benschop, Eur. J. Org. Chem., 1999, 2593; CrossRef (c) T. Granier and A. Vasella, Helv. Chim. Acta, 1998, 81, 865. CrossRef
11. Previous reports from these laboratories on the synthetic methodology based on L-glutamic acid, see: (a) P. Q. Huang, L. X. Liu, B. G. Wei and Y. P. Ruan, Org. Lett., 2003, 5, 1927; CrossRef (b) P. Q. Huang, B. G. Wei and Y. P. Ruan, Synlett, 2003, 1663; CrossRef (c) L. X. Liu, Y. P. Ruan, Z. Q. Guo and P. Q. Huang, J. Org. Chem., 2004, 69, 6001; CrossRef (d) Y. P. Ruan, B. G. Wei, X. Q. Xu, G. Liu, D. S. Yu, L. X. Liu and P. Q. Huang, Chirality, 2005, 17, 595; CrossRef (e) B. G. Wei, J. Chen and P. Q. Huang, Tetrahedron, 2006, 62, 190; CrossRef (f) C. G. Feng, J. Chen, J. L. Ye, Y. P. Ruan, X. Zheng and P. Q. Huang, Tetrahedron, 2006, 62, 7459; CrossRef (g) G. Liu, J. Meng, C. G. Feng and P. Q. Huang, Tetrahedron: Asymmetry, 2008, 19, 1297; CrossRef (h) L. X. Liu, Q. L. Peng and P. Q. Huang, Tetrahedron: Asymmetry, 2008, 19, 1200; CrossRef (i) P. Q. Huang, G. Chen and X. Zheng, J. Heterocycl. Chem., 2007, 44, 499. CrossRef
12. (a) G. Kokotos, J. M. Padron, T. Martin, W. A. Gibbons and V. S. Martin, J. Org. Chem., 1998, 63, 3741; CrossRef (b) M. Rodriquez, I. Bruno, E. Cini, M. Marchetti, M. Taddei and L. Gomez-Paloma, J. Org. Chem., 2006, 71, 103; CrossRef (c) M. Rodriquez and M. Taddei, Synthesis, 2005, 493. CrossRef
13. P. Q. Huang, X. Zheng and X. M. Deng, Tetrahedron Lett., 2001, 42, 9039. CrossRef
14. For reviews on α-amidoalkylation, see: (a) H. E. Zaugg, Synthesis, 1984, 85 and 181; For reviews on the N-acyliminium ions, see: (b) W. N. Speckamp and H. Hiemstra, Tetrahedron, 1985, 41, 4367; CrossRef (c) W. N. Speckamp and M. J. Moolenaar, Tetrahedron, 2000, 56, 3817; CrossRef (d) S. K. Bur and S. F. Martin, Tetrahedron, 2001, 57, 3221; CrossRef (e) C. M. Marson, Arkivoc, 2001, 1, 1, at www.arkat-usa.org; (f) B. E. Maryanoff, H. C. Zhang, J. H. Cohen, I. J. Turchi and C. A. Maryanoff, Chem. Rev., 2004, 104, 1431; CrossRef (g) J. Royer, Chem. Rev., 2004, 104, 2311. CrossRef
15. P. Q. Huang, X. Zheng, S. L. Wang, J. L. Ye, L. R. Jin and Z. Chen, Tetrahedron: Asymmetry, 1999, 10, 3309. CrossRef
16. S. D. Bull, S. G. Davies, G. Fenton, A. W. Mulvaney, R. S. Prasad and A. D. Smith, J. Chem. Soc., Perkin Trans. 1, 2000, 3765. CrossRef
17. For reviews on the reductive lithiation of phenyl thioethers, see: (a) T. Cohen and M. Bhupathy, Acc. Chem. Res., 1989, 22, 152; CrossRef (b) M. Yus, Chem. Soc. Rev., 1996, 25, 155; CrossRef (c) T. Cohen, Pure & Appl. Chem., 1996, 68, 913; CrossRef (d) R. Chinchilla, C. Nájera and M. Yus, Tetrahedron, 2005, 61, 3139; CrossRef For selective examples, see: (e) M. Bartels, J. Zapico and T. Gallagher, Synlett, 2004, 2636. CrossRef