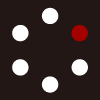
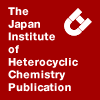
HETEROCYCLES
An International Journal for Reviews and Communications in Heterocyclic ChemistryWeb Edition ISSN: 1881-0942
Published online by The Japan Institute of Heterocyclic Chemistry
e-Journal
Full Text HTML
Received, 27th September, 2008, Accepted, 21st November, 2008, Published online, 25th November, 2008.
DOI: 10.3987/COM-08-S(D)37
■ 5,5’-Bipyridyl-2,4,6,2’,4’,6’-hexaone Derivatives (Hydurilic Acids): Syntheses, Mechanism of C-C-Bond Formation and Properties of the Dimeric Barbituric Acid Derivatives
Christa E. Müller,* Carolin Roegler, and Jörg Hockemeyer
Pharmaceutical Institute Poppelsdorf, University of Bonn, Kreuzbergweg 26, D-53115 Bonn, Germany
Abstract
A series of hydurilic acid derivatives (5,5’-bipyrimidinyl-2,4,6,2’,4’,6’-hexaones) including several new derivatives was synthesized from 5,6-diaminouracils. Mechanisms for their formation are proposed and discussed. Furthermore, a new method for the preparation of pyrimidine-2,4,5,6-tetraone-5-oxime derivatives (violuric acids) was found starting from 5-amino-6-nitrosouracils.INTRODUCTION
In the course of our efforts to synthesize novel xanthine derivatives as adenosine receptor antagonists1 we had developed a general synthesis of 1-monosubstituted xanthines.2 The conversion of 3-substituted 6-aminouracils3 to the corresponding 5,6-diaminouracil derivatives (1) and subsequent condensation with formic acid for 20 to 40 h generally provided 1-monosubstituted xanthines (2) in high yields2 (Scheme 1). One striking exception had been observed: the reaction of 5,6-diamino-3-(2-phenylethyl)uracil 3 with formic acid under reflux conditions did not lead to the corresponding 1-substituted xanthine derivative 4, but gave an unknown compound (5) in high yield. The desired 1-(2-phenylethyl)xanthine 4 could meanwhile be synthesized from 3 in a yield of 92 % using triethylorthoformate after a reaction time of only 4 h under reflux conditions.2 The present study was aimed at elucidating the structure of the new product 5, the synthesis and characterization of analogs, and the mechanism of their formation.
RESULTS AND DISCUSSION
The structure of product 5 was elucidated by a combination of analytical methods including chromatography, spectroscopy, and elemental analysis. All observations taken together proved the formation of a symmetrical 1,1’-bis-(2-phenylethyl)-substituted [5,5’]bipyrimidinyl-2,4,6,2’,4’,6’-hexaone 5 (Scheme 1). Presumably a mixture of stereoisomers was formed which could not be isolated. The fact that the CH-acidic isomers can easily be converted either into the corresponding enantiomer or into the meso-form could be a possible explanation for the difficulty in separating the stereoisomers.
The related, N-unsubstituted compound 12 (Scheme 2) with the trivial name hydurilic acid was first prepared and characterized by Bayer as early as 1863.4 The 5,5’-connected dimer of barbituric acid had been obtained from 5-hydroxypyrimidine-2,4,6-trione (5-hydroxybarbituric acid, 6) by heating in glycerol at 150 °C in a yield of 50 %,4 or in the absence of solvent at 190-200 °C in a lower yield of 34 %.5 Alternatively, 5-amino-1,3-dimethylpyrimidine-2,4,6-trione (5-amino-1,3-dimethylbarbituric acid, 7) has been used as starting material. In the presence of two equivalents of methyl isocyanate as ammonia scavenger a 54 % yield of tetramethyl hydurilic acid (13) was obtained after a reaction time of 16 h at 100 °C in a sealed tube.6 Based upon these findings a plausible pathway, involving three starting molecules, can be postulated to describe the aforementioned reactions (Scheme 2). Condensation reactions, including the formation of a carbon-carbon bond, are followed by elimination of pyrimidine-2,4,5,6-tetraone (alloxane, 10) or 5-imino-1,3-dimethylpyrimidine-2,4,6-trione (11), respectively.
In this context it is noteworthy that the by-product of the described reactions, alloxane (10), was reported to provide hydurilic acid itself at 170 °C in a sealed tube. The presence of a large excess of water as well as the absence of water was shown to prevent the formation of 12.7 Due to the observed water-sensitivity alloxane hydrate 14 (see scheme 3) could be postulated as the initial molecule for the formation of 12 from 10. Several different reaction steps have to be considered involving nucleophilic attacks, elimination of water or other molecules, and disproportionation reactions.
The formation of tetramethyl hydurilic acid 13 (Scheme 4) by refluxing of 5,6-diamino-1,3-dimethyluracil 15 in aqueous solution containing various concentrations of mineralic acid was first described by Bredereck using sulfuric acid;8 he obtained 13 in 19 % yield. Independently, Blicke and Godt obtained a maximal yield of 54 % of 13 by using an aqueous solution of two equivalents of hydrochloric acid in water.9 From the reaction mixtures both groups isolated the colorless pyrazine derivative 1,3,7,9-tetramethyl-(1H,3H,7H,9H)-pyrimido[5,4-g]pteridine-2,4,6,8-tetrone (16)10 in yields of 31 %,9 and 23 %, respectively8 in addition to hydurilic acid derivative 13. A careful reinvestigation of this reaction showed that the yellow isomer 1,3,6,8-tetramethyl(1H,3H,6H,8H)pyrimido[4,5-g]pteridine-2,4,7,9-tetrone (17)11 was formed as well. Due to its physicochemical properties 17 remained in the mother liquor, when the reaction mixture was worked up as described by the authors (Scheme 4). It was found by Blicke and Godt9 that the formation of 13 became predominant when the concentration of hydrochloric acid was increased. Our reinvestigation showed that the formation of pyrazine derivatives could not completely be suppressed, even in the presence of a large excess of hydrochloric acid.
In contrast to Blicke and Godt9 Bredereck presented a proposal for a detailed reaction mechanism correlating the formation of the reduced hydurilic acid derivative with the oxidized pyrazine ring system.8 Since the nature of the redox reactions proposed by Bredereck8 still remained unclear, additional experiments were performed to obtain more information about this reaction. Assuming that under vigorous aqueous acidic conditions at least a partial hydrolysis of the diaminouracil 15 would take place prior to any C-C-bond formation, a hydrolysis product of 15 should also lead to the hydurilic acid derivative 13 under the same reaction conditions.
5-Amino-1,3-dimethylbarbituric acid (7, Scheme 2) was selected to investigate its potential conversion to 1,1’,3,3’-tetramethylhydurilic acid (13) under reflux conditions in the presence of an excess of aqueous hydrochloric acid. Starting compound 7 could easily be prepared by reduction of the appropriate pyrimidine-2,4,5,6-tetraone-5-oxime (violuric acid) derivative 2212 using sodium dithionite in aqueous ammonia solution (Scheme 5).
The formation of 22 was achieved by hydrolysis of the corresponding 6-amino-5-nitrosouracil 18 in diluted aqueous hydrochloric acid under reflux conditions. To prove the general applicability of this method, a range of mono- and disubstituted violuric acid derivatives (22-25) was prepared. In all cases highly pure products were obtained with yields of around 80 % for the di-substituted and ca. 40 % for the mono-substituted derivatives. We observed that the cleavage of the derivatives 22-25 by diluted hydrochloric acid proceeded very slowly even under reflux conditions. To the best of our knowledge this route has not yet been described for the preparation of violuric acid derivatives.
The reduction of the purple solutions of violuric acid derivatives 22 and 23 at 60 °C provided the colorless 5-aminobarbituric acids 7 and 26 after short reaction times of less than 5 min. Although the colorless solids are extremely sensitive to oxidation, derivative 7 could be characterized by 1H and 13C NMR spectroscopy. The solution of 7 in d6-DMSO had to be measured immediately since it soon turned to deep purple due to the oxidation of 7 by the solvent yielding the oxidation product murexoin 27 (confirmed by TLC analysis).13 Further characterization of 7 and 26 was achieved by derivatization with an excess of benzoyl chloride under reflux conditions yielding the corresponding 4,6-dialkyl-2-phenyl-4H,6H-oxazolo[5,4-d]pyrimidine-5,7-diones 28 and 29.14,15
Aqueous solutions of freshly prepared 7 containing an excess of hydrochloric acid were refluxed, varying the reaction times from 1 h up to 18 h (scheme 6). After cooling to rt the aqueous colorless reaction mixtures were extracted with dichloromethane. Due to complex separation problems of water-soluble reaction products, the aqueous phases were not investigated in detail, but were considered to contain mainly decomposition products, such as methylammonium chloride. In all cases evaporation of the organic solvent led to a solid residue consisting of a mixture of colorless products, none of which could be identified as tetramethylhydurilic acid 13 or a pyrazine derivative. One main product could easily be crystallized from diethyl ether. An 1H NMR spectrum showing only two singlets at 3.08 (3H) and 4.68 ppm (2H), a 13C NMR spectrum with four peaks at 25.9, 68.0, 156.0 and 170.4 ppm and a molecular mass of 155 pointed to a small molecule. Together with the melting point of 133 °C (Lit.: 133-134 °C)16 all data indicated the formation of 3-methyloxazolidine-2,4-dione 3516 (Scheme 6). After a reaction time of 1 h compound 35 was obtained in a yield of 17 %, and after a reaction time of 3 h the yield was increased to 35 %. After 18 h a significant decrease in the yield was observed, and hydroxyacetic acid (37) was detected in the crude dichloromethane extract. Besides compound 35 another small heterocyclic compound was observed in the crude extract. Comparison with literature NMR data confirmed the formation of 3-methylimidazolidine-2,4-dione (3-methylhydantoine, 36).17 Compound 36 was obtained in a yield of about 5 % after a reaction time of 3 h. Longer reaction times did not significantly alter the yield of 36, but the enrichment of 36 in comparison with 35 in the crude organic extract indicated that the hydantoin derivative was more stable than the oxazolidine derivative under vigorous aqueous acidic conditions. Without providing any experimental details, Techow12 had reported on the crystallization of 5,5’-dihydroxy-1,3,1’,3’-tetramethyl[5,5’]bipyrimidyl-2,4,6,2’,4’,6’-hexaone (38, Scheme 6) from the neutralized reaction mixture after refluxing a solution of 7 in aqueous hydrochloric acid. This observation, which would involve a C-C-bond formation, could not be confirmed by our own experiments.
The formation of oxazolidine-2,4-diones (e.g. 35) by a ring-contraction reaction from 5-hydroxybarbituric acid derivatives (e.g. 30) is well known18 but has typically been performed in alkaline solution or under virtually neutral conditions.19 Several plausible mechanisms have been discussed19 and led to an understanding of the observed reaction (Scheme 6). Presuming the hydrolysis of 7 to the 5-hydroxybarbituric acid derivative 30, the following ring contraction leads to the intermediate 31. A competing process is the intramolecular reaction of the starting compound 7 yielding the intermediate 32. Hydrolysis of the resulting amide derivatives (31 and 32) followed by decarboxylation of the intermediates 33 and 34 would complete the formation of 35 and 36 (Scheme 6).
In consideration of the above mentioned results, the mechanism proposed by Bredereck8 concerning the formation of hydurilic acids from 5,6-diaminouracils upon catalysis by mineralic acids in water under reflux conditions has to be revised. Apparently the complete hydrolysis to a 5-hydroxybarbituric acid derivative could not be the first step since this would be followed by ring contraction.
In order to obtain more information about the actual intermediates, the reaction of 5,6-diamino-1,3-dimethyluracil (15) was investigated in more detail (Scheme 7). As shown above, conversion of the vinylic amino group of 5-amino-1,3-dimethyl-6-nitrosouracil (18) into a carbonyl group yielding violuric acid derivative 22 was completed within less than 10 min already (Scheme 5). We therefore stopped the reaction of 15 in refluxing hydrochloric acid after 0.5 h already: the formation of 13 as well as that of pyrazine derivatives 16 and 17 (see Scheme 4) was observed to be already at an advanced stage after that short reaction time. TLC analysis of the crude reaction mixtures showed that further products had been formed besides 13, 16 and 17. Two further products were isolated by extraction of the crude reaction mixture and subsequent column chromatography: 1,3-dimethylimidazolidine-2,4,5-trione (dimethylparabanic acid, 45)20 and 1,3-dimethylbarbituric acid (42).21 Compound 45 as a final product might be explained through decomposition of the alloxane derivative 44 similar to the formation of the 5-membered ring systems described in Scheme 6. The formation of 42 is more surprising since this derivative was neither observed nor expected before in this context. However, the formation of 42 could be explained by oxidation of the pyrazine precursor 39 (resp. 40) by the starting compound 15 forming the final pyrazine derivative 16 (resp. 17) as well as the intermediate 41, which is subsequently hydrolyzed to 42 (Scheme 7).
Another possibility for the formation of compound 45 has to be considered. The pyrazine derivatives 16 (resp. 17) may be reduced by the 5,6-diaminouracil 15 leading to 39 (resp. 40). The resulting intermediate 43 would lead to the stable dimethylparabanic acid (45) as described above (Scheme 8).
During the course of the above mentioned reaction a purple coloration was observed after ca. 10 min. Within the following 10 min the reaction mixture turned yellow. These changes of color could be associated with the formation of the well-known deep violet purpuric acid derivatives (compound 27, Scheme 5). They consist of two pyrimidyl ring systems linked by a nitrogen atom forming a large delocalized π-electron system. Structurally similar pyrazine precursors can be postulated for the reaction investigated and could provide a plausible explanation for the purple color. Subsequent oxidation to the final pyrazine derivatives would explain a further change of color from purple to yellow. The remaining color can be assigned to the yellow pyrazine isomer 17. These observations indicate that the formation of pyrazines should be completed after a reaction time of only 25 min.
Since the 5-unsubstituted barbituric acid (42) could still be detected after a reaction time of 12 h, the compound obviously does not participate in the formation process of hydurilic acid derivative 13. This observation is well in agreement with the finding by Biltz that 42 itself does not lead to 13 under strongly acidic conditions in the presence of an oxidizing agent.5 However, not even traces of the oxazolidine derivative 35 could be extracted from the reaction mixture. The absence of 35 is a further proof that a complete hydrolysis of the 5,6-diaminouracil 15 to the 5-hydroxybarbituric acid 30 cannot be the initial step in the reaction investigated. Both amino groups of the starting compound 15 appear to be essential not only for the formation of pyrazines but also for the C-C-bond formation of two uracil ring systems finally resulting in the C5-C5’-bond of derivative 13 (Scheme 4).
In order to gain more information about the properties of hydurilic acid derivatives a series of derivatives were prepared. Tetramethylhydurilic acid (13) was best prepared as previously described by Blicke and Godt.9 Further purification was achieved by column chromatography. 5,6-Diamino-1-alkyluracils were selected as starting compounds for 1,1’-disubstituted hydurilic acids. Since the reaction of 1-methyl- or 1-propyl-5,6-diaminouracil (46 and 47) with concentrated formic acid did not lead to hydurilic acids (see Scheme 1) the reaction was performed with an excess of hydrochloric acid under reflux conditions. After reaction times of less than 1 h the crude hydurilic acid derivatives 48 and 49, contaminated by corresponding pyrazine derivatives, precipitated from the cold reaction mixtures (Scheme 9). Due to its physicochemical properties the purification of 48 (a purity of about 80 % was determined for the crude product) proved to be extraordinary difficult and could not satisfactorily be achieved with standard methods. In contrast, compound 49 could easily be obtained in a pure form by recrystallisation from isopropanol. Both 1,1’-substituted hydurilic acids were obtained in moderate yields of about 20 %. Consistent with our theory as described above, longer reaction times did not result in higher yields.
The conversion of the diaminouracil 46 confirmed our proposed reaction mechanism. Next to the desired compound 46 and pyrazine derivatives the formation of the corresponding C5-unsubstituted barbituric acid (50)21 and the parabanic acid derivative 5120 were observed as side-products and characterized by comparison with literature NMR data. The small heterocyclic compounds 50 and 51 were extracted from the crude reaction mixture with dichloromethane and subsequently purified by column chromatography (Scheme 9).
The preparation of hydurilic acids from 5,6-diaminouracils was observed to be associated with a number of difficulties: a) the yield is limited due to the reaction mechanism, b) the co-formation of pyrazine derivatives appears to be inevitable or can at least not easily be suppressed, c) especially less lipophilic derivatives cannot be purified easily. The easy formation of the 1,1’-di-(2-phenylethyl)hydurilic acid 5 (Scheme 1) from the corresponding 5,6-diaminouracil 3 remained an exception.
5,5’-Unsubstituted hydurilic acids show characteristic NMR spectra as discussed below. In order to obtain a broader basis of information 5,5’-disubstituted hydurilic acid derivatives were additionally prepared (Scheme 10). The known 5-bromo-5’-methoxytetramethylhydurilic acid (52) was obtained as previously described starting from tetramethylhydurilic acid 13 with an excess of bromine in methanol under reflux conditions.22 The methylation of 13 using an excess of methyl iodide in DMF in the presence of a potassium carbonate under microwave irradiation (10 W, 80 °C, 1.5 bar, 0.5 h) yielded hexamethylhydurilic acid (53, Scheme 10).
The previously described hydurilic acid derivatives 13, 48, 52 and 53 as well as the new derivatives 5 and 49 were investigated by 1H and 13C NMR spectroscopy.22 The 1H NMR spectra of all N-methyl substituted derivatives showed singlets for the N-methyl groups in the typical range from 3.08 (48) to 3.37 ppm (52). The C5 H-atom of the C5-unsubstituted derivatives were exchangeable, and appeared as very broad singlets within a small range from 4.85 to 4.99 ppm (compounds 5, 13, 48 and 49, measured in DMSO-d6). The NH-signals for the derivatives 5, 48 and 49 were consistently observed around 11.7 ppm. In the 13C NMR spectra the NCH3 signals for the derivatives 13, 48, 52 and 53 appeared in the expected region around 29 ppm. The chemical shifts for unsubstituted C5-atoms (derivatives 5, 13, 48 and 49) were observed around 48.5 ppm, showing very little variation. One remarkable effect could be observed comparing the spectra of tetramethylhydurilic acid 13 measured in CHCl3 and in DMSO-d6, respectively. The peak for the C5-atoms at 48.2 ppm appeared broadened and not very clear when DMSO-d6 was used as a solvent, while in CDCl3 the signal appeared very clear and well pronounced. This effect could be explained by a reduced tendency of the CH-acidic compound to form enol-tautomers in the non-polar CDCl3. The well pronounced C2 signals of all derivatives investigated did not show any significant variations and were observed in the typical region around 150 ppm. In contrast to this the C4- and the C6-signals, respectively, of the C5-unsubstituted derivatives 5, 13, 48 and 49, appeared around 166 ppm appeared broadened and were only weak. Again, this effect could be explained by the formation of tautomers. The spectra of the C5-substituted derivatives 52 and 53 provided the proof for this assumption: the formation of tautomers could be blocked by substitution of the C5-positions so that the C4/C6-signals appeared clearly and well defined.
CONCLUSIONS
A series of differently substituted hydurilic acids was prepared from 5,6-diaminouracil derivatives including several new derivatives. The reaction mechanism was investigated in detail, and a new pathway was postulated based on the isolation and structural determination of intermediate products. The products were characterized by spectroscopic methods and 5,5’-unsubstituted derivatives not previously described in the literature were shown to tautomerize in polar solvents such as DMSO.
EXPERIMENTAL
1,1’,3,3’-Tetramethyl[5,5’]bipyrimidinyl-2,4,6,2’,4’,6’-hexaone (13): The compound was prepared as previously described.9 1H NMR (CDCl3) ppm: 3.31 (s, 12H, NCH3), 4.65 (s sharp, 2H, C5H); 13C NMR (CDCl3) ppm: 29.1 (NCH3), 48.72 (s sharp, C5), 151.0 (C2), 166.28 (C4/C6); 1H NMR (DMSO-d6) ppm: 3.15 (s, 12H, NCH3), 4.99 (s br), 2H, C5H); 13C NMR (DMSO-d6) ppm: 28.6 (NCH3), 48.7 (br, C5), 151.4 (C2), 167.1 (br, C4/C6).
1,1’-Dimethyl[5,5’]bipyrimidinyl-2,4,6,2’,4’,6’-hexaone (48):8,22 Freshly prepared 5,6-diamino-1-methyluracil (46, 1 g, < 6 mmol, containing an undetermined amount of water) was refluxed in a mixture of concentrated hydrochloric acid (5 mL) and water (5 mL). After 1 h the reaction was stopped and the yellow solution was evaporated under reduced pressure. The residue was crystallized from a minimum of water at 4 °C. After ca. 12 h the precipitate was filtered off under reduced pressure, washed with little water and dried at 70 °C yielding 160 mg of a pale yellow (due to impurities of the corresponding yellow pyrazine derivatives) solid. For the (colorless) 48 a purity of about 80 % was determined by 1H NMR spectroscopy. Until now no satisfactory purification method could be developed. 1H NMR (DMSO-d6) ppm: 3.08 (s, 6H, CH3), 4.85 (s, 2H, C5-H), 11.72 (s, 2H, NH); 13C NMR (DMSO-d6) ppm: 27.68, 48.31 (C5), 150.9 (C2), 167.6 and 168.4 (br, C4,C6).
1,1’-Dipropyl[5,5’]bipyrimidinyl-2,4,6,2’,4’,6’-hexaone (49): Freshly prepared 5,6-diamino-1-propyluracil (47, ca. 850 mg, 4,6 mmol) was refluxed in a mixture of concentrated hydrochloric acid (3 mL) and water (5 mL). After 30 min a colorless solid precipitated and the reaction was stopped. The precipitation was completed at 4 °C and the crude product (270 mg) was filtered off under reduced pressure. The solid was washed with water and recrystallized from isopropanol yielding 155 mg of colorless crystals (20 %). Alternatively the crude product could be purified by column chromatography on silica gel: eluting first with CH2Cl2/MeOH (5 : 1) in order to separate the impurities (pyrazine derivatives) and finally with CH2Cl2/MeOH (3 : 1): mp 215 °C; 1H NMR (DMSO-d6) ppm: 0.81 (br s, 6H, CH3), 1.48 (br s , 4H, CH2), 3.65 (br s, 4H, NCH2), 4.87 (s, 2H, C5-H), 11.68 (s, 2H, NH); 13C NMR (DMSO-d6) ppm: 11.0 (CH3) , 20.8 (CH2), 42.3 (NCH2), 48.5 (C5), 150.7 (C2), 167-169 (br, C4,C6); EIMS (m/z, %) 338 (M+ , 10) 170 (M/2H+, 100). Anal. Calcd for C14H18N4O6: C, 49.7; H, 5.36; N, 16.56. Found: C, 49.6; H, 5.33; N, 16.53.
1,1’-Diphenylethyl[5,5’]bipyrimidinyl-2,4,6,2’,4’,6’-hexaone (5): 5,6-Diamino-3-(2-phenethyl)uracil (3, 1.2 g, 4.9 mmol) was suspended in 30 mL of formic acid (98%). The mixture was refluxed for 28 h. The excess of formic acid was distilled off and the residue was suspended in water. The precipitate was collected by filtration and washed with water. Further purification was achieved by dissolution of the compound in 1 M NaOH solution, precipitation by acidification using acetic acid and crystallization from a small amount of CHCl3 yielding colorless crystals (66 % yield): mp 195 °C; 1H NMR (CDCl3): 2.87 (m, 4H, PhCH2), 4.05 (m, 4H, NCH2), 4.53 (s, 2H, C5-H), 7.17-7.30 (m, 10H, Harom.), 8.61 (s, 2H, NH) ppm; 13C NMR (CDCl3) ppm: 33.8, 43.3, 48.2, 126.8, 128.6, 128.88, 128.92, 138.44, 137.50, 149.2 (C2), 166.0 (br, C4,C6) ppm; EIMS (m/z, %) 462 (M+, 9) 104 ([CH2CH2Ph]+, 100), FDMS (m/z, %) 462 (M+ , 100); Anal. Calcd for C24H22N4O6: C, 62.3; H, 4.79; N, 12.1. Found: C, 62.2; H, 4.73; N, 12.2. TLC analysis: (CHCl3 : MeOH = 3:1, Rf = 0.95).
5-Bromo-5’-methoxy-1,1’,3,3’-tetramethyl[5,5’]bipyrimidinyl-2,4,6,2’,4’,6’-hexaone (52): The compound was prepared from 13 as previously described22 (mp ca. 240 °C (dec.); lit mp 245-247 °C).22 1H NMR (CDCl3) ppm: 3.31 (s, 3H, OCH3), 3.36 (s, 6H, NCH3), 3.37 (s, 6H, NCH3). 13C NMR (CDCl3) ppm: 29.6 (NCH3), 30.4 (NCH3), 56.9 (OCH3), 64.9 (C5), 84.9 (C5), 150.2 and 150.5 (C2 or C2’), 161.5 and 162.8 (C4/C6 or C4’/C6’).
1,1’,3,3’,5,5’-Hexamethyl[5,5’]bipyrimidinyl-2,4,6,2’,4’,6’-hexaone (53):22 A suspension of 13 (60 mg, 0.19 mmol), K2CO3 (0.055 g, 0.38 mmol) and methyl iodide (1 mL, 16 mol) in DMF (1 mL) was placed in a 10 mL pressure tube and irradiated by microwave for 0.5 h (10 W, 80 °C, 1.5 bar). Subsequently the mixture was evaporated under reduced pressure. After the addition of water (75 mL) the residue was extracted twice, with 50 mL CH2Cl2 each. The organic extracts were dried over K2CO3, filtered, evaporated and concentrated. The product was crystallized with Et2O: 50 mg colorless crystals (77 %), mp 258.7-259.1 °C; 1H NMR (CDCl3) ppm: 1.86 (s, 6H, C5CH3), 3.25 (s, 12 H, NCH3); 13C NMR (CDCl3) ppm: 21.5 (C5CH3), 29.1 (NCH3), 55.7 (C5), 150.3 (C2), 170.6 (C4/C6); EIMS (m/z, %) 338 (M+ , 2) 170 (M/2H+, 100). Anal. Calcd for C14H18N4O6: C, 49.7; H, 5.36; N, 16.56. Found: C, 49.5; H, 5.61; N, 16.60.
1,3-Dimethylpyrimidine-2,4,5,6-tetraone-5-oxime (22): A suspension of 6-amino-5-nitroso-1,3-dimethyluracil 18 (4.6 g, 25 mmol) in 35 mL of diluted hydrochloric acid (2 M) was heated under reflux until an almost decolorized mixture was obtained (ca. 10 min). The colorless product was crystallized from the reaction mixture at 4 °C, filtered off under reduced pressure, washed with water and dried at 70 °C (84 % yield): mp 144.9-145.5 °C (lit.23, mp 141 °C). 1H NMR (CDCl3): (a single tautomer) 3.38 (s, 3H, NCH3), 3.41 (s, 3H, NCH3), 15.91 (br s, 1H) ppm; 13C NMR (CDCl3): 27.9 (NCH3), 29.0 (NCH3), 135.5, 149.2, 156.2, 160.4 ppm; 1H NMR (DMSO-d6): 3.12 (s, 3H, NCH3), 3.17 (s, 3H, NCH3), 14.70 (br s, 1H) ppm; 13C NMR (DMSO-d6): 27.7 (NCH3), 28.4 (NCH3), 135.8, 150.8, 153.7, 158.3 ppm.
1-Methylpyrimidine-2,4,5,6-tetraone-5-oxime (24): A suspension of 6-amino-5-nitroso-3-methyluracil 20 (2.1 g, 12 mmol) in 15 mL of diluted hydrochloric acid (2 M) was heated under reflux until an almost decolorized mixture was obtained (ca. 10 min). The colorless product was crystallized from the reaction mixture at 4 °C, filtered off under reduced pressure, washed with water and dried at 70 °C (39 % yield): mp 208.3 °C (lit.24, mp 202-203 °C (dec.)); 1H NMR (DMSO-d6): (mixture of two tautomer forms ca. 1 : 0.9) 3.05 (s, 3H, NCH3), 3.11 (s, 3H, NCH3), 11.51 (br s, 1H), 11.06 (s, 1H), 14.70 (br s, 2H) ppm; 13C NMR (DMSO-d6) ppm: 26.8 (NCH3), 27.5 (NCH3), 135.9, 136.4, 150.18, 150.24, 154.1, 154.8, 158.3, 159.1 ppm.
1,3-Dibutylpyrimidine-2,4,5,6-tetraone-5-oxime (23):25 A mixture of finally ground 6-amino-1,3-dibutyl-5-nitrosouracil 19 (3.2 g, 12 mmol) and 30 mL of diluted hydrochloric acid was heated under stirring and vigorous reflux. After cooling to rt the mixture was diluted with 100 mL of water and extracted with 150 mL of Et2O. The ether extract was filtered, dried over MgSO4, filtered again and evaporated. The product was crystallized from petroleum ether (82 % yield): mp 74.5-75.2 °C; 1H NMR (CDCl3) ppm: (a single tautomer) 0.94 (m, 6H, CH3), 1.36 (m, 4H, CH2), 1.36 (m, 4H, CH2), 1.60 (m, 4H, CH2), 3.93 (m, 4H, NCH2), 16.00 (br s, 1H), 13C NMR (CDCl3) ppm: 13.56, 13.62, 20.0, 29.7, 29.8, 41.6, 42.5, 135.5, 148.8, 156.0, 160.4.
1-Prop-2-ynylpyrimidine-2,4,5,6-tetraone-5-oxime (25): A suspension of 6-amino-5-nitroso-3-prop-2-ynyluracil 21 (1.2 g, 6 mmol) in 9 mL of diluted hydrochloric acid (2 M) was heated under reflux for 10 min. The hot solution was filtered in order to remove a small amount of decomposed material. Subsequently the off-white solid was allowed to crystallize at 4 °C, filtered under reduced pressure, washed with water and dried at 70 °C (39 % yield): mp > 173 °C (dec.); 1H NMR (DMSO-d6): (mixture of two tautomers, ca. 1 : 0.9) 3.13 (t, J = 2.5 Hz, 1H), 3.14 (t, J = 2.5 Hz, 1H), 4.41 (d, J = 2.5 Hz, 2H), 4.47 (d, J = 2.5 Hz , 2H), 11.64 ( br s, 1H), 11.73 (s, 1H), 14.76 (br s, 2H) ppm; 13C NMR (DMSO-d6): 29.6, 30.2, 73.62, 73.64, 78.86, 78.88, 135.64, 136.30, 149.3, 149.4, 153.2, 163.7, 158.1, 158.3 ppm; EIMS (m/z, %) 195 (M+ , 100).
5-Amino-1,3-dimethylpyrimidine-2,4,6-trione (7):12 A deep-purple solution of 1,3-dimethylpyrimidine-2,4,5,6-tetraone-5-oxime 22 (1 g, 5.4 mmol) in 20 mL 12.5% aq NH4OH was prepared and heated at 60 °C. Subsequently sodium dithionite (ca. 2.5 g) was rapidly added under stirring until the color turned yellowish. The solution was concentrated under reduced pressure to two-thirds of the original volume. After cooling to rt the air-sensitive, colorless crystals were filtered off and washed with water, EtOH and Et2O and directly used for following steps (56 % yield): mp 200 °C; 1H NMR (DMSO-d6): 3.08 (s, 6H, NCH3), 8.12 (s br, 3H, NH2, OH) ppm; 13C NMR (DMSO-d6): 26.9 (NCH3), 83.3, 152.1, 158.6 ppm.
5-Amino-1,3-dibutylpyrimidine-2,4,6-trione (26): A deep-purple mixture of 1,3-dibutylpyrimidine-2,4,5,6-tetraone-5-oxime 23 (1 g, 3.9 mmol) in 30 mL 12.5% aq NH4OH was prepared and heated at 80 °C. Subsequently the solution was decolorized by the addition of sodium dithionite (2.7 g) upon stirring. The mixture was heated at 60 °C for additional 10 min, filtered to remove some unconverted starting material and concentrated under reduced pressure to two-thirds of the original volume. After cooling to rt colorless needles were rapidly filtered off, washed with water and directly used for following steps (yield: 1.0 g, max. 3.7 mmol, containing an undetermined percentage of water). Due to its extreme air-sensitivity compound 26 was only characterized by derivatization with benzoyl chloride yielding 4,6-dibutyl-2-phenyl-4H,6H-oxazolo[5,4-d]pyrimidine-5,7-dione (29).
4,6-Dimethyl-2-phenyl-4H,6H-oxazolo[5,4-d]pyrimidine-5,7-dione (28): The compound was prepared as previously described:14 mp 234 °C (lit., mp 247 °C;15 240-242 °C26).
1H NMR (CDCl3): 3.42 (s, 3H, NCH3), 3.63 (s, 3H, NCH3) 7.46-7.48 (m, 3H, Harom.), 8.01-8.04 (m, 2H, Harom.) ppm; 13C NMR (CDCl3): 28.8 (NCH3), 30.6 (NCH3), 112.6 (C3a), 125.5, 126.5, 129.0, 131.3 (Carom.), 150.2, 154.4, 156.5, 156.6 (C2, C4, C6, C7a) ppm.
4,6-Dibutyl-2-phenyl-4H,6H-oxazolo[5,4-d]pyrimidine-5,7-dione (29): A mixture of freshly prepared 5-amino-1,3-dibutylpyrimidine-2,4,6-trione 26 (1 g, 3.7 mmol) was refluxed with an excess of benzoyl chloride (3 mL, 25.8 mmol) for 0.5 h, cooled to rt, treated with 5% NaOH solution (50 mL), refluxed again for 10 min and finally stirred at rt until the viscous residue turned into a solid. The solid was extracted twice with 50 mL CH2Cl2 each, dried over K2CO3, filtered over silica gel and concentrated under reduced pressure leading to a highly pure product. Colorless needles were obtained by recrystallization from CH2Cl2 : petroleum ether (1 : 6) (ca. 30 % yield): mp 91 °C, 1H NMR (CDCl3): 0.93 (t, J = 7.6 Hz, 3H, CH3), 0.99 (t, J = 7.6 Hz, 3H, CH3), 1.37 (m, 2H, CH3CH2), 1.42 (m, 2H, CH3CH2), 1.63 (m, 2H, CH2CH2CH2), 1.80 (m, 2H, CH2CH2CH2), 4.02 (m, 2H, NCH2), 4.08 (m, 2H, NCH2), 7.45-7.50 (m, 3H, Harom.), 8.00-8.05 (m, 2H, Harom.) ppm; 13C NMR (CDCl3): 13.6, 13.8, 19.9, 20.1, 29.9, 30.3, 42.0 (NCH2), 44.5 (NCH2), 112.7 (C3a), 125.7, 126.5, 129.0, 131.3 (Caromat), 149.7, 154.3, 156.4, 156.5 (C2, C4, C6, C7a) ppm; EIMS (m/z, %) 341 (M+ , 100).
Reaction of 5-amino-1,3-dimethylpyrimidine-2,4,6-trione (7) with hydrochloric acid: Typical procedure: Freshly prepared 7 (1,1 g, ca. 6 mmol) was dissolved in a mixture of concentrated hydrochloric acid (10 mL) and water (10 mL) and heated under reflux. After 3 h the reaction was stopped. The colorless solution was cooled to rt, diluted with a concentrated aqueous solution of NaCl (50 mL) and extracted four times, with 50 mL CH2Cl2 each. The organic extracts were dried over MgSO4, filtered and evaporated. The colorless solid residue (300 mg) was investigated by NMR spectroscopy indicating the formation of 35 (88 %) and 36 (12 %). Recrystallisation with CH2Cl2/Et2O (1 : 5) afforded pure 35 ( 220 mg, 32 %).
3-Methyloxazolidine-2,4-dione (35): mp 133 °C; 1H NMR (CDCl3): 3.08 (s, 3H, CH3), 4.68 (s, 2H, CH2), ppm; 13C NMR (CDCl3): 25.9 (NCH3), 68.0 (CH2), 156,0 (C2), 170.4 (C4) ppm; EIMS (m/z, %) 155 (M+, 100).
Reaction of 5,6-diamino-1,3-dimethyl-1H-pyrimidine-2,4,dione (15) with hydrochloric acid: Typical procedure: Freshly prepared 15 (0.5 g, ca. 6 mmol) was dissolved in a mixture of concentrated hydrochloric acid (3 mL) and water (3 mL) and heated under reflux. After 1 h the yellow solution was cooled to rt and extracted three times, with 75 mL CH2Cl2 each. The organic extracts were dried over MgSO4, filtered and evaporated. The product (260 mg) was separated by column chromatography on silica gel (eluent: CH2Cl2 /MeOH 7 : 1). The separated products 13, 16, 17, 42 and 45 were characterized by their physico-chemical properties (TLC, mp, fluorescence) and NMR spectroscopy followed by comparison with literature data as far as accessible. Since part of the individual compounds remained in the aqueous phase yields could not be precisely determined. Due to the extractive method which was selected for separation only qualitative results could reliably be obtained.
1,3,7,9-Tetramethyl-(1H,3H,7H,9H)-pyrimido[5,4-g]pteridine-2,4,6,8-tetrone (16):27 colorless crystals: mp > 360 °C; 1H NMR (CDCl3) 3.52 (s, 6H, NCH3), 3.72 (s, 6H, NCH3) ppm, 13C NMR (CDCl3): 29.1 (NCH3), 29.9 (NCH3), 123.6, 149.4, 150.3, 157.9 ppm.
1,3,6,8-Tetramethyl-(1H,3H,6H,8H)-pyrimido[4,5-g]pteridine-2,4,7,9-tetrone (17):11 blue fluorescent yellow crystals: 1H NMR (CDCl3) 3.55 (s, 6H, NCH3), 3.80 (s, 6H, NCH3) ppm, 13C NMR (CDCl3): 29.4 (NCH3), 30.1 (NCH3), 129.0, 144.3, 150.0, 158.6 ppm.
ACKNOWLEDGMENTS
We gratefully acknowledge support for our studies by the Fonds der Chemischen Industrie and the Bundesministerium für Bildung und Forschung. We thank Dr. Joachim C. Burbiel and Dr. Andreas Brunschweiger for fruitful discussions.
References
1. C. E. Müller, D. Shi, M. Manning Jr., and J. W. Daly, J. Med. Chem., 1993, 36, 3341; CrossRef C. E. Müller, J. Org. Chem., 1994, 59, 1928; CrossRef C. E. Müller, R. Sauer, U. Geis, W. Frobenius, P. Talik, and M. Pawlowski, Arch. Pharm. Pharm. Med. Chem., 1997, 330, 181; CrossRef C. E. Müller, U. Schobert, J. Hipp, U. Geis, W. Frobenius, and M. Pawlowski, Eur. J. Med.Chem., 1997, 32, 709. CrossRef
2. C. E. Müller, Synthesis, 1993, 125. CrossRef
3. C. E. Müller, Tetrahedron Lett., 1991, 32, 6539. CrossRef
4. A. Bayer, Liebigs Ann. Chem., 1863, 127, 1.
5. H. Biltz and M. Heyn, Chem. Ber., 1919, 52, 1298.
6. H. Biltz, K. Strufe, E. Topp, M. Heyn, and R. Robl, Liebigs Ann. Chem., 1921, 423, 200. CrossRef
7. J. Murdoch and O. Doebner, Chem. Ber., 1876, 9, 1102.
8. H. Bredereck, I. Henning, W. Pfleiderer, and O. Deschler, Chem. Ber., 1953, 86, 845. CrossRef
9. F. F. Blicke and H. C. Godt, J. Am. Chem. Soc., 1954, 76, 2798. CrossRef
10. E. C. Taylor, C. K. Cai, and H. M. Loux, J. Am. Chem. Soc., 1954, 76, 1874. CrossRef
11. E. C. Taylor, H. M. Loux, E. A. Falcoand, and G. H. Hitchings, J. Am. Chem. Soc., 1955, 77, 2243. CrossRef
12. W. Techow, Chem. Ber., 1894, 27, 3082.
13. H. Auterhoff and F. J. Bohle, Arch. Pharm., 1968, 301, 73. CrossRef
14. H. Biltz and K. Strufe, Ann. Chem., 1914, 404, 170.
15. C. E. Müller, Habilitationsschrift (habilitation thesis), Tübingen, 1994.
16. O. S. Tee and M. Endo, J. Heterocycl. Chem., 1967, 13, 149. CrossRef
17. S. Cortes and H. Kohn, J. Org. Chem., 1983, 48, 2246. CrossRef
18. J. W. Clark-Levis, Chem. Rev., 1958, 58, 63. CrossRef
19. H. C. Van der Plas, in: Ring Transformations of Heterocycles, Vol. 2, Academic Press, N. Y., 1973, p. 125ff, and references therein.
20. H. Muramatsu, J. Org. Chem., 1990, 55, 1396. CrossRef
21. M. V. Jovanovic and E. R. Biehl, J. Heterocyclic Chem., 1987, 24, 191.
22. H. Biltz, M. Heyn, and T. Hamburger, Chem. Ber., 1916, 49, 662; S. Kato and G. Dryhurst, Journal of Electroanalytical Chemistry and Interfacial Electrochemistry, 1977, 79, 391; CrossRef H. Bredereck, P. Menzel, R. Argosino, and W. Bihlmaier, Makromolekulare Chemie, 1975, 176, 1713. CrossRef
23. E. Fischer and L. Ach, Chem. Ber., 1895, 28, 3135.
24. H. Biltz and T. Hamburger, Chem. Ber., 1916, 49, 635.
25. R. D. Bush, PCT Int. Appl., 1995, , WO 9500112 (no mp or spectroscopic data are given).
26. K. Senga, J. Sato, and S. Nishigaki, Heterocycles, 1977, 6, 689. CrossRef
27. F. F. Blicke, E. C. Taylor, C. K. Cai, and H. M. Loux, J. Am. Chem. Soc., 1955, 76, 1874.