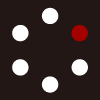
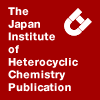
HETEROCYCLES
An International Journal for Reviews and Communications in Heterocyclic ChemistryWeb Edition ISSN: 1881-0942
Published online by The Japan Institute of Heterocyclic Chemistry
e-Journal
Full Text HTML
Received, 29th September, 2008, Accepted, 28th November, 2008, Published online, 2nd December, 2008.
DOI: 10.3987/COM-08-S(D)44
■ Syntheses of N-Acylisoxazolidine Derivatives, Related to a Partial Structure Found in Zetekitoxin AB, a Golden Frog Poison
Toshio Nishikawa,* Daisuke Urabe, and Minoru Isobe
Graduate of School, Nagoya University, Chikusa, Nagoya, Aichi 464-8601, Japan
Abstract
Syntheses of four N-acylisoxazolidine derivatives related to a partial structure of zetekitoxin AB were described. 13C NMR spectra of these compounds could not explain an unusual chemical shift observed in the N-acylisoxazolidine moiety of zetekitoxin.INTRODUCTION
In 1969, H. Mosher and co-workers reported isolation of zetekitoxin, a water soluble toxic compound from Panamanian Golden Frog, Atelopus zeteki.1 Pharmacological studies revealed that the biological profile of zetekitoxin is very similar to tetrodotoxin and saxitoxin, well-known voltage-dependent Na+ channel blocker.2 However, since the frog is designated as an endangered species,3 further collection of the frog is difficult and the structure remains to be clarified.
In 2004, Yamashita, Daly and co-workers proposed the structure of zetekitoxin AB to be as depicted for compound 1 (Figure 1) from extensive NMR and MS analyses using about 0.3 mg of the remaining sample.4 The structure of zetekitoxin is closely related to saxitoxin (2),5 a paralytic shellfish poison, but possesses two additional unique structural features such as a 10-membered macrolactam fused with isoxazolidine and a N-hydroxyl carbamate connected to guanidine through a methylene unit. Interestingly, a carbon signal observed at 156.5 ppm in the 13C NMR spectrum was assigned to the carbonyl carbon at the C-13 position, a carbonyl carbon of the N-acylisoxazolidine. Since carbonyl carbon of the N-alkoxyamide is generally observed around 170-175 ppm (ex. a Weinreb amide (3) in Figure 1), the value of 156.5 ppm is clearly unusual. One possible explanation may be an effect of the guanidinium groups at α- and β- position of the amide carbonyl group. We found that 13C-NMR spectrum of bicyclic isoxazolidine 46 showed a chemical shift at 155 ppm for the carbonyl carbon, suggesting a strong effect of the nitro group at the α-position of the amide.
In order to find out the reason(s) for the unusual value of the chemical shift observed in zetekitoxin, we planed to synthesize four compounds 5, 6, 7, and 8 related to the partial structure of zetekitoxin (Figure 2). 13C-NMR spectra of these compounds should clarify the effect of the guanidinium group on the chemical shift of the carbonyl carbon.
Syntheses of N-acylisoxazolidines
N-Acyl isoxazolidine 57 was prepared by acetylation of the known isoxazolidine hydrochloride 98 (Eq. 1).
Synthesis of 6 commenced with a nitro aldol reaction of the known aldehyde 109 with nitromethane in the presence of KF as a base10 (Scheme 1). The aldol product was dehydrated under conditions using methanesulfonylation to give nitro olefin 11 in a 53% overall yield from 10. Conjugate reduction was carried out with NaBH4 in the presence of silica gel11 to afford 12 in high yield. Upon treatment of 12 with Boc2O and DMAP,12 the resulting nitrile oxide 13 underwent an intramolecular 1,3-dioplar cycloaddition to give a diastereomeric mixture of 14a and 14b in 64 and 22% yield, respectively. After separation by silica gel column chromatography, the major product 14a was reduced with NaBH3CN in acetic acid followed by acetylation to give N-acetylisoxazolidine 15. On the other hand, the minor product 14b was transformed to N-acetylisoxazolidine 16 by similar reactions. The stereochemistries of 15 and 16 were determined by NOESY spectra as shown in Scheme 1. After hydrolysis of the acetonide of major product 15 with TFA in aqueous methanol, the resulting 1,2-diol was cleaved with NaIO4, and subsequent reduction with NaBH4 afforded a 15:1 mixture of diol 613 and its epimer in a combined yield of 69%. The stereochemistry of 6 was confirmed by conducting NOESY experiments on tetrahydropyran 18 derived from 6 under the Mitsunobu condition.
N-Acyl isoxazolidine 7 was synthesized by coupling isoxazolidine hydrochloride 9 with Fmoc-glycine 19 (Scheme 2). The coupling was carried out by EDCI and HOBt to give amide 20 in 55% yield. The Fmoc group was deprotected with morpholine and the resulting crude amine was directly treated with Boc-protected isothiourea (21) in one-pot to afford di-Boc guanidine 2214 in 60% overall yield. The two Boc groups were then deprotected with TFA in CH2Cl2 to give N-acyl isoxazolidine 715 in 69% yield after recrystallization from EtOH. NMR spectra of compound 7 was measured in DMSO-d6, since 7 forms the 5-membered guanidine 23 in D2O via an intramolecular acylation.
N-Acyl isoxazolidine 8 would be synthesized from a commercially available DL-2,3-diaminopropionic acid monohydrochloride 24. The two amino groups of 24 were protected with Fmoc groups, and the resulting 25 was coupled with isoxazolidine hydrochloride 9 in the presence of EDCI and HOBt to give amide 26 in 72% yield. Deprotection of the Fmoc groups was followed by guanidinylation with Boc-protected isothiourea (21) in the presence of HgCl2 to afford bis-di-Boc guanidine 27.16 However, the formation of guanidine 8 by deprotection of the four Boc groups under acidic conditions was unsuccessful, probably due to a similar intramolecular acylation of guanidine as encountered in the synthesis of 7.
With the synthetic compounds 5, 6, 7, and 27 in our hands, 13C NMR chemical shifts of the amide carbonyl group of these compounds were compared with zetekitoxin AB (Table 1). Although the model compound 8 could not be prepared, 13C NMR spectrum of 27 was used instead of 8 for the comparison, because the Boc groups of the guanidine have little influence on the chemical shifts of the amide carbon, judging from comparison between 22 and 7 (Table 1). The model compounds 5 and 22 show two different amide absorptions, respectively, due to the existence of amide rotamers. Chemical shifts for the amide carbon of 5 and 6 were observed in the usual range for amide carbons. Chemical shifts for the amide carbon in 7 and 27 were shifted upfield by about 5 ppm due to the inductive effect of guanidine at the α-position. The guanidine function at β-position has little influence on the amide carbon.
CONCLUSION
We have synthesized N-acyl isoxazolidine derivatives 5, 6, 7 and 27, and measured 13C NMR spectra of these synthetic compounds. Comparison of the 13C NMR chemical shift of 5, 6, 7, 27 and zetekitoxin led us to conclude that the unusual chemical shift of the C-13 of zetekitoxin AB could not be rationalized only by the substituent effect of the guanidinium groups. The chemical shift at the C-13 might be explained by unknown factor(s) derived from the rigid 10-membered macrolactam structure of zetekitoxin. Further efforts to find the factor are in progress in our laboratory.
ACKNOWLEDGEMENTS
This work was financially supported by PRESTO, JST, a Grand-in Aid for Scientific Research, 21st century COE program and Global-COE program from MEXT, and the Naito Foundation. We thank Prof. M. Yotsu-Yamashita (Tohoku University, Japan) and Dr. M. Uchiyama (RIKEN, Japan) for valuable discussion. We thank Dr. M. Sydnes for valuable help during manuscript preparation.
References
1. F. A. Fuhrman, G. J. Fuhrman, and H. S. Mosher, Science, 1969, 165, 1376. CrossRef
2. G. B. Brown, Y. H. Kim, H. Küntzel, H. S. Mosher, G. J. Fuhrman, and F. A. Fuhrman, Toxicon, 1977, 15, 115. CrossRef
3. See: The IUCN Red list of Threatened Species, (http://www.iucnredlist.org/static/introduction).
4. M. Yotsu-Yamashita, Y. H. Kim, S. C. Dudley, G. Choudhary, A. Pfahnl, Y. Oshima, and J. W. Daly, Proc. Nalt. Acad. Sci. USA, 2004, 101, 4346. CrossRef
5. For a recent review on saxitoxin, see: L. E. Llewellyn, Nat. Prod. Rep., 2006, 23, 200. CrossRef
6. K. S.-L. Huang, E. H. Lee, M. M. Olmstead, and M. J. Kurth, J. Org. Chem., 2000, 65, 499. CrossRef
7. Compound (5): 1H NMR (400 MHz, CDCl3) δ 2.32 (2H, quintet J = 7 Hz, CH2-CH2-CH2), 3.71 (2H, t, J = 7 Hz, N-CH2), 3.97 (2H, t, J = 7 Hz, O-CH2). 13C NMR (75 MHz, CDCl3) δ 20.2, 20.7, 27.5, 42.9, 69.1, 170.7, 173.3. Anal. Calcd for C5H9NO2: C, 52.16; H, 7.88; N, 12.17. Found: C, 52.16; H, 7.97; N, 12.38.
8. T. L. Cupps, R. H. Boutin, and H. Rapoport, J. Org. Chem., 1985, 50, 3972. CrossRef
9. H. R. Moon, W. J. Choi, H. O. Kim, and L. S. Jeong, Tetrahedron: Asymmetry, 2002, 13, 1189. CrossRef
10. (a) R. H. Wollenberg and S. J. Miller, Tetrahedron Lett., 1978, 19, 3219; CrossRef (b) R. V. Stevens, N. Beaulieu, W. H. Chan, A. R. Daniewski, T. Takeda, A. Waldner, P. G. Williard, and U. Zutter, J. Am. Chem. Soc., 1986, 108, 1039. CrossRef
11. A. K. Sinhababu and R. T. Borchardt, Tetrahedron Lett., 1983, 24, 227. CrossRef
12. Y. Basel and A. Hassner, Synthesis, 1997, 309. CrossRef
13. Compound (6): 1H NMR (400 MHz, CDCl3) δ 1.52 (1H, m, CHAHB-CH2-O), 1.86 (1H, dddd, J = 14, 12, 5, 3 Hz, CHAHB-CH2-O), 2.16 (3H, s, Ac), 2.94 (1H, m, -CH-), 3.58 (1H, td, J = 12, 3 Hz, CH2-CHAHB), 3.69 (1H, m, CH-CHAHB), 3.70 (1H, dd, J = 10.5, 8 Hz, CH-CHAHB-OH), 3.83 (1H, dd, J = 10.5, 6 Hz, CH-CHAHB-OH), 4.04 (2H, d, J = 6 Hz, CH2-O), 4.67 (1H, ddd, J = 12, 8, 6 Hz, N-CH). 13C NMR (75 MHz, CDCl3) δ 20.1, 31.2, 46.2, 54.2, 58.6, 59.7, 72.0, 172.7. HRMS (FAB) for C8H16NO4 (M+H), calcd 190.1079, found 190.1066. Compound (6): 1H NMR (400 MHz, CDCl3) δ 1.52 (1H, m, CHAHB-CH2-O), 1.86 (1H, dddd, J = 14, 12, 5, 3 Hz, CHAHB-CH2-O), 2.16 (3H, s, Ac), 2.94 (1H, m, -CH-), 3.58 (1H, td, J = 12, 3 Hz, CH2-CHAHB), 3.69 (1H, m, CH-CHAHB), 3.70 (1H, dd, J = 10.5, 8 Hz, CH-CHAHB-OH), 3.83 (1H, dd, J = 10.5, 6 Hz, CH-CHAHB-OH), 4.04 (2H, d, J = 6 Hz, CH2-O), 4.67 (1H, ddd, J = 12, 8, 6 Hz, N-CH). 13C NMR (75 MHz, CDCl3) δ 20.1, 31.2, 46.2, 54.2, 58.6, 59.7, 72.0, 172.7. HRMS (FAB) for C8H16NO4 (M+H), calcd 190.1079, found 190.1066.
14. Compound (22): 1H NMR (400 MHz, CDCl3) major peak; δ 1.50 (18H, s, Boc x 2), 2.33 (2H, quintet J = 7 Hz, CH2-CH2-CH2), 3.75 (2H, t, J = 7.5 Hz, N-CH2), 3.99 (2H, t, J = 7 Hz, O-CH2), 4.34 (2H, d, J = 4.5 Hz, CO-CH2), 9.08 (1H, m, NH), 11.40 (1H, br s, NH). minor peak; δ 1.51 (18H, s, Boc x 2), 3.45 (2H, t, J = 5 Hz, CH2-CH2-CH2), 3.67 (4H, m, CH2-N, CH2-O), 4.25 (2H, d, J = 4 Hz, CH2-NH), 9.42 (1H, m, NH), 11.39 (1H, m, NH). 13C NMR (75 MHz, CDCl3) δ 27.3, 28.0, 28.2 (two peaks), 42.3, 42.5, 42.9, 43.5, 44.8, 66.3, 66.7, 69.4, 79.3, 79.7, 83.1, 83.3, 152.6, 152.7, 155.4, 155.8, 162.8, 163.1, 166.0, 168.2. Anal. Calcd for C16H28N4O6: C, 51.60; H, 7.58; N, 15.04. Found: C, 51.60; H, 7.50; N, 14.89.
15. Compound (7): 1H NMR (400 MHz, DMSO-d6) δ 2.26 (2H, quintet, J = 7 Hz, CH2-CH2-CH2), 3.69 (2H, t, J = 7 Hz, N-CH2), 3.97 (2H, t, J = 7 Hz, O-CH2), 4.10 (2H, d, J = 5 Hz, CO-CH2), 7.20-7.72 (5H, m, NH). 13C NMR (100 MHz, DMSO-d6) δ 27.3, 42.4, 43.9, 69.4,157.7, 168.3. HRMS (FAB) for C6H13N4O2 (M+H), calcd 173.1039, found 173.1078.
16. Compound (27): 1H NMR (400 MHz, DMSO-d6) δ 1.38 (18H, s, Boc x 2), 1.45 (9H, s, Boc), 1.46 (9H, s, Boc), 2.30 (2H, m, CH2CH2CH2), 3.47 (1H, m, N-CHAHB), 3.60-3.82 (3H, m, N-CHAHB, CH2NH), 4.10 (1H, m, O-CHAHB), 4.17 (1H, m, O-CHAHB), 5.06 (1H, m, CH), 8.42 (1H, br s, NH), 8.76 (1H, br s, NH), 11.41 (1H, br s, NH), 11.45 (1H, br s, NH). 13C NMR (100 MHz, DMSO-d6) δ 15.3, 25.6, 27.3, 27.7, 27.8, 28.1, 30.6, 31.5, 43.7, 50.9, 51.0, 55.1, 65.1, 69.5, 78.3, 78.4, 78.5, 8 .0, 83.1, 83.2, 83.3, 148.5, 151.2, 151.8, 151.9 (two peaks), 155.0, 156.2, 162.8, 162.9, 163.0, 163.1, 167.0. HRMS (FAB) for C28H50N7O10 (M+H), calcd 644.3619, found 644.3593.