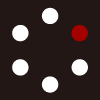
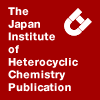
HETEROCYCLES
An International Journal for Reviews and Communications in Heterocyclic ChemistryWeb Edition ISSN: 1881-0942
Published online by The Japan Institute of Heterocyclic Chemistry
e-Journal
Full Text HTML
Received, 29th September, 2008, Accepted, 16th January, 2009, Published online, 16th January, 2009.
DOI: 10.3987/COM-08-S(D)42
■ Synthetic Studies toward (+)-Lysergic Acid: Construction of the Tetracyclic Ergoline Skeleton
Tohru Inoue, Satoshi Yokoshima, and Tohru Fukuyama*
Graduate School of Pharmaceutical Sciences, University of Tokyo, 7-3-1 Hongo, Bunkyo-ku, Tokyo 113-0033, Japan
Abstract
We have successfully constructed the tetracyclic skeleton of lysergic acid, which features an acylation at the C3 position of the indole as well as an intramolecular Heck reaction. The optically active D ring unit could be derived from L-pyroglutamic acid via a disrotatory electrocyclic reaction of a dibromocyclopropane.Ergot alkaloids are biosynthesized by coupling of a variety of amine moieties with a common carboxylic acid, lysergic acid (1).1 Lysergic acid has attracted a lot of attention because of its unique tetracyclic structure as well as the interesting biological activities of ergot alkaloids. While numerous synthetic studies and total syntheses of racemic lysergic acid have been reported to date,2 the first total synthesis of (+)-lysergic acid was reported in 2004 by Szántay and coworkers, which involves resolution of a racemic tetracyclic intermediate using (–)-dibenzoyl-L-tartaric acid.3 We have recently achieved the total synthesis of (+)-lysergic acid methyl ester, featuring an enzymatic desymmetrization of a 1,3-diol, an addition of 2,6-dibromophenyllithium to a nitroalkene, and most notably a palladium-mediated double cyclization.4 While we were successful in the asymmetric synthesis of (+)-lysergic acid methyl ester, we were not particularly satisfied with the long synthetic route consisting of more than 30 steps. Herein, we report our more recent studies toward the total synthesis of (+)-lysergic acid.
RESULTS AND DISCUSSION
As shown in the retrosynthesis outlined in Scheme 1, the C ring of 1 could be constructed by means of an intramolecular Heck reaction. For smooth β-H elimination, the D ring should be prepared as a trans-2,5-disubstituted 1,2,5,6-tetrahydropyridine. Disconnection of 3 at the 3-position of the indole could lead to indole unit 4 and D-ring unit 5. We envisaged that the tetrahydropyridine moiety of D-ring unit 5 could be derived from L-pyroglutamic acid (7) using an electrocyclic reaction of dibromocyclopropane 6.5
Our synthesis commenced with the acylation of the known lactam 8, which was derived from L-glutamic acid (Scheme 2).6 Thus, successive treatment of 8 with LHMDS and CbzCl gave 9 in good yield. Reduction of 9 with DIBAL and subsequent dehydration of the resulting hemiaminal under slightly acidic conditions afforded enamide 10. Dibromocyclopropanation of the enamide proceeded smoothly under
biphasic conditions in the presence of a phase-transfer catalyst to furnish dibromocyclopropane 11, which, upon heating in refluxing xylene, underwent a disrotatory electrocyclic reaction to give directly the optically active dihydropyridine 13 as a result of the spontaneous deprotonation of the cation 12.
Dihydropyridine 13 was transformed into D-ring unit 18 by way of a seven-step sequence (Scheme 3). Thus, reduction of 13 with triethylsilane under acidic conditions occurred regioselectively to give 14, which was then subjected to carbonylation with a palladium catalyst to afford ester 15. Removal of the Cbz group in the presence of a double bond was achieved using Ritter’s method,7 and subsequent nosylation of the resulting secondary amine furnished 16. Acidic cleavage of the pivalate ester followed by Jones oxidation gave carboxylic acid 17,8 which was converted into acid chloride 18 according to Ghosez’s procedure.9
The crucial coupling between the D ring and the indole units was effected by acylation of the magnesium salt of 4-bromoindole (Scheme 4).10 Thus, deprotonation of 4-bromoindole with ethylmagnesium bromide and subsequent addition of acid chloride 18 provided coupling product 20 in moderate yield. We next focused on reduction of the carbonyl functionality. Since a direct reduction of the ketone in 20 could not be achieved due to the electron-donating nature of the indole nitrogen, an Alloc group was introduced at the indole nitrogen to activate the carbonyl group. As expected, reduction of the N-Alloc compound proceeded smoothly to furnish a diastereomeric mixture of secondary alcohol 21. Removal of the Ns group and subsequent reductive methylation afforded 22.11 After cleavage of the Alloc group, deoxygenation of the alcohol was achieved with triethylsilane under acidic conditions to give 24.12
After protection of the indole NH with a Boc group, isomerization of the double bond was performed (Scheme 5). Thus, successive treatment of α,β-unsaturated ester 25 with lithium 2,2,6,6-tetramethylpiperidide (LTMP) and quenching with a bulky phenol, 2,6-di(t-Bu)phenol, furnished β,γ-unsaturated ester 26 with a 3:1 diastereoselectivity. The diastereomers were separated and each isomer was subjected to a Heck reaction. While the attempted Heck reaction of the minor isomer resulted in the formation of an intractable mixture, the major isomer gave 27, suggesting that the isomerization afforded the desired trans isomer as the major isomer. Finally, the Boc group was cleaved with trifluoroacetic acid to give 28,13 which, according to the Oppolzer’s procedure,2e can be transformed into lysergic acid.
In summary, we have successfully constructed the tetracyclic ergoline skeleton, which features an acylation at the C3 position of the indole as well as an intramolecular Heck reaction. The optically active D ring unit was synthesized by a disrotatory electrocyclic reaction of a dibromocyclopropane, which, to the best of our knowledge, is the first example of an optically active dihydropyridine prepared from L-pyroglutamic acid.
ACKNOWLEDGEMENTS
This work was financially supported in part by Grant-in-Aids (15109001, 16073205, and 20002004) from the Ministry of Education, Culture, Sports, Science and Technology of Japan.
References
1. For reviews of ergot alkaloids, see: (a) A. Stoll and A. Hofmann, ‘The Alkaloids,’ Vol. 8, ed. by R. H. F. Manske, Academic Press, Inc., NewYork, 1965, pp. 725-783; (b) P. A. Stadler and P. Stütz, ‘The Alkaloids,’ Vol. 15, ed. by R. H. F. Manske, Academic Press, Inc., New York, 1975, pp. 1-40; (c) I. Ninomiya and T. Kiguchi, ‘The Alkaloids,’ Vol. 38, ed. by A. Brossi, Academic Press, Inc., New York, 1990, pp. 1-156; (d) D. Gröger and H. G. Floss, ‘The Alkaloids,’ Vol. 50, ed. by G. A. Cordell, Academic Press, Inc., New York, 1998, pp. 171-218; (e) M. Somei, Y. Yokoyama, Y. Murakami, I. Ninomiya, T. Kiguchi, and T. Naito, ‘The Alkaloids,’ Vol. 54, ed. by G. A. Cordell, Academic Press, Inc., New York, 2000, pp. 191-257; (f) C. L. Schardl, D. G. Panaccione, and P. Tudzynski, ‘The Alkaloids,’ Vol. 63, ed. by G. A. Cordell, Academic Press, Inc., New York, 2006, pp. 45-86.
2. (a) E. C. Kornfeld, E. J. Fornefeld, G. B. Kline, M. J. Mann, D. E. Morrison, R. G. Jones, and R. B. Woodward, J. Am. Chem. Soc., 1956, 78, 3087; CrossRef (b) M. Julia, F. L. Goffic, J. Igolen, and M. Baillarge, Tetrahedron Lett., 1969, 10, 1569; CrossRef (c) V. W. Armstrong, S. Coulton, and R. Ramage, Tetrahedron Lett., 1976, 17, 4311; CrossRef (d) R. Ramage, V. W. Armstrong, and S. Coulton, Tetrahedron Suppl., 1981, 37, 157; CrossRef (e) W. Oppolzer, E. Francotte, and K. Battig, Helv. Chim. Acta, 1981, 64, 478; CrossRef (f) J. Rebek, Jr., D. F. Tai, and Y. K. Shue, J. Am. Chem. Soc., 1984, 106, 1813; CrossRef (g) I. Ninomiya, C. Hashimoto, T. Kiguchi, and T. Naito, J. Chem. Soc., Perkin Trans. 1, 1985, 941; CrossRef (h) T. Kurihara, T. Terada, and R. Yoneda, Chem. Pharm. Bull., 1986, 34, 442; (i) S. Cacchi, P. G. Ciattini, E. Morera, and G. Ortar, Tetrahedron Lett., 1988, 29, 3117; CrossRef (j) J. B. Hendrickson and J. Wang, Org. Lett., 2004, 6, 3; CrossRef (k) S. Inuki, S. Oishi, N. Fujii, and H. Ohno, Org. Lett., 2004, 10, 5239. CrossRef
3. (a) I. Moldvai, E. Temesvári-Major, M. Incze, É. Szentirmay, E. Gács-Baitz, and C. Szántay, J. Org. Chem., 2004, 69, 5993; CrossRef (b) I. Moldvai, E. Temesvári-Major, M. Incze, G. Dornyei, É. Szentirmay, and C. Szántay, Helv. Chim. Acta, 2005, 88, 1344. CrossRef
4. T. Kurokawa, M. Isomura, H. Tokuyama, and T. Fukuyama, Synlett, in press.
5. For a recent review of electrocyclic reactions of dibromocyclopropanes, see: B. Halton and J. Harvey, Synlett, 2006, 1975. CrossRef
6. B. Felix, de M. Pedro, F. Marta, F. Josep, G. Timothy, and M. Sergio, Tetrahedron: Asymmetry, 2002, 13, 437. CrossRef
7. L. Birkofer, E. Bierwirth, and A. Ritter, Chem. Ber., 1961, 94, 821. CrossRef
8. 17: [α]D24 +7.46 (c 0.44, CHCl3); IR (film) 1718, 1544, 1439, 1370, 1281, 1168, 1120, 1095, 1062, 762 cm—1; 1H NMR (CDCl3, 400 MHz) δ 8.09-8.12 (m, 1H), 7.58-7.75 (m, 3H), 7.00 (br s, 1H), 4.99 (t, J = 3.2 Hz, 1H), 4.31 (d, J = 15.6 Hz, 1H), 4.11 (d, J = 15.6 Hz, 1H), 3.75 (s, 3H), 2.86 (br s, 2H); 13C NMR (CDCl3, 100 MHz) δ 175.6, 164.7, 147.6, 134.6, 133.9, 131.9, 131.8, 131.0, 126.6, 124.6, 52.2, 52.0, 41.5, 27.9; HRMS (FAB) Calcd for C14H14N2O8S (M+): 370.0471, Found: 370.0475.
9. L. Ghosez, B. Haveaux, and H. G. Viehe, Angew. Chem., Int. Ed. Engl., 1969, 8, 454; CrossRef B. Haveaux, A. Dekoker, M. Rens, A. R. Sidani, J. Toye, and L. Ghosez, Org. Synth. Coll. Vol. VI, 1982, 282.
10. J. Bergman and L. Venemalm, Tetrahedron Lett., 1987, 28, 3741. CrossRef
11. While removing the Ns group, partial cleavage of the Alloc group concomitantly occurred to afford a mixture of 32 and 33. 33 could be converted into 23 via a reductive amination (NaBH3CN, AcOH, aq HCHO, MeOH, rt).
12. 24: [α]D24 –15.7 (c 0.38, CHCl3); IR (film) 2949, 2925, 1700, 1653, 1437, 1397, 1336, 1305, 1267, 1192, 1158, 1141, 1098, 1067, 1042, 1025, 911, 800, 768 cm–1; 1H NMR (CDCl3, 400 MHz) δ 8.30 (br, 1H), 7.29 (d, J = 7.8 Hz, 1H), 7.26 (s, 1H), 7.05 (br, 1H), 7.00 (t, J = 7.8 Hz, 1H), 6.98 (br, 1H), 3.75 (s, 3H), 3.56 (dd, J= 14.1, 4.1 Hz, 1H), 3.52 (dd, J = 17.2, 3.4 Hz, 1H), 3.35 (dd, J = 17.2, 3.4 Hz, 1H), 3.16-3.19 (m, 1H), 2.75 (dd, J = 14.1, 10.3 Hz, 1H), 2.60 (s, 3H), 2.20 (s, 2H); 13C NMR (CDCl3, 100 MHz) δ 166.4, 137.6, 137.6, 128.0, 125.4, 124.6, 124.1, 122.8, 114.2, 113.7, 110.6, 57.6, 51.6, 51.2, 41.0, 28.7, 25.6; HRMS (FAB) Calcd for C17H19BrN2O2 (M+): 362.0630, Found: 362.0632.
13. 28: 1H NMR (CDCl3, 400 MHz) δ 7.90 (br, 1H), 7.26 (d, J = 7.2 Hz, 1H), 7.21 (d, J = 7.2 Hz, 1H), 7.08 (s, 1H), 7.01 (t, J = 7.2 Hz, 1H), 6.88 (s, 1H), 4.10 (s, 1H), 3.70 (s, 3H), 3.52 (d, J = 18.4 Hz, 1H), 3.49-3.40 (m, 3H), 3.38 (d, J = 18.4 Hz, 1H), 3.02 (dd, J = 14.7, 4.6 Hz, 1H), 2.77 (dd, J = 14.7, 11.9 Hz, 1H), 2.63 (s, 3H); HRMS (FAB) Calcd for C17H18N2O2 (M+): 281.1290, Found: 281.1284.