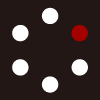
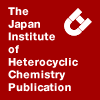
HETEROCYCLES
An International Journal for Reviews and Communications in Heterocyclic ChemistryWeb Edition ISSN: 1881-0942
Published online by The Japan Institute of Heterocyclic Chemistry
e-Journal
Full Text HTML
Received, 29th September, 2008, Accepted, 1st December, 2008, Published online, 3rd December, 2008.
DOI: 10.3987/COM-08-S(D)38
■ Stereocontrolled Total Synthesis of (±)-FR901483
Shigeru Ieda, Yusuke Asoh, Teppei Fujimoto, Haruka Kitaoka, Toshiyuki Kan, and Tohru Fukuyama*
Graduate School of Pharmaceutical Sciences, University of Tokyo, 7-3-1 Hongo, Bunkyo-ku, Tokyo 113-0033, Japan
Abstract
The total synthesis of potent immunosuppressant FR901483 (1) is reported. The remarkable feature of our convergent synthesis is the p-methoxybenzyl and methylamino groups are stereoselectively incorporated within the tri-cyclic core skeleton. The skeleton itself is constructed by an intramolecular aldol reaction on a symmetrical keto-aldehyde (14), which is readily derived by an eight-step sequence from nitromethane and methyl acrylate.INTRODUCTION
FR901483 (1) is a novel immunosuppressant isolated from the fermentation broth of the Cladobotryum species.1 The promising biological activity and intriguing structure of this compound have made it an attractive target for total synthesis.2,3 Although several total syntheses and synthetic studies of 1 have been reported, there are few stereoselective syntheses.4 Herein, we describe the details of our stereocontrolled total synthesis of racemic 1, which may lead to a plethora of diverse analogs.
RESULTS AND DISCUSSION
Scheme 1 illustrates our retrosynthetic analysis. Our synthesis features an intermediacy of tricycle 3 and uses multiple carbonyl groups to append the requisite p-methoxybenzyl and methylamino groups at C(1) and C(10), respectively. The synthesis of 3 would be accomplished using an intramolecular aldol reaction on symmetrical keto-aldehyde 6 as a key step. The spiro-lactam would be derived from triester 9, which would be obtained by a Michael addition with acryrates and nitromethane.
As shown in Scheme 2, cyclization precursor 14 was readily prepared by an eight-step sequence from inexpensive nitromethane 7 and methyl acrylate 8. Upon treatment of 7 and three equivalents of 8 with 5 mol% DBU, the desired Michael reaction proceeded smoothly to afford triester 9. Subsequent reduction of the nitro group and concomitant ester-amide exchange proceeded to provide 10, which possessed the required tetra-substituted carbon. After Dieckmann condensation of diester 10, basic hydrolysis of the corresponding methyl ester and neutralization with acid caused decarboxylation of the β-keto acid to afford spiro-lactam 12 in high yield. Cyclization precursor 14 was prepared from 12 by a four-step sequence involving the protection of the ketone as the dimethyl ketal, allylation of the amide, acidic hydrolysis of the dimethyl ketal, and oxidative cleavage of the double bond. Upon treatment of 14 in the presence of 5 mol% CSA, the crucial intramolecular aldol reaction furnished desired tricycle 5 as a single isomer. The synthesis of 5 from 7 and 8 could be readily scaled up because tedious chromatographic purifications were unnecessary during the synthetic process, which produced 5 as white crystals. A desymmetrizing aldol reaction of 14 could also potentially be used to prepare an optically active compound.5
With desired tri-cyclic compound 5 in hand, we then focused our attention to the ketone of 5. Reduction with NaBH(OAc)36,7 gave the desired α-alcohol. The reaction of ketone 5 underwent hydroxyl group directed reduction to give diol 15. Meanwhile, the reduction of the acetate derivatives of 5 mediated by NaBH4 afforded undesired β-alcohol 16, which is formed when the hydride attacks from the convex face of the molecule (Scheme 3). The stereochemistry of the alcohols in 15 and 16 could not be elucidated by the completion of this total synthesis because the difference of the coupling constants in the 4-position protons is not so significant, which were observed as broad singlets by NMR. Based on the well-known reaction mechanisms, we supposed that 15 have the desired stereochemistry. The two hydroxy groups of 15 were differentiated in a stepwise manner. After protection of 15 as the TBS ether, selective cleavage of the equatorial TBS ether 17 was carried out by treatment with TBAF. Subsequent oxidation of the resultant alcohol under Swern conditions provided key intermediate 19 (Scheme 4).8
The next challenge in the synthesis was stereoselective incorporation of the p-methoxybenzyl groups into tricyclic ketone 19 (Scheme 5). Diastereoselective alkylation proceeded smoothly upon treatment of 19 with LHMDS and p-methoxybenzyl bromide, although the yield was low. Changing from LHMDS to KHMDS increased the yield and provided concomitantly the dialkylated product. As shown in Scheme 5, alkylation was accomplished by treatment with KHMDS in the presence of TMEDA, which presumably plays a key role in the reaction,9 to give desired ketone 20 as a single isomer.
Conversion of ketone 20 into the exo-oriented alcohol was accomplished by a one-electron reduction because reduction with LAH gave exclusively undesired endo-alcohol 22. Because the hydride should attack from the convex face of 20, a one-electron reduction with samarium (II) was investigated. Thus, upon treatment with samarium (II) diiodide (SmI2)10 in the presence of HMPA, ketone 20 was smoothly reduced at –78 °C to provide desired 23. In this SmI2 mediated reaction of 20, the addition of HMPA was essential for high selectivity. A similar reduction of 20 without HMPA did not proceed at –78 °C, and the selectivity resulted in approximately 5:1 to 7:1 ratio. As shown in Scheme 6, chelation between the radical anion and the neighboring carbonyl group with the samarium cation should play a key role in the high selectivity.11 Subsequent protection of 23 with the TBS ether gave 24.
The next challenge was the incorporation of a nitrogen atom into amide 24. Initially, we selected Oppolzer’s protocol, as shown in Scheme 7.12 Although the trapping of chloronitroso compound 25 with the lithium enolate of 24 and subsequent reduction afforded desired amine 28 as a single isomer, the yield was unsatisfactory. However, as shown in Scheme 8, Curtius rearrangement of 29 was investigated because carboxylation of 24 with solid CO2 proceeded smoothly. 29 easily underwent the desired rearrangement upon treatment with DPPA and Et3N, and addition of BnOH to the isocyanate intermediate gave Cbz protected 30 as a 1:1 mixture.
As shown in Scheme 9, an amine group was stereoselectively incorporated using a one-electron reduction as a key step. Upon treatment of 29 with sodium nitrite under acidic conditions a sequential nitrosylation and decarboxylation proceeded smoothly to provide oxime 31. The crucial reduction of 31 was accomplished by treatment with zinc in acetic acid to provide desired amine 28 as a single isomer.
Mono-N-methylation of primary amine 28 was achieved in a stepwise manner. After conversion of 28 into the formamide, treatment with lithium aluminum hydride allowed the simultaneous reduction of both the lactam and the formamide, while concomitant deprotection of one of the TBS groups provided the corresponding methylamine derivative, which was then protected with a Cbz group to afford 32. After deprotection of the TBS ether, regioselective incorporation of the phosphate ester was achieved via the phosphoramidite method13 to give 34.14 Finally, simultaneous cleavage of Cbz and the benzyl ester groups by hydrogenolysis conditions yielded racemic FR901483 (1), which had spectral data (1H NMR, 13C NMR, IR and HRMS) that fully agreed with those of the natural product (Scheme 10).
In conclusion, a highly stereoselective total synthesis of FR901483 (1) was accomplished by alkylation of key intermediate 5, which itself was obtained by an intramolecular aldol reaction. Our synthesis features the stereoselective construction of the exo-oriented alcohol by a SmI2 mediated reduction. Finally, the amine stereochemistry at C(10) is set by a one-electron reduction.
EXPERIMENTAL
General. Nuclear magnetic resonance (1H NMR (400 MHz) and 13C NMR (100 MHz)) spectra were determined on a JEOL-LA400 instrument. Chemical shifts for 1H NMR are reported in parts per million (ppm) downfield from tetramethylsilane (δ) in deuterochloroform as the internal standard, while coupling constants are in hertz (Hz). The following abbreviations are used for spin multiplicity: s = singlet, d = doublet, t = triplet, q = quartet, m = multiplet, and br = broad. Chemical shifts for 13C NMR are reported in ppm relative to the centerline of a triplet at 77.0 ppm for deuterochloroform (CDCl3). Melting points (mp) were determined on a Yanaco Micro Melting Point Apparatus. Infrared spectra (IR), which are reported in wavenumbers (cm–1), were recorded on a JASCO FT/IR-410 Fourier Transform Infrared Spectrometer. Mass spectra (MS) were obtained on a JEOL JMS-GCmate MS-DIP20 with polyethylene glycol as the matrix. Analytical thin layer chromatography (TLC) was performed on Merck precoated analytical plates, 0.25 mm thick, silica gel 60 F254. Preparative TLC separations were made on Merck precoated analytical plates, 0.50 mm thick, silica gel 60 F254. Compounds were eluted from the adsorbent with 10% methanol (MeOH) in chloroform (CHCl3). Flash column chromatography separations were performed on KANTO CHEMICAL Silica Gel 60 (40-100 mesh). All non-aqueous reactions were carried out in oven-dried glass apparatuses under a slight positive pressure of argon. All solvents were dried over molecular sieves 3A or 4A before use. All other reagents were commercially available, and used without further purification, unless otherwise specified.
4-(2-Methoxycarbonyl-ethyl)-4-nitro-heptanedioic acid dimethyl ester (9).
To a stirred solution of MeNO2 7 (20.0 g, 328 mmol) in MeCN (400 mL) at 0 °C were added methyl acrylate 8 (118 mL, 1.32 mol) and DBU (5.40 mL, 36.2 mmol). After stirring at 0 °C for 30 min, saturated aqueous ammonium chloride was added and the mixture was extracted with Et2O. The combined organic layer was washed with brine then dried over MgSO4. The solvent was removed under reduced pressure and the resulting oil 9 (107 g) was used in the next reaction without further purification: 1H-NMR (CDCl3, 400 MHz) δ 2.24-2.35 (12H, m), 3.70 (9H, s); 13C-NMR (CDCl3, 100 MHz) δ 28.4, 30.2, 52.0, 91.8, 172.1; IR (film) 1738, 1538, 1438, 1176 cm¬–1.
3-[2-(2-Methoxycarbonyl-ethyl)-5-oxo-pyrrolidin-2-yl]-propionic acid methyl ester (10).
To a stirred solution of foregoing crude product 9 (50.0 g) in MeOH (250 mL) at rt was added Ra-Ni (W2) (5.00 g). After stirring at 60 °C under hydrogen gas atmosphere (5 kgw/cm2) for 6 h, the mixture was filtered through a pad of Celite and washed with MeOH. The solvent was removed under reduced pressure and the resulting crude product of 10 (40.8 g) was used in the next reaction without further purification: 1H-NMR (CDCl3, 400 MHz) δ 1.82-1.96 (6H, m), 2.30-2.47 (6H, m), 3.69 (6H, s), 6.85 (1H, s); 13C-NMR (CDCl3, 100 MHz) δ 28.7, 30.2, 34.6, 51.9, 60.6, 173.5, 177.3; IR (film) 3213, 3086, 2953, 1731, 1696, 1436 cm¬–1; HRMS (FAB) calcd for C12H20NO5 258.1336 [(M+H)+], found 258.1326.
8-Hydroxy-2-oxo-1-aza-spiro[4.5]dec-7-ene-7-carboxylic acid methyl ester (11).
To a stirred solution of foregoing crude product of 10 (20.0 g) in benzene (150 mL) at 0 °C was added MeOH (315 mL, 7.77 mmol) followed by NaH (7.77 g, 194 mmol). After stirring at 90 °C for 30 min, aqueous 3 N HCl was added and the mixture was extracted with AcOEt. The combined organic layer was washed with saturated aqueous sodium hydrogen bicarbonate and brine then dried over MgSO4. The solvent was removed under reduced pressure. Resulting solid residue was triturated with hexane to afford 11 (12.8 g, 56.8 mmol, 76% for 3 steps) as a white solid: 1H-NMR (CDCl3, 400 MHz) δ 1.71-2.05 (4H, m), 2.35-2.51 (6H, m), 3.76 (3H, s), 6.73 (1H, br), 12.16 (1H, s); 13C-NMR (CDCl3, 100 MHz) δ 26.4, 29.7, 32.2, 32.8, 35.1, 51.6, 57.2, 95.2, 170.7, 172.3, 177.2; IR (film) 3203, 3084, 2951, 1745, 1695, 1620, 1443, 1361 cm¬–1; HRMS (FAB) calcd for C11H16NO4 226.1074 [(M+H)+], found 226.1069.
1-Aza-spiro[4.5]decane-2,8-dione (12).
To a stirred solution of 11 (1.66 g, 7.37 mmol) in MeOH (15 mL) at rt was added aqueous 4 N NaOH
(3.67 mL, 14.7 mmol). The reaction mixture was stirred at 100 °C for 3.5 h. After the reaction mixture was cooled to rt, small amount of methyl orange was added, and was neutralized with aqueous 12 N HCl carefully. The solvent was removed under reduced pressure and the resulting crude product of 12 was used in the next reaction without further purification: 1H-NMR (CDCl3, 400 MHz) δ 1.93-2.10 (6H, m), 2.36-2.61 (6H, m), 8.34 (1H, br); 13C-NMR (CDCl3, 100 MHz) δ 30.0, 32.3, 37.7, 37.8, 58.4, 178.2, 209.6; IR (film) 3202, 1705, 1652, 1323, 1268 cm¬–1; HRMS (FAB) calcd for C9H13NO2 167.0946 (M+), found 167.0962.
1-Allyl-1-aza-spiro[4.5]decane-2,8-dione (13).
To a stirred solution of 12 (7.37 mmol as theoretical amount) in MeOH (15 mL) at rt was added HC(OMe)3 (0.964 mL, 8.81 mmol) followed by CSA (85.3 mg, 0.367 mmol). After stirring at rt for 30 min, the mixture was neutralized with Et3N. The solvent was removed under reduced pressure and the resulting crude product of the dimethyl acetal was used in the next reaction without further purification: 1H-NMR (CDCl3, 400 MHz) δ 1.62-1.73 (8H, m), 1.96 (2H, t, J = 8.2 Hz), 2.40 (2H, t, J = 8.2 Hz), 3.18 (6H, s), 6.36 (1H, br); 13C-NMR (CDCl3, 100 MHz) δ 29.1, 29.7, 34.3, 34.4, 47.7, 58.6, 98.8, 177.1; IR (film) 3207, 2952, 1737, 1692, 1439, 1105, 1056 cm¬–1; HRMS (FAB) calcd for C10H16NO2 182.1181 [(M-OMe)+], found 182.1197.
To a stirred solution of the above dimethyl acetal (7.37 mmol as theoretical amount) in 3:1 THF/DMF (15 mL) at rt was added NaH (861 mg, 21.5 mmol). The mixture was stirred at rt for 10 min then allyl bromide (1.40 mL, 16.2 mmol) was added. After stirring at rt for 3.5 h, saturated aqueous ammonium chloride was added and the mixture was extracted with AcOEt. The combined organic layer was dried over MgSO4. The solvent was removed under reduced pressure and the resulting crude product of the lactam was used in the next reaction without further purification: 1H-NMR (CDCl3, 400 MHz) δ 1.37 (2H, d, J = 13.6 Hz), 1.48 (2H, dt, J = 4.0, 13.6 Hz), 1.84 (2H, dt, J = 4.0, 13.6 Hz), 1.96 (2H, t, J = 4.0 Hz), 2.03 (2H, d, J = 13.6 Hz), 2.40 (2H, t, J = 4.0 Hz), 3.18 (3H, s), 3.21 (3H, s), 3.82 (2H, d, J = 4.8 Hz), 5.10 (1H, d, J = 9.6 Hz), 5.17 (1H, d, J = 15.6 Hz), 5.80 (1H, ddd, J = 4.8, 9.6, 15.6 Hz); 13C-NMR (CDCl3, 100 MHz) δ 28.9, 29.0, 29.1, 31.2, 41.7, 47.6, 47.9, 63.5, 98.5, 116.1, 134.7, 173.6.
To a stirred solution of the above lactam (7.37 mmol as theoretical amount) in 2:1 acetone/H2O (15 mL) at rt was added a catalytic amount of CSA (85.3 mg, 0.368 mmol). The reaction mixture was stirred at 70 °C for 30 min. After the reaction mixture was cooled to rt, Et3N (0.15 mL, 1.08 mmol) was added. The solvent was removed under reduced pressure to afford 13 (1.14 g, 5.50 mmol, 75% for 4 steps) as a white solid: 1H-NMR (CDCl3, 400 MHz) δ 1.80-1.85 (2H, m), 2.10-2.23 (4H, m), 2.40-2.57 (6H, m), 3.85 (2H, d, J = 5.6 Hz), 5.12 (1H, d, J = 10.0 Hz), 5.17 (1H, d, J = 18.4 Hz), 5.79 (1H, ddd, J = 5.6, 10.0, 22.8 Hz); 13C-NMR (CDCl3, 100 MHz) δ 28.8, 28.9, 34.1, 37.6, 41.9, 62.5, 116.6, 134.5, 174.0, 208.6; IR (film) 2938, 1717, 1685, 1404, 1324, 1226, 1151 cm¬–1; HRMS (FAB) calcd for C12H17NO2 207.1259 (M+), found 207.1249.
(2,8-Dioxo-1-aza-spiro[4.5]dec-1-yl)-acetaldehyde (14).
Through a stirred solution of 13 (5.30 g, 25.6 mmol) in 1:1 MeOH/CH2Cl2 (50 mL) at –78 °C was bubbled ozone gas. After the disappearance of starting material monitored by TLC, argon gas was bubbled and to this solution at –78 °C was added Me2S (18.8 mL, 256 mmol). After stirring for 30 min, the solvent was removed under reduced pressure and the resulting crude product of 14 was used in the next reaction without further purification: 1H-NMR (CDCl3, 400 MHz) δ 1.89-1.93 (4H, m), 2.32 (2H, t, J = 8.0 Hz), 2.42-2.62 (6H, m), 3.96 (2H, s), 9.56 (1H, s).
6-Hydroxytetrahydro-1H-7,10a-methanopyrrolo[1,2-a]azocine-3,8(2H,5H)-dione (5).
To a stirred solution of foregoing crude product of 14 (25.6 mmol as theoretical amount) in benzene (50 mL) at rt was added a catalytic amount of CSA (297 mg, 1.28 mmol). The reaction mixture was heated to 90 °C for 5 min. After the reaction mixture was cooled to rt, the mixture was filtered to give a white solid, which was washed with Et2O and dried in vacuo, to yield pure 5 (4.30 g, 20.6 mmol, 80% for 2 steps) as a white solid: 1H-NMR (CDCl3, 400 MHz) δ 1.82-2.25 (6H, m), 2.42-2.71 (4H, m), 2.90-2.96 (2H, m), 4.00 (1H, m), 4.42 (1H, dd, J = 7.3, 13.7 Hz); 13C-NMR (CDCl3, 100 MHz) δ 30.0, 31.7, 35.4, 37.4, 38.9, 43.8, 51.7, 57.2, 66.9, 173.6, 210.7; IR (KBr) 3189, 2915, 1705, 1665, 1641, 1450, 1419, 1218, 1087 cm¬–1; HRMS (FAB) calcd for C11H16NO3 210.1125 [(M+H)+], found 210.1124.
6,8-Dihydroxyoctahydro-3H-7,10a-methanopyrrolo[1,2-a]azocin-3-one (15).
To a stirred mixture of NaBH(OAc)3 (72.0 g, 340 mmol), MeCN (380 mL) and AcOH (77 mL) was added 5 (17.6 g, 84.1 mmol) at 0 °C. After stirring at 0 °C for 2 h, the mixture was warmed to rt where it was stirred for an additional 2 h. The reaction mixture was filtered through a pad of Celite and the solvent was removed under reduced pressure. The resulting residue was purified by silica gel chromatography (CH2Cl2:MeOH=95:5-90:10) to afford crude product of 15, which was used in the next reaction without further purification.
6,8-Bis{[tert-butyl(dimethyl)silyl]oxy}octahydro-3H-7,10a-methanopyrrolo[1,2-a]azocin-3-one (17).
To a stirred solution of foregoing crude product of 15 (84.1 mmol as theoretical amount) in CH2Cl2 (250 mL) at rt was added Et3N (94 mL, 674 mmol) followed by TBSOTf (57.5 mL, 250 mmol). After stirring at rt for 2 h, saturated aqueous ammonium chloride was added and the mixture was extracted with CH2Cl2. The combined organic layer was washed with brine then dried over MgSO4. The solvent was removed under reduced pressure then resulting residue was purified by silica gel chromatography (AcOEt:hexane=0:1-1:1) to afford crude product of 17, which was used in the next reaction without further purification.
8-{[tert-Butyl(dimethyl)silyl]oxy}-6-hydroxyoctahydro-3H-7,10a-methanopyrrolo[1,2-a]azocin-3-
one (18).
To a stirred solution of foregoing crude product of 17 (84.1 mmol as theoretical amount) in THF (450 mL) at rt was added TBAF (1 M in THF) (134 mL, 134 mmol) slowly. After complete addition (2 h), saturated aqueous ammonium chloride was added and the mixture was extracted with AcOEt. The combined organic layer was washed with brine then dried over Na2SO4. The solvent was removed under reduced pressure then resulting residue was purified by silica gel chromatography (AcOEt:hexane=0:1-9:1) to afford 18 (26.6 g, 81.7 mmol, 97% for 3 steps) as a white solid: 1H-NMR (CDCl3 d 7.26, 400 MHz) δ 0.05 (6H, s), 0.88 (9H, s), 1.67-2.00 (6H, m), 2.22-2.36 (3H, m), 2.44-2.52 (1H, m), 2.96 (1H, br), 3.04 (1H, t, J = 11.9 Hz), 4.02 (1H, br), 4.18 (1H, dd, J = 8.2, 13.7 Hz), 4.28 (1H, s); 13C-NMR (CDCl3, 100 MHz) δ -4.9, 18.0, 25.8, 30.1, 30.5, 32.9, 33.0, 34.1, 43.0, 45.0, 58.6, 64.4, 66.7, 173.8; IR (KBr) 3209, 2953, 2928, 1678, 1452, 1252 cm¬–1; HRMS (FAB) calcd for C17H31NO3Si 325.2073 (M+), found 325.2065.
8-{[tert-Butyl(dimethyl)silyl]oxy}tetrahydro-1H-7,10a-methanopyrrolo[1,2-a]azocine-3,6(2H,5H)-
dione (19).
To a solution of oxalyl chloride (0.398 mL, 4.56 mmol) in CH2Cl2 (6 mL) at –78 °C was added DMSO (0.485 mL, 6.83 mmol). After the resulting mixture was stirred for 1 h, 18 (0.741 g, 2.28 mmol) in CH2Cl2 (4 mL) was added and the mixture was stirred for 30 min before Et3N (1.27 mL, 9.11 mmol) was added. The mixture was allowed to warm to rt. After stirring at rt for 30 min, saturated aqueous ammonium chloride was added and the mixture was extracted with AcOEt. The combined organic layer was dried over MgSO4. The solvent was removed under reduced pressure then resulting residue was purified by silica gel chromatography (AcOEt:hexane=1:2-1:1) to afford the pure 19 (620 mg, 1.92 mmol, 84%) as a white solid: 1H-NMR (CDCl3 δ 7.26, 400 MHz) d 0.07 (6H, s), 0.89 (9H, s), 1.48-1.57 (1H, m), 1.70-2.05 (6H, m), 2.34-2.40 (1H, m), 2.48-2.52 (1H, m), 2.57-2.66 (1H, m), 2.72 (1H, s), 3.99 (1H, d, J = 22.0 Hz), 4.00 (1H, d, J = 3.7 Hz), 4.20 (1H, d, J = 22.0 Hz); 13C-NMR (CDCl3, 100 MHz) δ ¬–5.1, 18.0, 25.6, 29.2, 30.1, 31.1, 32.1, 34.2, 52.0, 52.4, 58.6, 67.2, 174.0, 205.7; IR (film) 2933, 2894, 2857, 1711, 1688, 1411, 1255 cm¬–1; HRMS (FAB) calcd for C17H30NO3Si 324.1995 [(M+H)+], found 324.1981.
8-{[tert-Butyl(dimethyl)silyl]oxy}-5-(4-methoxybenzyl)tetrahydro-1H-7,10a-
methanopyrrolo[1,2-a]azocine-3,6(2H,5H)-dione (20).
To a solution of 19 (17.3 g, 53.5 mmol) in THF (540 mL) at –78 °C were added TMEDA (40.3 mL, 267 mmol) and KHMDS (0.5 M in toluene), (110.2 mL, 55.1 mmol). After stirring at –78 °C for 30 min, p-methoxybenzylbromide (30.0 mL, 160 mmol) in THF (200 mL) was added slowly. After complete addition (10 h), the reaction mixture was allowed to warm to rt and then, saturated aqueous ammonium chloride was added. The mixture was extracted with AcOEt. The combined organic layer was washed with brine then dried over Na2SO4. The solvent was removed under reduced pressure then resulting residue was purified by silica gel chromatography (AcOEt:hexane=1:9-2:8) to afford 20 (15.2 g, 34.2 mmol, 64%) as a colorless oil: 1H-NMR (CDCl3 δ 7.26, 400 MHz) d 0.03 (6H, s), 0.84 (9H, s), 1.23-1.34 (2H, m), 1.46-1.59 (3H, m), 1.71-1.89 (2H, m), 2.26 (1H, s), 2.38-2.44 (1H, m), 2.57-2.67 (1H, m), 3.10 (1H, dd, J = 2.7, 13.7 Hz), 3.77 (3H, s), 3.80 (2H, m), 3.92 (1H, m), 4.54 (1H, d, J = 2.7 Hz), 6.78 (2H, d, J = 9.2 Hz), 6.88 (2H, d, J = 8.2 Hz); 13C-NMR (CDCl3, 100 MHz) δ ¬–5.1, 17.9, 25.6, 27.0, 28.9, 30.9, 31.5, 34.1, 35.3, 51.8, 55.2, 59.4, 64.2, 68.0, 113.8, 128.7, 130.8, 158.7, 174.5, 210.0; IR (film) 2935, 2857, 1713, 1689, 1513, 1396, 1252, 1029 cm¬–1; HRMS (FAB) calcd for C25H38NO4Si 444.2570 [(M+H)+], found 444.2565.
8-{[tert-Butyl(dimethyl)silyl]oxy}-6-hydroxy-5-(4-methoxybenzyl)octahydro-3H-7,10a-
methanopyrrolo[1,2-a]azocin-3-one (23).
The solution of SmI2 in THF was freshly prepared by addition of diiodomethane (6.08 mL, 75.7 mmol) to a suspension of Sm (13.6 g, 90.6 mmol) in THF (725 mL) and stirring at rt for 9 h. To the above solution of SmI2 in THF, a degassed mixture of MeOH (6.12 mL, 151 mmol), HMPA (65.8 mL, 378 mmol) and THF (70 mL) was added. To the mixture, 20 (6.63 g, 15.1 mmol) in degassed THF (100 mL) was added dropwise at –78 °C and the reaction was left to stir overnight. Saturated aqueous sodium hydrogen bicarbonate was added at rt and the mixture was extracted with AcOEt. The combined organic layer was washed with brine then dried over MgSO4. The solvent was removed under reduced pressure then resulting residue was purified by silica gel chromatography (AcOEt:hexane=1:9-6:4) to afford 23 containing inseparable HMPA, which was used in the next reaction without further purification.
6,8-Bis{[tert-butyl(dimethyl)silyl]oxy}-5-(4-methoxybenzyl)octahydro-3H-7,10a-
methanopyrrolo[1,2-a]azocin-3-one (24).
To a stirred solution of the foregoing crude 23 (34.9 mmol as theoretical amount) in CH2Cl2 (174 mL) at rt was added 2,6-lutidine (12.2 mL, 105 mmol) followed by TBSOTf (14.9 mL, 69.6 mmol). After stirring at rt for 2.5 h, saturated aqueous ammonium chloride was added and the mixture was extracted with CH2Cl2. The combined organic layer was dried over MgSO4. The solvent was removed under reduced pressure then resulting residue was purified by silica gel chromatography (AcOEt:hexane=5:95-3:7) to afford 24 (16.6 g, 29.7 mmol, 85% for 2 steps) as a pale yellow oil: 1H-NMR (CDCl3 δ 7.26, 400 MHz) δ -0.01-0.02 (12H, m), 0.86 (9H, s), 0.92 (9H, s), 1.55-1.95 (6H, m), 2.03 (1H, s), 2.25-2.43 (2H, m), 3,07-3.13 (1H, m), 3.63 (1H, m), 3.77 (1H, s), 3.78 (3H, s), 3.82-3.87 (1H, m), 4.04-4.09 (1H, m), 6.81 (2H, d, J = 9.2 Hz), 7.21 (2H, d, J = 8.2 Hz); 13C-NMR (CDCl3, 100 MHz) δ ¬–5.0, –4.9, ¬–4.7, –4.1, 18.0, 18.3, 25.7, 25.9, 27.2, 29.5, 31.1, 32.1, 33.7, 34.0, 45.3, 55.2, 58.5, 60.6, 67.5, 69.3, 113.5, 130.2, 132.6, 157.7, 176.1; IR (film) 2953, 2930, 2857, 1693, 1513, 1250, 1077, 1053 cm¬–1; HRMS (FAB) calcd for C31H53NO4Si2 559.3513 (M+), found 559.3528.
6,8-Bis{[tert-butyl(dimethyl)silyl]oxy}-5-(4-methoxybenzyl)-3-oxooctahydro-1H-7,10a-
methanopyrrolo[1,2-a]azocine-2-carboxylic acid (29).
To a solution of diisopropylamine (1.20 mL, 8.56 mmol) in THF (10 mL) was added n-BuLi (1.59 M in hexane)(4.38 mL, 6.96 mmol) at –78 °C, the resulting solution was stirred at 0 °C for 20 min before it was cooled to –78 °C. To the solution was added a solution of 24 (321 mg, 0.573 mmol) in THF (1.5 mL) by dropwise. After stirring for 1 h at –78 °C, crashed dry-ice blocks were added until remaining dry-ice solids were observed. After stirring at –78 °C for 20 min, saturated aqueous ammonium chloride was added and the mixture was extracted with AcOEt (7 times) and CH2CH2 (6 times). The combined organic layer was dried over Na2SO4. The solvent was removed under reduced pressure then resulting residue was washed with hexane and filtered over a pad of Celite. The filtrate was concentrated under reduced pressure to give crude product of 29, which was used in the next reaction without further purification: MS (FAB) m/z 604 (M++1), 582, 482, 450.
6,8-Bis{[tert-butyl(dimethyl)silyl]oxy}-5-(4-methoxybenzyl)hexahydro-1H-7,10a-methanopyrrolo[1,2-a]azocine-2,3-dione 2-oxime (31).
To a stirred solution of 29 in 5:1 Et2O/H2O (2.4 mL) at 0 °C was added NaNO2 (400 mg, 5.8 mmol) followed by conc.HCl (0.25 mL, 3.00 mmol) cautiously in four portions for 3 h. After stirring at rt for 2.5 h, saturated aqueous ammonium chloride was added and stirred for an additional 1 h. The mixture was extracted with AcOEt and CH2CH2 (4 times). The combined organic layer was dried over MgSO4. The solvent was removed under reduced pressure then resulting residue was purified by PTLC (AcOEt:hexane=3:2 x2) to afford 31 (12.6 mg, 21.4 μmol, 57% for 2 steps) as a pale orange solid: 1H-NMR (CDCl3 δ 7.26, 400 MHz) δ 0.01-0.04 (12H, m), 0.86 (9H, s), 0.92 (9H, s), 1.60-2.08 (7H, m), 2.56 (2H, q, J = 18.0 Hz), 3.12 (1H, dd, J = 9.2, 15.6 Hz), 3.65 (1H, s), 3.78 (3H, s), 4.11-4.18 (2H, m), 6.82 (2H, d, J = 8.2 Hz), 7.20 (2H, d, J = 8.2 Hz), 9.42 (1H, br); 13C-NMR (CDCl3, 100 MHz) δ –5.0, –4.9, –4.8, –4.1, 18.0, 18.3, 25.7, 26.0, 28.8, 30.2, 32.7, 33.5, 37.1, 45.2, 55.2, 57.9, 60.1, 66.7, 68.9, 113.7, 130.0, 131.9, 152.8, 157.8, 163.9; IR (film) 3269, 2953, 2930, 2857, 1708, 1660, 1513, 1251 cm¬–1; HRMS (FAB) calcd for C31H52N2O5Si2 588.3415 (M+), found 588.3410.
2-Amino-6,8-bis{[tert-butyl(dimethyl)silyl]oxy}-5-(4-methoxybenzyl)octahydro-3H-7,10a-
methanopyrrolo[1,2-a]azocin-3-one (28).
To a stirred solution of oxime 31 (526 mg, 0.893 mmol) in AcOH (20 mL) at rt was added activated zinc dust (2.30 g, 35.2 mmol) and NH4Cl (ca.1.00 g, 18.7 mmol). After stirring at 50 °C overnight, the mixture was filtered over a pad of Celite and the filtrate was concentrated under reduced pressure. To the resulting residue, was added saturated aqueous sodium hydrogen bicarbonate and the mixture was extracted with CH2CH2. The combined organic layer was dried over Na2SO4. The solvent was removed under reduced pressure then resulting residue was purified by silica gel chromatography (AcOEt:hexane=1:1–MeOH:CH2Cl2=1:9) to afford 28 (406 mg, 0.706 mmol, 79%) as a pale orange solid: 1H-NMR (CDCl3 δ 7.26, 400 MHz) δ –0.02-0.06 (12H, m), 0.85 (9H, s), 0.93 (9H, s), 1.61-2.07 (10H, m), 2.21 (1H, m), 3.13 (1H, dd, J = 7.3, 14.6 Hz), 3.46 (1H, dd, J = 8.7, 10.5 Hz), 3.51 (1H, s), 3.70 (1H, br), 3.79 (3H, s), 3.87 (1H, t, J = 6.9 Hz), 3.98 (2H, dd, J = 6.4, 14.7 Hz), 6.83 (2H, d, J = 8.2 Hz), 7.19 (2H, d, J = 8.2 Hz); 13C-NMR (CDCl3, 100 MHz) δ ¬–5.0, –4.9, –4.8, –4.0, 18.0, 18.1, 25.7, 25.8, 26.4, 30.2, 32.6, 33.5, 42.7, 45.4, 52.6, 55.2, 57.2, 59.3, 67.2, 69.5, 113.7, 129.7, 132.4, 157.8, 176.7; IR (film) 2952, 2930, 2857, 1697, 1513, 1250, 1078, 1050 cm¬–1; HRMS (FAB) calcd for C31H55N2O4Si2 575.3700 [(M+H)+], found 575.3728.
Benzyl 8-{[tert-butyl(dimethyl)silyl]oxy}-6-hydroxy-5-(4-methoxybenzyl)octahydro-1H-7,10a-
methanopyrrolo[1,2-a]azocin-2-yl]methylcarbamate (32).
To a stirred solution of 28 (13.4 mg, 23.3 μmol) in CH2Cl2 (2.0 mL) at 0 °C was added the mixture (0.2 mL) of HCO2H (1.3 mL, 34 mmol) and Ac2O (0.80 mL, 8.5 mmol) dropwise. After stirring at 0 °C for 10 min, the reaction mixture was warmed to rt and was added small amount of toluene. The solvent was removed under reduced pressure to give crude product of the above formamide, this unstable product was used in the next reaction without purification: 1H-NMR (CDCl3 δ 7.26, 400 MHz) δ –0.01-0.06 (12H, m), 0.85 (9H, s), 0.92 (9H, s), 1.57-2.10 (10H, m), 2.61 (1H, dd, J = 8.2, 12.8 Hz), 3.09 (1H, dd, J = 10.1, 17.4 Hz), 3.53 (1H, d, J = 2.7 Hz), 3.70 (1H, br), 3.79 (3H, s), 3.90-3.96 (2H, m), 4.36 (1H, q, J = 6.4 Hz), 6.39 (1H, br), 6.84 (2H, d, J = 8.2 Hz), 7.18 (2H, d, J = 9.2 Hz), 8.19 (1H, s); 13C-NMR (CDCl3, 100 MHz) δ ¬–4.9, –4.9, –4.8, –4.0, 18.0, 18.1, 25.7, 25.8, 25.9, 30.1, 32.2, 33.6, 41.4, 45.2, 50.8, 55.2, 58.6, 59.4, 67.1, 69.5, 113.8, 129.6, 131.9, 157.9, 161.7, 172.2; IR (film) 3286, 2952, 2931, 2857, 1677, 1541, 1250, 1078, 1051 cm¬–1; HRMS (FAB) calcd for C32H55N2O5Si2 603.3644 [(M+H)+], found 603.3618.
To a stirred solution of foregoing crude product of the above formamide (23.0 μmol as theoretical amount) in THF (2.0 mL) at 0 °C was added LAH (28.0 mg, 0.740 mmol). After stirring at 50 °C overnight, water (28 μL), 15% aqueous sodium hydroxide (28 μL), water (84 μL) and sufficient amount of Et2O was added at rt successively. After stirring for 30 min, the mixture was filtered over a pad of Celite and the filtrate was concentrated under reduced pressure to give the crude methylamine, this polar product was used in the next reaction without purification.
To a stirred solution of the foregoing crude methylamine (23.0 μmol as theoretical amount) in 3:1 CH2Cl2/H2O (2.4 mL) at rt was added Na2CO3 (25.0 mg, 236 μmol) followed by CbzCl (15.0 μL, 105 μmol). After stirring at rt for 1 h, saturated aqueous ammonium chloride was added and the mixture was extracted with CH2Cl2. The combined organic layer was dried over Na2SO4. The solvent was removed under reduced pressure then resulting residue was purified by PTLC (AcOEt:hexane=1:1 x2) to afford 32 (11.5 mg, 19.3 μmol, 84% for 3 steps) as a pale yellow oil: 1H-NMR (CDCl3 δ 7.26, 400 MHz) δ –0.03-0.02 (6H, m), 0.85 (9H, s), 1.43-2.02 (8H, m), 2.71-2.75 (1H, m), 2.82 (3H, s), 3.23 (1H, br), 3.45 (2H, br), 3.71 (1H, s), 3.79 (3H, s), 4.82 (1H, br), 5.11 (2H, d, 2.7 Hz), 6.83 (2H, d, J = 9.2 Hz), 7.20 (2H, d, J = 9.2 Hz), 7.31-7.36 (5H, m); 13C-NMR (CD3CN δ 118.2, 100 MHz) δ ¬–4.6, –4.5, 18.6, 23.3, 26.3, 29.3, 31.5, 31.8, 36.3, 44.4, 46.9, 51.3, 52.7, 55.7, 58.6, 59.6, 67.4, 68.6, 114.4, 128.5, 128.7, 129.4, 131.1, 132.7, 138.4, 156.8, 158.8; IR (film) 3448, 2952, 2931, 1698, 1512, 1329, 1249, 1161, 1041 cm¬–1; HRMS (FAB) calcd for C34H51N2O5Si 595.3562 [(M+H)+], found 595.3531.
Benzyl 6,8-dihydroxy-5-(4-methoxybenzyl)octahydro-1H-7,10a-methanopyrrolo[1,2-a]azocin-2-
yl]methylcarbamate (33).
To a stirred solution of 32 (179 mg, 0.301 mmol) in MeCN (9 mL) at 0 °C was added 48% aqueous HF (2.0 mL). After stirring at 0 °C for 5 h, the reaction mixture was diluted with CH2Cl2 and was added saturated aqueous sodium hydrogen bicarbonate. The mixture was extracted with CH2Cl2 (3 times) and CHCl3 (3 times), and the combined organic layer was dried over Na2SO4. The solvent was removed under reduced pressure then resulting residue was purified by silica gel chromatography (AcOEt:hexane=3:1–MeOH:CH2Cl2=1:9) to afford 33 (127 mg, 0.264 μmol, 88%) as a white foam: 1H-NMR (CD3CN δ 1.93, 400 MHz) δ 1.35-2.25 (9H, m), 2.55-2.59 (8H, m), 3.30 (1H, br), 3.49 (2H, br), 3.68 (1H, br), 3.73 (3H, s), 4.69 (1H, br), 5.04 (2H, s), 6.82 (2H, d, J = 8.2 Hz), 7.22 (2H, d, J = 9.2 Hz), 7.35 (5H, s); 13C-NMR (CD3CN δ 118.2, 100 MHz) δ 2.93, 29.7, 35.7, 44.5, 50.2, 51.9, 55.0, 58.3, 66.9, 67.1, 113.7, 127.6, 127.9, 128.3, 130.0, 130.2, 136.4, 156.3, 157.9; IR (film) 3406, 2935, 1684, 1512, 1454, 1334, 1248 cm¬–1; HRMS (FAB) calcd for C28H37N2O5 481.2697 [(M+H)+], found 481.2694.
Benzyl 8-{[bis(benzyloxy)phosphoryl]oxy}-6-hydroxy-5-(4-methoxybenzyl)octahydro-1H-7,10a-
methanopyrrolo[1,2-a]azocin-2-yl]methylcarbamate (34)
To a stirred solution of 33 (125 mg, 0.260 mmol) in CH2Cl2 (20 mL) at 0 °C was added 1-H-tetrazole (182 mg, 2.60 mmol). After stirring for 20 min at 0 °C, dibenzyl(N,N-diisopropyl)phosphoramidite (90%) was added portionwise (slowly) until no starting material was detectable by TLC analysis (ca.147 μL, 0.49 mmol). When the reaction was completed, the reaction mixture was cooled to –78 °C. To the solution was added TBHP (5 M in decane)(0.15 mL) and allowed to stir for 30 min at –78 °C before the addition of saturated aqueous Na2SO3. The mixture was extracted with CH2CH2 and the combined organic layer was dried over Na2SO4. The solvent was removed under reduced pressure then resulting residue was purified by silica gel chromatography (AcOEt:hexane=4:1–MeOH:CH2Cl2=2:8) to afford pure 34 (130 mg, 0.175 mmol, 68%) as a pale yellow oil: 1H-NMR (CD3OD δ 3.31, 400 MHz) δ 1.25-1.55 (2H, m), 1.69 (2H, br), 1.75-2.18 (4H, m), 2.26 (1H, br), 2.72 (3H, s), 2.75-2.97 (3H, m), 3.28 (1H, br), 3.40 (1H, br), 3.66 (1H, br), 3.76 (3H, s), 4.32 (1H, br), 4.71 (1H, br), 4.96-5.05 (4H, m), 5.08 (2H, s), 6.84 (2H, d, J = 9.2 Hz), 7.21 (2H, d, J = 8.2 Hz), 7.34 (10H, s), 7.34 (5H, s); 13C-NMR (CD3OD δ 49.0, 100 MHz) 23.0, 29.2, 29.9, 31.1, 36.2, 44.0, 44.7, 51.8, 53.4, 55.7, 59.5, 67.8, 68.3, 70.8, 77.0, 114.8, 128.8, 129.1, 129.2 129.5, 129.7, 129.7, 131.2, 132.2, 137.2, 138.1, 157.9, 159.6; IR (film) 3408, 2935, 1695, 1512, 1454, 1248, 1009 cm¬–1; HRMS (FAB) calcd for C42H50N2O8P 741.3299 [(M+H)+], found 741.3298.
FR901483 (1)
To a solution of 34 (54.2 mg, 73.1 μmol) in MeOH (5 mL) was added aqueous 1 N HCl (0.1 mL). The solvent was removed under reduced pressure giving HCl salt. Pd/C (10%, dry)(150 mg, 141 μmol) and MeOH (15 mL) were added, and the mixture was stirred at rt under 1 atom of hydrogen gas atmosphere for 5 h and filtered over a pad of Celite, which was washed with MeOH. The combined filtrate was concentrated under reduced pressure and then rinsed with MeCN to give FR901483 (1) (19.7 mg, 46.2 μmol, 63%) as a white solid: 1H-NMR (CD3OD δ 3.31, 400 MHz) δ 1.91 (1H, d, J = 13.7 Hz), 2.02-2.40 (6H, m), 2.45 (1H, br), 2.66 (1H, dd, J = 9.2, 13.7 Hz), 2.78 (3H, s), 3.11 (1H, dd, J = 3.2, 12.4 Hz), 3.64 (1H, br), 3.78 (3H, s), 3.85-3.97 (2H, m), 4.22-4.35 (2H, m), 4.49 (1H, dd, J = 9.6, 13.3 Hz), 6.90 (2H, d, J = 8.2 Hz), 7.33 (2H, d, J = 9.2 Hz); 13C-NMR (CD3OD δ 49.0, 100 MHz) δ 22.4, 27.7, 28.2, 32.4, 34.0, 41.7, 42.8, 51.9, 55.0, 55.7, 62.0, 63.9, 68.8, 71.0, 115.3, 128.6, 131.7, 160.5; IR (film) 3336, 2933, 1612, 1514, 1458, 1248, 1180, 1009 cm¬–1; HRMS (FAB) calcd for C20H32N2O6P 427.1992 [(M+H)+], found 427.1996.
ACKNOWLEDGMENTS
The authors thank Mr. Koji Nagao (Astellas Pharma Inc.) for providing a sample of natural product 1. This work was financially supported by CREST, JST, and a Grant-in-Aid from the Ministry of Education, Culture, Sports, Science and Technology of Japan.
References
1. K. Sakamoto, E. Tsujii, F. Abe, T. Nakanishi, M. Yamashita, N. Shigematsu, S. Izumi, and M. Okuhara, J. Antibiot., 1996, 49, 37.
2. For a review of biomimetic synthesis of this family compounds, see: H. Takayama, J. Synth. Org. Chem. Jpn., 2002, 60, 350.
3. For the synthetic studies, see: (a) T. Fujimoto, H. Kitaoka, S. Ieda, T. Kan, and T. Fukuyama, Abstract of papers, 79th Symposium on Organic Synthesis, Japan, The Society of Synthetic Organic Chemistry, Japan: Tokyo, June 2001; 17, ; H. Suzuki, N. Yamazaki, and C. Kibayashi, Tetrahedron Lett., 2001, 42, 3013; CrossRef K. M. Brummond and J. L. Lu, Org. Lett., 2001, 3, 1347; CrossRef D. J. Wardrop and W. M. Zhang, Org. Lett., 2001, 3, 2001; CrossRef G. Puigbo, F. Diaba, and J. Bonjoch, Tetrahedron, 2003, 59, 2657; CrossRef J. Bonjoch, F. Diaba, G. Puigbo, E. Peidro, and D. Sole, Tetrahedron Lett., 2003, 44, 8387; CrossRef J. E. Kropf, I. C. Meigh, M. W. P. Bebbington, and S. M. Weireb, J. Org. Chem., 2006, 71, 2046; CrossRef S. Kanden and H.-U. Lessig, Org. Lett., 2006, 8, 4763; CrossRef D. B. Gotchev and D. L. Comins, J. Org. Chem., 2006, 71, 9393; CrossRef F. Diaba, E. Ricou, D. Sole, E. Teixido, N, Valls, and J. Bonjoch, ARKIVOC, 2007, 320; A. Asari, P. Angelov, J. M. Auty, and C. J. Hayes, Tetrahedron Lett., 2007, 48, 2631; CrossRef S. T. Simila and S. F. Martin, J. Org. Chem., 2007, 72, 5342; CrossRef H. Seike and E. J. Sorensen, Synlett., 2008, 695.
4. For total syntheses, see: (a) B. B. Snider and H. Lin, J. Am. Chem. Soc., 1999, 121, 7778; CrossRef G. Scheffler, H. Seike, and E. J. Sorensen, Angew. Chem. Int. Ed., 2000, 39, 4593; CrossRef M. Ousmer, N. A. Braun, C. Bavoux, M. Perrin, and M. A. Ciufolini, J. Am. Chem. Soc., 2001, 123, 7543; CrossRef J. Maeng and R. L. Funk, Org. Lett., 2001, 3, 1125; CrossRef T. Kan, T. Fujimoto, S. Ieda, Y Asoh, H. Kitaoka, and T. Fukuyama, Org. Lett., 2004, 6, 2729; CrossRef K. M. Brummond and S. Hong, J. Org. Chem., 2005, 70, 907; CrossRef We are in the process of publishing the formal total synthesis of (-)-FR901483 using Ugi reaction, which was investigated independently of 3m. CrossRef
5. For a review on enantioselective desymmetrization, see: M. C. Willis, J. Chem. Soc., Perkin Trans. 1, 1999, 13, 1765. CrossRef
6. D. A. Evans, K. T. Chapman, and E. M. Carreira, J. Am. Chem. Soc., 1988, 110, 3560. CrossRef
7. For a similar reaction, see ref 4d.
8. A synthetic protocol based on a NaBH(OAc)3 reduction and selective deprotection of the TBS ether has already been reported in ref 4d. However, transformation of 5 to 18 has been developed independently in our laboratory, see ref 3a.
9. A similar enhancement of the mono-alkylation reaction by the addition of alkyl amine derivatives has been reported, see: M. Goto, K. Akimoto, K. Aoki, M. Shindo, and K. Koga, Tetrahedron Lett., 1999, 40, 8129. CrossRef
10. For a recent review of SmI2, see: (a) G. A. Molander and C. R. Harris, Chem. Rev., 1996, 96, 307; CrossRef G. A. Molander, Chem. Rev., 1992, 92, 29. CrossRef
11. The enhancement of the reduction potency of SmI2 by the addition of HMPA has been reported, see: J. Inanaga and M. Yamaguchi, J. Synth. Org. Chem. Jpn., 1989, 47, 200.
12. W. Oppolzer, O. Tamura, and J. Deerberg, Helv. Chim. Acta, 1992, 75, 1965. CrossRef
13. For a review of the phosphoramidite method, see: R. P. Iyer, Tetrahedron, 1992, 48, 2223. CrossRef
14. For a similar regioselective phosphorylation, see ref 4a.