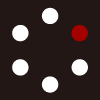
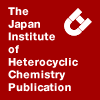
HETEROCYCLES
An International Journal for Reviews and Communications in Heterocyclic ChemistryWeb Edition ISSN: 1881-0942
Published online by The Japan Institute of Heterocyclic Chemistry
e-Journal
Full Text HTML
Received, 29th September, 2008, Accepted, 8th December, 2008, Published online, 10th December, 2008.
DOI: 10.3987/COM-08-S(D)39
■ Intermolecular Carbon Radical Addition to Cyclic Nitrone
Masafumi Ueda, Hideto Miyabe, Nami Nonoguchi, Okiko Miyata, Osamu Tamura, and Takeaki Naito*
Kobe Pharmaceutical University, Motoyamakita, Higashinada, Kobe 658-8558, Japan
Abstract
The intermolecular radical addition to chiral glyoxylic nitrone was studied. The ethyl radical addition to nitrone by using triethylborane proceeded smoothly to give the desired ethylated product with moderate diastereoselectivities accompanying with the ethylated nitrone and the diethylated product. The radical reaction of nitrone took place even in aqueous media. The investigation of optimal reaction conditions and the reaction pathway were described.INTRODUCTION
The carbon-nitrogen double bonds of imine derivatives are of great interest as radical acceptors in synthetic organic chemistry. The reductive radical addition to C=N bonds is important due to the prevalence of organic compounds in nature that contain the amine functional group. Compared with the extensive investigations into radical cyclization of imine derivatives,1,2 the intermolecular radical addition to imines has not been widely studied. Therefore, the studies on intermolecular carbon-carbon bond-forming radical reactions of various imines have been recently demonstrated by several groups.3-10
Nitrone is well-known to be a reactive substrate for 1,3-dipolar cycloaddition reactions, nucleophilic additions of organometallic reagents, and so on.11,12 Although nitrone has also evolved as a useful trap for short-lived reactive free radicals,13 the synthetically useful radical reactions of nitrone are not available until now.14,15 As a part of our program directed toward the screening of reactive imino radical acceptors, we have recently reported the highly diastereoselective radical addition to chiral nitrone 1 for the asymmetric synthesis of α-amino acids 3 (Scheme 1).16 In this paper, we describe full details of radical addition to simple nitrone 4 and the reaction pathway.17
RESULTS AND DISCUSSION
The radical addition reaction of nitrone 4 using triethylborane as an ethyl radical source was studied under the several reaction conditions (Scheme 2, Table 1).18 To a solution of nitrone 4 in CH2Cl2 was added 1.0 M solution of Et3B in hexane (5 equiv), and then the reaction mixture was stirred at 25 oC for 5 min. As expected, the radical reaction proceeded smoothly to give a 78:22 diastereomeric mixture of the desired ethylated product 5 in 46% yield, accompanying with 15% yield of the ethylated nitrone 6 and 9% yield of the diethylated product 7 (Table 1, entry 1). We were able to separate and purify each diastereomeric isomers 3R-5 and 3S-5 by PTLC. Thus, the absolute configuration of the minor product 5 could be determined to be S by X-ray analysis (Figure 1). Changing the temperature from 25 to –78 oC did not show significant effect in the diastereoselectivity (entry 2). Although the replacement of CH2Cl2 with benzene as a solvent did not lead to good diastereoselectivities (entry 3), changing the temperature from 25 oC to reflux in benzene led to an effective increase in the yield of the desired ethylated compound 5. Treatment of 4 with Et3B in boiling benzene for 1 min gave a 71:29 diastereomeric mixture of 5 in 63% yield (entry 4). The ratio of undesired ethylated nitrone 6 to the desired ethylated compound 5 was also dependent on the amount of Et3B. The reaction using only 1 equiv of Et3B in boiling benzene afford a significant amount of 6 (Table 1, entry 5).
As shown in Scheme 3, the ethylated nitrone 6 would be obtained as a result of disproportionation reaction of the intermediate radical B, which was generated by the ethyl radical addition to nitrone 4. These results indicate that Et3B worked as not only a radical initiator but also a radical terminator to trap the intermediate radical B to give an adduct C and a chain-propagating ethyl radical, therefore a large amount of Et3B is required for the selective formation of the desired ethylated product 5.19 However, the reaction of intermediate radical B with Et3B is a slow process; thus, the formation of C- and O-diethylated product 7 may result from the accumulation of the nitroxide radical B in reaction mixture due to the persistent radical effect.
The ratio of the desired ethylated product 5 to the ethylated nitrone 6 was also dependent on the concentration of the substrate 4 (Table 2). Under the high dilution conditions, the yield of the desired product 5 diminished and the yield of the undesired product 6 increased. Good results were obtained by the use of 1 mL of CH2Cl2 or benzene for 0.26 mmol of substrate 4 at 25 °C (entries 1 and 4). Treatment of 4 in CH2Cl2 (1 mL) for 5 min gave a 77:23 diastereomeric mixture of 5 in 66% yield (entry 1), which is better than that obtained by the reaction in CH2Cl2 (10 mL) (entry 3). The reaction of 4 proceeded smoothly in benzene (1 mL) at 25 °C to afford 5 in 71% yield without the formation of the undesired product 6 (entry 4). In contrast, the reaction in benzene (100 mL) gave 31% yield of 5 and 20% yield of 6, accompanying with 27% yield of starting nitrone 4 (entry 6). When the reaction was carried out in refluxing benzene, a similar trend was observed (entries 7-9).
To suppress the formation of the diethylated product 7, several Lewis acids were screened (Table 3). As expected, the undesired product 7 was not formed in the presence of Lewis acid. In the presence of BF3·OEt2, 30% yield of 5 as a 48:52 diastereomeric mixture was obtained without the formation of 7 (entry 1). However, the addition of Lewis acid led to a decease in the ratio of the desired ethylated product 5 to the ethylated nitrone 6. In the case of Bu2BOTf, 33% yield of 5 and 39% yield of 6 were obtained (entry 2). In the case of MAD (Methylaluminum bis(2,6-di-tert-butyl-4-methylphenoxide), 38% yield of 5 and 19% yield of 6 were obtained (entry 3). These results indicate that the intermediate radical B was not effectively trapped by Et3B as a result of the competitive coordination of Lewis acid and Et3B to oxygen atom of B. Thus, the disproportionation reaction of the intermediate radical B was a significant side reaction. Additionally, the addition of Lewis acids influenced the degree of stereoselectivity. Good diastereoselectivities were not observed even at low reaction temperature (entries 4-8).
The stereochemical feature of this reaction can be rationalized in terms of steric control in the conformationally restricted nitrone 4 (Figure 2). In the absence of Lewis acid, the radical addition to the less hindered re (top) face is favored, presumably due to steric interactions with the phenyl group in nitrone D, which prevents addition to si (bottom) face. In the presence of Lewis acid, the steric repulsion between Lewis acids and phenyl group in E led to a decrease in diastereoselectivity.
To suppress the formation of undesired ethylated nitrone 6, we next employed Bu3SnH as a hydride atom donor (Scheme 4). As expected, the yield of 6 diminished as a result of the predominant reaction of intermediate radical B with Bu3SnH to give a 65:35 diastereomeric mixture of the desired ethylated product 5 in 67% yield, accompanying with 16% yield of product 8 as a result of the reduction of nitrone 4 with Bu3SnH.
We next investigated the reaction of nitrone 4 using Et2Zn as an ethyl radical source under the similar reaction conditions (Table 4).20 Et2Zn worked well. Although the use of Et2Zn led to a decrease in diastereoselectivity due to coordination of Et2Zn as a Lewis acid, the formation of the ethylated nitrone 6 was not observed at 25 °C, because the reaction of intermediate radical B with Et2Zn is a quick process (entries 1 and 2). The reaction in CH2Cl2 at 25 °C gave a 54:46 diastereomeric mixture of the desired ethylated product 5 without the formation of undesired products 6 and 7 (entry 1). The formation of undesired products 6 was observed by performed the reaction at -78 °C (entry 3).
The use of water as a solvent has generated considerable interest from both economical and environmental points of view.21 Particularly, the carbon-carbon bond formation in aqueous media is a challenging problem.22,23 We finally investigated the reaction of 4 in aqueous media (Scheme 5). To a solution of 4 in H2O/MeOH was added a solution of Et3B in MeOH (5 equiv), and then the reaction mixture was stirred at 25 oC for 10 min. The radical reaction smoothly proceeded to give a 75:25 diastereomeric mixture of the desired ethylated product 5 in 51% yield, accompanying with 6% yield of the diethylated product 7.
In conclusion, we have demonstrated that nitrone is an excellent radical acceptor for intermolecular carbon-carbon bond-forming radical reactions even in the presence of water. In addition to intermolecular radical reaction of oxime ethers, hydrazones, and N-sulfonylimines, the radical reactions of nitrones disclosed a broader aspect of the utility of imine derivatives as a radical acceptor for the synthesis of various types of amino compounds.
EXPERIMENTAL
General.
Melting points are uncorrected. 1H and 13C NMR spectra were recorded at 200, 300 or 500 MHz and at 50, 75 or 125 MHz, respectively. IR spectra were recorded using FTIR apparatus. Mass spectra were obtained by EI, CI, or SIMS methods. Preparative TLC separations were carried out on precoated silica gel plates (E. Merck 60F254). Flash column chromatography was performed using E. Merck Kieselgel 60 (230-400 mesh).
Et3B-mediated ethyl radical addition to nitrone 4 (Table 1 and 2).
To a solution of nitrone 4 (50 mg, 0.26 mmol) in CH2Cl2 or benzene (1, 5, 10, or 100 mL) was added Et3B (1.0 M in hexane, 1.31 mL, 1.31 mmol or 0.26 mL, 0.26 mmol) at –78 °C, +25 °C or reflux. After being stirred at the same temperature for 1, 5 or 60 min, the reaction mixture was concentrated at reduced pressure. Purification of the residue by preparative TLC (hexane/AcOEt 3:1, 2-fold developments) afforded the desired ethylated product R-5, S-5, the ethylated nitrone 6 and diethylated product 7, respectively.
(3R,5R)-3-Ethyl-4-hydroxy-5-phenyl-2-morpholinone (R-5) colorless crystals: mp 73-77 °C (benzene). IR (CHCl3) 3577, 2966, 1743 cm-1. 1H NMR δ 7.42-7.32 (5H, m), 5.51-5.46 (1H, m), 4.92-4.84 (1H, m), 4.65 (1H, dd, J=11.5, 5.0 Hz), 4.42 (1H, dd, J = 9.5, 5.0 Hz), 3.69-3.63 (1H, m), 2.15-1.85 (2H, m), 1.11 (3H, t, J = 7.5 Hz). 13C NMR (CDCl3) δ 170.6, 135.9, 128.9, 128.5, 127.7, 67.1, 66.9, 60.2, 23.3, 11.2. HRMS: Calcd for C12H15NO3 (M+): 221.1051, Found: 221.1071. Anal. Calcd for C12H15NO3: C, 65.14; H, 6.83; N, 6.33. Found: C, 65.10; H, 6.86; N, 6.08. [α]17D +33.0 (c 1.01, CHCl3).
(3S,5R)-3-Ethyl-4-hydroxy-5-phenyl-2-morpholinone (S-5) colorless crystals: mp 122-127 °C (benzene/hexane). IR (CHCl3) 3574, 3381, 3019, 2975, 1741 cm-1. 1H NMR δ 7.44-7.34 (5H, m), 5.11 (1H, br s), 4.25-4.18 (2H, m), 4.05 (1H, t, J = 7.5 Hz), 3.72 (1H, t, J = 4.3 Hz), 2.18-2.02 (2H, m), 1.07 (3H, t, J = 7.5 Hz). 13C NMR (CDCl3) δ 169.7, 136.1, 129.1, 128.8, 127.8, 70.0, 69.5, 67.1, 22.9, 9.3. HRMS: Calcd for C12H15NO3 (M+): 221.1051, Found: 221.1069. Anal. Calcd for C12H15NO3: C, 65.14; H, 6.83; N, 6.33. Found: C, 64.84; H, 6.75; N, 6.33. [α]18D -58.1 (c 1.21, CHCl3). Crystal data of S-5: C12H15NO3, space group MONOCLINIC, p21 with a = 9.415 (2), b = 6.8150 (9), c = 9.817 (1) Å, V = 605.3 (1) Å3, final R value 0.0312 for 1194 reflections.
(5R)-3-Ethyl-5, 6-dihydro-5-phenyl-2H-1,4-oxadin-2-one 4-oxide (6)
a colorless oil: IR (CHCl3) 3013, 1721, 1549 cm-1. 1H NMR δ 7.44-7.31 (5H, m), 5.11 (1H, t, J = 4.0 Hz), 4.82 (1H, dd, J = 12.5, 4.0 Hz), 4.68 (1H, dd, J = 12.5, 4.0 Hz), 2.87-2.75 (2H, m), 1.18 (3H, t, J = 7.5 Hz). 13C NMR (CDCl3) δ 158.8, 140.9, 132.3, 129.7, 129.3, 127.0, 70.8, 66.9, 19.3, 9.2. HRMS: Calcd for C12H13NO3 (M+): 219.0895, Found: 219.0909.
(5R)-4-Ethoxy-3-ethyl-5-phenyl-2-morpholinone (7)
a colorless oil (5:1mixture of diasteromers): IR (CHCl3) 2979, 1736, 1455 cm-1. 1H NMR δ 7.46-7.32 (5H, m), 4.66-4.58 (1H, m), 4.44-4.34 (1H, m), 4.30-4.21 (1H, m), 3.66-3.56 (3H, m), 2.15-1.82 (2H, m), 1.15 (15/6H, t, J = 7.5 Hz), 1.09 (3/6H, t, J = 7.5 Hz) 1.01 (15/6H, br t, J = 7.5 Hz), 0.98 (3/6H, t, J = 7.5 Hz). 13C NMR (CDCl3) δ 173.1, 170.9, 138.3, 128.8, 128.7, 128.62, 128.58, 128.5, 128.2, 127.8, 127.7, 127.0, 75.0, 68.5, 67.4, 64.2, 53.5, 33.0, 32.2, 13.9, 11.3, 8.8, 8.0. HRMS: Calcd for C14H19NO3 (M+): 249.1364, Found: 249.1376.
Et3B-mediated ethyl radical addition to nitrone 4 in the presence of Lewis acid (Table 3).
To a solution of nitrone 4 (50 mg, 0.26 mmol) and Lewis acid (0.26 mmol) in CH2Cl2 (10 mL) was added Et3B (1.0 M in hexane, 1.31 mL, 1.31 mmol) at –78 °C or +25 °C. After being stirred at the same temperature for 15-60 min, the reaction mixture was diluted with saturated aqueous NaHCO3 and then extracted with CH2Cl2. The organic phase was dried over MgSO4 and concentrated at reduced pressure. Purification of the residue by preparative TLC (hexane/AcOEt 3:1, 2-fold developments) afforded the desired ethylated product R-5, S-5 and the ethylated nitrone 6, respectively.
Et3B-mediated ethyl radical addition to nitrone 4 in the presence of Bu3SnH.
To a solution of nitrone 4 (50 mg, 0.26 mmol) in CH2Cl2 (10 mL) were added Et3B (1.0 M in hexane, 1.31 mL, 1.31 mmol) and Bu3SnH (0.085 mL, 0.31 mmol) at 25 °C. After being stirred at the same temperature for 5 min, the reaction mixture was concentrated at reduced pressure. Purification of the residue by preparative TLC (hexane/AcOEt 3:1, 2-fold developments) afforded the ethylated product 5 (39.0 mg, 67%, diastereomeric ratio 65:35), diethylated product 7 (18.1 mg, 28%) and reduction product 8 (8.1 mg, 16%).
(5R)-4-Hydroxy-5-phenyl-2-morpholinone (8)
colorless crystals: mp 95-97 °C (hexane/AcOEt). IR (CHCl3) 3572, 1748 cm-1. 1H NMR δ 7.44 -7.34 (5H, m), 5.52 (1H, br s), 4.43-4.26 (2H, m), 4.13, 3.66 (each 1H, d, J = 17 Hz), 3.96 (1H, t, J = 8 Hz). 13C NMR (CDCl3) δ 167.1, 135.0, 128.9, 128.8, 127.8, 69.6, 66.6, 58.9. HRMS: Calcd for C10H11NO3 (M+) 193.0738, Found: 193.0742. [α]21D -124.2 (c 1.11, CHCl3).
Et2Zn-mediated ethyl radical addition to nitrone 4.
To a solution of nitrone 4 (50 mg, 0.26 mmol) in CH2Cl2 or benzene (10 mL) was added Et2Zn (1.0 M in
hexane, 1.31 mL, 1.31 mmol) at –78 °C or +25 °C. After being stirred at the same temperature for 10 min, the reaction mixture was diluted with saturated aqueous NH4Cl and then extracted with CH2Cl2. The organic phase was dried over MgSO4 and concentrated at reduced pressure. Purification of the residue by preparative TLC (hexane/AcOEt 3:1, 2-fold developments) afforded the desired ethylated product R-5, S-5, the ethylated nitrone 6 and diethylated product 7, respectively.
Et3B-mediated ethyl radical addition to nitrone 4 in aqueous media.
To a solution of nitrone 4 (50 mg, 0.26 mmol) in H2O-MeOH (1:2, 12 mL) was added Et3B (1.0 M in hexane, 1.31 mL, 1.31 mmol) at 25 °C. After being stirred at the same temperature for 10 min, the reaction mixture was diluted with saturated aqueous NaHCO3 and then extracted with CH2Cl2. The organic phase was dried over MgSO4 and concentrated at reduced pressure. Purification of the residue by preparative TLC (hexane/AcOEt 3:1, 2-fold developments) afforded the desired ethylated product 5 (29.6 mg, 51%, diastereomeric ratio 70:30) and the diethylated product 7 (3.6 mg, 6%), respectively.
ACKNOWLEDGEMENTS
We wish to thank Grant-in Aid for Scientific Research (B) (T.N.), for Scientific Research (C) (H.M. and O.M.) and for Young Scientists (B) (M.U.) from the Ministry of Education, Culture, Sports, Science and Technology of Japan, and the Science Research Promotion Fund of the Japan Private School Promotion Foundation for research grants. We also thank Dr. H. Hiramatsu and Dr. K. Aoe, Tanabe Seiyaku, Co. Ltd, for X-ray analysis.
References
1. For reviews, see: H. Miyabe, O. Miyata, and T. Naito, J. Synth. Org. Chem., Jpn., 2002, 60, 1087; H. Ishibashi, T. Sato, and M. Ikeda, Synthesis, 2002, 695; CrossRef G. K. Friestad, Tetrahedron, 2001, 57, 5461; CrossRef T. Naito, Heterocycles, 1999, 50, 505; CrossRef A. G. Fallis and I. M. Brinza, Tetrahedron, 1997, 53, 17543; CrossRef B. Giese, B. Kopping, T. Göbel, J. Dickhaut, G. Thoma, K. J. Kulicke, and F. Trach, Org. React., (N.Y.), 1996, 48, 301; ‘Radicals in Organic Synthesis’, Vol. 1 and 2, ed. by P. Renaud and M. P. Sibi, Wiley-VCH, Weinheim, 2001.
2. For recent examples of the radical cyclization of imine derivatives, see: A. Shirai, O. Miyata, N. Tohnai, M. Miyata, D. J. Procter, D. Sucunza, and T. Naito, J. Org. Chem., 2008, 73, 4464; CrossRef F. Portela-Cubillo, J. S. Scott, and J. C. Walton, Chem. Commun., 2008, 2935; CrossRef G. K. Friestad and A. K. Mathies, Tetrahedron, 2007, 63, 9373; CrossRef G. K. Friestad, T. Jiang, and A. K. Mathies, Tetrahedron, 2007, 63, 3964; CrossRef M. Kitamura and K. Narasaka, Bull. Chem. Soc. Jpn., 2008, 81, 539; CrossRef O. Miyata, A. Shirai, S. Yoshino, T. Nakabayashi, Y. Takeda, T. Kiguchi, D. Fukumoto, M. Ueda, and T. Naito, Tetrahedron, 2007, 63, 10092; CrossRef M. Tojino, N. Otsuka, T. Fukuyama, H. Matsubara, and I. Ryu, J. Am. Chem. Soc., 2006, 128, 7712; CrossRef O. Miyata, A. Shirai, S. Yoshino, Y. Takeda, M. Sugiura, and T. Naito, Synlett, 2006, 893.
3. For reviews, see. G. K. Friestad and A. K. Mathies, Tetrahedron, 2007, 63, 2541; CrossRef H. Miyabe, M. Ueda, and T. Naito, Synlett, 2004, 1140; K. Yamada, Y. Yamamoto, and K. Tomioka, J. Synth. Org. Chem. Jpn., 2004, 62, 1158.
4. D. J. Hart and F. L. Seely, J. Am. Chem. Soc., 1988, 110, 1633. CrossRef
5. S. Kim, I. Y. Lee, J.-Y. Yoon, and D. H. Oh, J. Am. Chem. Soc., 1996, 118, 5138; CrossRef S. Kim and J.-Y. Yoon, J. Am. Chem. Soc., 1997, 119, 5982; CrossRef I. Ryu, H. Kuriyama, S. Minakata, M. Komatsu, J.-Y. Yoon, and S. Kim, J. Am. Chem. Soc., 1999, 121, 12190. CrossRef
6. M. P. Bertrand, L. Feray, R. Nouguier, and L. Stella, Synlett, 1998, 780; CrossRef M. P. Bertrand, L. Feray, R. Nouguier, and P. Perfetti, Synlett, 1999, 1148; CrossRef M. P. Bertrand, L. Feray, R. Nouguier, and P. Perfetti, J. Org. Chem., 1999, 64, 9189. CrossRef
7. G. K. Friestad and A. Ji, Org. Lett., 2008, 10, 2311; CrossRef G. K. Friestad, C. Draghici, M. Soukri, and J. Qin, J. Org. Chem., 2005, 70, 6330; CrossRef G. K. Friestad and J. Qin, J. Am. Chem. Soc., 2000, 122, 8329; CrossRef G. K. Friestad and J. Qin, J. Am. Chem. Soc., 2001, 123, 9922; CrossRef G. K. Friestad, Y. Shen, and E. L. Ruggles, Angew. Chem. Int. Ed., 2003, 42, 5061. CrossRef
8. T. Akindele, Y. Yamamoto, M. Maekawa, H. Umeki, K. Yamada, and K. Tomioka, Org. Lett., 2006, 8, 5729; CrossRef K. Yamada, H. Fujihara, Y. Yamamoto, Y. Miwa, T. Taga, and K. Tomioka, Org. Lett., 2002, 4, 3509; CrossRef K. Yamada, Y. Yamamoto, and K. Tomioka, Org. Lett., 2003, 5, 1797; CrossRef K. Yamada, Y. Yamamoto, M. Maekawa, and K. Tomioka, J. Org. Chem., 2004, 69, 1531; CrossRef K. Yamada, Y. Yamamoto, M. Maekawa, J. Chen, and K. Tomioka, Tetrahedron Lett., 2004, 45, 6595. CrossRef
9. M. Ueda, A. Ono, D. Nakao, O. Miyata, and T. Naito, Tetrahedron Lett., 2007, 48, 841; CrossRef H. Miyabe, A. Nishimura, Y. Fujishima, and T. Naito, Tetrahedron, 2003, 59, 1901; CrossRef H. Miyabe, C. Konishi, and T. Naito, Org. Lett., 2000, 2, 1443; CrossRef H. Miyabe, C. Ushiro, M. Ueda, K. Yamakawa, and T. Naito, J. Org. Chem., 2000, 65, 176; CrossRef H. Miyabe, M. Ueda, N. Yoshioka, and T. Naito, Synlett, 1999, 465; CrossRef H. Miyabe, N. Yoshioka, M. Ueda, and T. Naito, J. Chem. Soc., Perkin Trans. 1, 1998, 3659; CrossRef H. Miyabe, Y. Fujishima, and T. Naito, J. Org. Chem., 1999, 64, 2174; CrossRef H. Miyabe, C. Ushiro, and T. Naito, Chem. Commun., 1997, 1789.
10. E. Risberg, A. Fischer, and P. Somfai, Chem. Commun., 2004, 2088; CrossRef N. Singh, R. D. Anand, and S. Trehan, Tetrahedron Lett., 2004, 45, 2911; CrossRef M. Fernández and R. Alonso, Org. Lett., 2003, 5, 2461; CrossRef S. Torrente and R. Alonso, Org. Lett., 2001, 3, 1985; CrossRef G. A. Russell, L. Wang, and R. Rajaratnam, J. Org. Chem., 1996, 61, 8988. CrossRef
11. For reviews on 1,3-dipolar cycloaddition of nitrones, see: J. Revuelta, S. Cicchi, A. Goti, and A. Brandi, Synthesis, 2007, 485; R. Pal, S. C. Ghosh, K. Chandra, and A. Basak, Synlett, 2007, 2321.
12. For a review on nucleophilic addition of nitrones, see: P. Merino, Comptes Rendus Chimie, 2005, 8, 775; CrossRef M. Lombardo and C. Trombini, Synthesis, 2000, 759; CrossRef P. Merino, S. Franco, F. L. Merchan, and T. Tejero, Synthesis, 2000, 442.
13. For some examples, see: D. A. Becker, J. Am. Chem. Soc., 1996, 118, 905; CrossRef Y.-T. Park and K.-W. Kim, J. Org. Chem., 1998, 63, 4494. CrossRef
14. Silylnitronates and nitroalkyl anions have been used as radical acceptors. See: B. P. Branchaud and G.-X. Yu, Tetrahedron Lett., 1991, 32, 3639; CrossRef S. Kim, J.-Y. Yoon, and C. J. Lim, Synlett, 2000, 1151.
15. For recent reports on SmI2-induced radical coupling reaction of nitrones. See: M. Chavarot, M. Rivard, F. Rose-Munch, E. Rose, and S. Py, Chem. Commun., 2004, 2330; CrossRef Y.-W. Zhong, M.-H. Xu, and G.-Q. Lin, Org. Lett., 2004, 6, 3953; CrossRef D. Riber and T. Skrydstrup, Org. Lett., 2003, 5, 229; CrossRef G. Masson, P. Cividino, S. Py, and Y. Vallée, Angew. Chem. Int. Ed., 2003, 42, 2265; CrossRef G. Masson, S. Py, and Y. Vallée, Angew. Chem. Int. Ed., 2002, 41, 1772. CrossRef
16. We recently reported that a high degree of stereocontrol in radical addition to bulky nitrone 1 was achieved. See: M. Ueda, H. Miyabe, M. Teramachi, O. Miyata, and T. Naito, Chem. Commun., 2003, 426; CrossRef M. Ueda, H. Miyabe, M. Teramachi, O. Miyata, and T. Naito, J. Org. Chem., 2005, 70, 6653. CrossRef
17. For preparation and diastereoselective 1,3-dipolar cycloaddition reactions of cyclic nitrone 4 and its application, see. T. Ueda, M. Inada, I. Okamoto, N. Morita, and O. Tamura, Org. Lett., 2008, 10, 2043; CrossRef O. Tamura, N. Mita, Y. Imai, T. Nishimura, T. Kiyotani, M. Yamasaki, M. Shiro, N. Morita, I. Okamoto, T. Takeya, H. Ishibashi, and M. Sakamoto, Tetrahedron, 2006, 62, 12227; CrossRef O. Tamura, T. Shiro, M. Ogasawara, A. Toyao, and H. Ishibashi, J. Org. Chem., 2005, 70, 4569; CrossRef O. Tamura, T. Shiro, A. Toyao, and H. Ishibashi, Chem. Commun., 2003, 2678; CrossRef O. Tamura, K. Gotanda, J. Yoshino Y. Morita, R. Terashima, M. Kikuchi, T. Miyawaki, N. Mita, M. Yamashita, H. Ishibashi, and M. Sakamoto, J. Org. Chem., 2000, 65, 8544; CrossRef O. Tamura, S. Yoshida, H. Sugita, N. Mita, Y. Uyama, N. Morita, M. Ishiguro, T. Kawasaki, H. Ishibashi, and M. Sakamoto, Synlett, 2000, 1553; O. Tamura, T. Kuroki, Y. Sakai, J. Takizawa, J. Yoshino, Y. Morita, N. Mita, K. Gotanda, and M. Sakamoto, Tetrahedron Lett., 1999, 40, 895; CrossRef O. Tamura, K. Gotanda, R. Terashima, M. Kikuchi, T. Miyawaki, and M. Sakamoto, Chem. Commun., 1996, 1861; T. B. Nguyen, A. Martel, R. Dhal, and G. Dujardin, J. Org. Chem., 2008, 73, 2621; CrossRef S. W. Baldwin, B. G. Young, and A. T. McPhail, Tetrahedron Lett., 1998, 39, 6819; CrossRef A. Long and S. W. Baldwin, Tetrahedron Lett., 2001, 42, 5343. CrossRef
18. For an example of the reaction of nitrones with Et3B, see: W. G. Hollis, Jr., P. L. Smith, D. K. Hood, and S. M. Cook, J. Org. Chem., 1994, 59, 3485. CrossRef
19. Renaud reported that TEMPO reacts with B-alkylcatecholboranes to generate alkyl radicals. See: C. Ollivier, R. Chuard, and P. Renaud, Synlett, 1999, 807. CrossRef
20. Recently, Ryu and Komatsu reported that diethylzinc-air system can serve as an initiator of tin hydride-mediated radical reaction. See: I. Ryu, F. Araki, S. Minakata, and M. Komatsu, Tetrahedron Lett., 1998, 39, 6335. CrossRef
21. P. P. Garner, D. T. Parker, J. J. Gajewski, A. Lubineau, J. Angé, Y. Queneau, I. P. Beletskaya, A. V. Cheprakov, F. Fringuelli, O. Piermatti, F. Pizzo, and S. Kobayashi, ‘Organic Synthesis in Water’, ed. by P. A. Grieco, Blackie Academic & Professional, London, 1998, ; For reviews, see: C. J. Li, Chem. Rev., 1993, 93, 2023; CrossRef A. Lubineau, J. Angé, and Y. Queneau, Synthesis, 1994, 741; CrossRef C. J. Li, Tetrahedron, 1996, 52, 5643. CrossRef
22. Oshima’s group reported that triethylborane can be applied to the radical reactions in aqueous media, see: O. Yamazaki, H. Togo, G. Nogami, and M. Yokoyama, Bull. Chem. Soc. Jpn., 1997, 70, 2519; CrossRef H. Yorimitsu, T. Nakamura, H. Shinokubo, and K. Oshima, J. Org. Chem., 1998, 63, 8604; CrossRef T. Nakamura, H. Yorimitsu, H. Shinokubo, and K. Oshima, Synlett, 1998, 1351. CrossRef
23. M. Ueda, H. Miyabe, H. Sugino, and T. Naito, Org. Biomol. Chem., 2005, 3, 1124; CrossRef H. Miyabe and T. Naito, Org. Biomol. Chem., 2004, 2, 1267; CrossRef S. McNabb, M. Ueda, and T. Naito, Org. Lett., 2004, 6, 1911; CrossRef H. Miyabe, M. Ueda, A. Nishimura, and T. Naito, Tetrahedron, 2004, 60, 4227; CrossRef M. Ueda, H. Miyabe, A. Nishimura, O. Miyata, Y. Takemoto, and T. Naito, Org. Lett., 2003, 5, 3835; CrossRef M. Ueda, H. Miyabe, A. Nishimura, H. Sugino, and T. Naito, Tetrahedron: Asymmetry, 2003, 14, 2857; CrossRef H. Miyabe, A. Nishimura, M. Ueda, and T. Naito, Chem. Commun., 2002, 1454; CrossRef H. Miyabe, M. Ueda, A. Nishimura, and T. Naito, Org. Lett., 2002, 4, 131; CrossRef H. Miyabe, M. Ueda, and T. Naito, Chem. Commun., 2000, 2059; CrossRef H. Miyabe, M. Ueda, and T. Naito, J. Org. Chem., 2000, 65, 5043. CrossRef