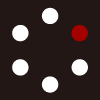
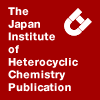
HETEROCYCLES
An International Journal for Reviews and Communications in Heterocyclic ChemistryWeb Edition ISSN: 1881-0942
Published online by The Japan Institute of Heterocyclic Chemistry
e-Journal
Full Text HTML
Received, 29th September, 2008, Accepted, 28th November, 2008, Published online, 2nd December, 2008.
DOI: 10.3987/COM-08-S(D)45
■ Convergent Synthesis of Fluorescence Labeled Solamin
Naoto Kojima, Takekuni Morioka, Masahiro Yano, Yuki Suga, Naoyoshi Maezaki, and Tetsuaki Tanaka*
Medicinal and Organic Chemsitry, Graduate School of Pharmaceutical Science, Osaka University, 1-6 Yamadaoka, Suita, Osaka 560-0871, Japan
Abstract
The convergent synthesis of dansyl-labeled solamin, an antitumor Annonaceous acetogenin, has been achieved. The carbon skeleton was assembled from three fragments: the THF ring fragment, the fluorescent fragment, and the γ-lactone fragment, by asymmetric alkynylation.Annonaceous acetogenins are waxy derivatives of long-chain fatty acids (C32 or C34) that are linked by a 2-propanol unit at C-2 position to form a γ-methyl-α,β-unsaturated-γ-lactone.1 Most acetogenins are characterized by one to three 2,5-disubstituted tetrahydrofuran(s) (THFs) with one or two flanking hydroxyl group(s) at the center of a long carbon chain. Many researchers are engaged in the synthesis of acetogenins2 and their analogues3 due to their unique structures and potent antitumor activities. The mechanism of action is considered to involve the inhibition of mitochondrial NADH ubiquinone oxidoreductase (complex I). The inhibition suppresses ATP production, leading to apoptosis of cancer cells.4 However, the structure–activity relationship for complex I inhibition is not completely related to its cytotoxicity. McLaughlin and co-workers suggested that as the mitochondrial assay is cell-free, it does not take into consideration factors such as membrane transport, intracellular transport, and metabolic inactivation.5 In order to visualize cell distribution, Poupon and co-workers synthesized a γ-lactone-free dansyl-labeled squamocin analogue.6 Moreover, Yao and co-workers reported the synthesis of a new fluorescent acetogenin analogue whose THF rings were replaced with acyclic ethers for simplification.7 In living cells, it was suggested that the hydrophilic THF moiety in natural acetogenins is located at the surface of the mitochondrial inner membrane and that the γ-lactone moiety interacts with the active site of the membrane.8 Therefore, we synthesized 7-nitrobenzo[c][1,2,5]oxadiazol-4-yl-amino (NBD-NH-) solamin (2) that retains all functionalities.9 However, the total yield of our synthesis was low and the preparation of other derivatives was inconvenient because the synthetic route was linear. Herein, we report a novel approach to labeled solamins and its application to 5-dimethylaminonaphthalen-1-yl-sulfonamide (dansyl-NH-) solamin (3), a fluorescent mono-THF annonaceous acetogenin, bearing all functionalities.
The retrosynthesis of fluorescent acetogenins is summarized in Scheme 1. Fluorescent acetogenins (4) are divided into three fragments: fluorescent fragment (5), THF fragment (6), and γ-lactone fragment (7). The fragments could be connected by reagent-controlled asymmetric alkynylation. This synthetic plan should provide ready access to diverse fluorescent derivatives that have other fluorescent groups or long hydrocarbon chains as linker.
Synthesis of THF fragment (6) started from known aldehyde (8) and 3-butyne-1,2-diol derivative (9)10 prepared from 8 (Scheme 2). Asymmetric alkynylation of aldehyde (8) with alkyne (9) was carried out under Carreira’s conditions11 with (1R,2S)-N-methylephedrine as chiral ligand to give syn-adduct12 (10) in 93% yield with high diastereoselectivity (>97:3 dr). Hydrogenation of the triple bond and deprotection of the benzylidene acetal gave triol (11) in 84% yield. Selective sulfonylation of primary alcohol followed by base treatment of resulting 12 afforded trans/threo THF-ring (13). DMSO oxidation of 13 with SO3·pyridine complex gave α-tetrahyrofuranyl aldehyde (6) in good yield.
Fluorescent fragment (16) was prepared from known iodoalkyne (14)13 (Scheme 3). Azidation of 14 was carried out to give azide (15) in high yield. The reduction of 15 under Staudinger conditions followed by coupling with dansyl chloride afforded fluorescent fragment (16).
γ-Lactone fragment (7) was easily prepared by alkylation of known α-sulfenyl-γ-lactone (18)14 with 12-iodo-1-dodecyne (17) 15 under basic conditions in high yield (Scheme 4).
With all the fragments in hand, we examined the assembly of THF fragment (6) and γ-lactone fragment (7) because we thought it best that the expensive fluorescent fragment (16) be introduced at the late stage of the synthesis (Scheme 5). Coupling reaction of the two fragments (6 and 7) was accomplished by asymmetric alkynylation with high stereoselectivity (95:5 dr).16 After the secondary alcohol of adduct (19) was protected as a TBDPS ether, the hydrolysis of acetonide gave diol (21). Oxidative cleavage of 1,2-diol moiety gave α-tetrahydrofuranyl aldehyde (22) in 98% yield.
The introduction of fluorescent fragment (16) into α-tetrahydrofuranyl aldehyde (22) proceeded successfully, giving desired propargyl alcohol (23) in 66% yield with high stereoselectivity (91:9 dr).16 Resulting diyne (23) was hydrogenated on Wilkinson’s catalyst to give alcohol (24) in 73% yield. Oxidation of the sulfide followed by thermal elimination of the resulting sulfoxide afforded α,β-unsaturated-γ-lactone (25). Synthesis of dansyl-labeled solamin (3) was completed via deprotection of TBDPS ether under acidic conditions.17
In conclusion, we have accomplished the convergent synthesis of fluorescent solamin bearing all functionalities from 8 in 7.5% yield over 14 steps. Our synthesis should provide ready access to diverse derivatives labeled by other fluorescent groups. Further synthesis of other fluorescence labeled acetogenins and visualization of cell distribution are under way.
ACKNOWLEDGEMENT
We acknowledge financial support through a Grant-in-Aid for Scientific Research on Priority Area ‘Creation of Biologically Functional Molecules’ from The Ministry of Education, Culture, Sports, Science, and Technology, Japan.
References
1. For reviews on Annonaceous acetogenins: (a) J. L. McLaughlin, J. Nat. Prod., 2008, 71, 1311; CrossRef (b) A. Bermejo, B. Figadère, M.-C. Zafra-Polo, I. Barrachina, E. Estornell, and D. Cortes, Nat. Prod. Rep., 2005, 22, 269. CrossRef
2. For recent total synthesis of acetogenins: (a) H. Konno, Y. Okuno, H. Makabe, K. Nosaka, A. Onishi, Y. Abe, A. Sugimoto, and K. Akaji, Tetrahedron Lett., 2008, 49, 782; CrossRef (b) Y. Hattori, Y. Kimura, A. Moroda, H. Konno, M. Abe, H. Miyoshi, T. Goto, and H. Makabe, Chem. Asian J., 2006, 1, 894; CrossRef (c) N. G. Bandur, D. Brueckner, R. W. Hoffmann, and U. Koert, Org. Lett., 2006, 8, 3829; CrossRef (d) S. Takahashi, Y. Hongo, N. Ogawa, H. Koshino, and T. Nakata, J. Org. Chem., 2006, 71, 6305; CrossRef (e) J. A. Marshall and J. J. Sabatini, Org. Lett., 2006, 8, 3557; CrossRef (f) D. P. Curran, Q. Zhang, C. Richard, H. Lu, V. Gudipati, and C. S. Wilcox, J. Am. Chem. Soc., 2006, 128, 9561; CrossRef (g) T. R. Hoye, B. M. Eklov, J. Jeon, and M. Khoroosi, Org. Lett., 2006, 8, 3383; CrossRef (h) V. Gudipati, D. P. Curran, and C. S. Wilcox, J. Org. Chem., 2006, 71, 3599; CrossRef (i) D. Strand, P.-O. Norrby, and T. Rein, J. Org. Chem., 2006, 71, 1879; CrossRef (j) H. Tominaga, N. Maezaki, M. Yanai, N. Kojima, D. Urabe, R. Ueki, and T. Tanaka, Eur. J. Org. Chem., 2006, 1422. CrossRef
3. For recent synthesis of acetogenin analogues: (a) N. Kojima, T. Fushimi, N. Maezaki, T. Tanaka, and T. Yamori, Bioorg. Med. Chem. Lett., 2008, 18, 1637; CrossRef (b) H.-X. Liu, G.-R. Huang, H.-M. Zhang, J.-R. Wu, and Z.-J. Yao, Bioorg. Med. Chem. Lett., 2007, 17, 3426; CrossRef (c) J. A. Marshall, J. J. Sabatini, and F. Valeriote, Bioorg. Med. Chem. Lett., 2007, 17, 2434; CrossRef (d) R. A. Duval, E. Poupon, V. Romero, E. Peris, G. Lewin, D. Cortes, U. Brandt, and R. Hocquemiller, Tetrahedron, 2006, 62, 6248; CrossRef (e) S. Derbre, R. Duval, G. Roue, A. Garofano, E. Poupon, U. Brandt, S. A. Susin, and R. Hocquemiller, ChemMedChem, 2006, 1, 118; CrossRef (f) R. A. Duval, G. Lewin, E. Peris, N. Chahboune, A. Garofano, S. Droese, D. Cortes, U. Brandt, and R. Hocquemiller, Biochemistry, 2006, 45, 2721, and references cited therein. CrossRef
4. (a) D. J. Morré, R. de Cabo, C. Farley, N. H. Oberlies, and J. L. McLaughlin, Life Sci., 1995, 56, 343; CrossRef (b) E. J. Wolvetang, K. L. Johnson, K. Krauer, S. J. Ralph, and A. W. Linnane, FEBS Lett., 1994, 339, 40. CrossRef
5. N. H. Oberlies, C. Chang, and J. L. McLaughlin, J. Med. Chem., 1997, 40, 2102. CrossRef
6. S. Derbré, G. Roué, E. Poupon, S. A. Susin, and R. Hocquemiller, ChemBioChem, 2005, 6, 979. CrossRef
7. H.-X. Liu, G.-R. Huang, H.-M. Zhang, S. Jiang, J.-R. Wu, and Z.-J. Yao, ChemBioChem, 2007, 8, 172. CrossRef
8. (a) K. Kuwabara, M. Takada, J. Iwata, K. Tatsumoto, K. Sakamoto, H. Iwamura, and H. Miyoshi, Eur. J. Biochem., 2000, 267, 2538, and references cited therein; CrossRef (b) H. Shimada, J. B. Grutzner, J. F. Kozlowski, and J. L. McLaughlin, Biochemistry, 1998, 37, 854; CrossRef (c) H. Shimada, J. F. Kozlowski, and J. L. McLaughlin, Pharmacol. Res., 1998, 37, 357. CrossRef
9. N. Maezaki, D. Urabe, M. Yano, H. Tominaga, T. Morioka, N. Kojima, and T. Tanaka, Heterocycles, 2007, 73, 159. CrossRef
10. (a) N. Maezaki, N. Kojima, and T. Tanaka, Synlett, 2006, 993; CrossRef (b) N. Kojima, Yakugaku Zasshi, 2004, 124, 673; CrossRef (c) N. Kojima, N. Maezaki, H. Tominaga, M. Yanai, D. Urabe, and T. Tanaka, Chem. Eur. J., 2004, 10, 672; CrossRef (d) N. Kojima, N. Maezaki, H. Tominaga, M. Asai, M. Yanai, and T. Tanaka, Chem. Eur. J., 2003, 9, 4980; CrossRef (e) N. Maezaki, N. Kojima, H. Tominaga, M. Yanai, and T. Tanaka, Org. Lett., 2003, 5, 1411; CrossRef (f) N. Maezaki, N. Kojima, M. Asai, H. Tominaga, and T. Tanaka, Org. Lett., 2002, 4, 2977. CrossRef
11. (a) E. El-Sayed, N. K. Anand, and E. M. Carreira, Org. Lett., 2001, 3, 3017; CrossRef (b) N. K. Anand and E. M. Carreira, J. Am. Chem. Soc., 2001, 123, 9687; CrossRef (c) H. Sasaki, D. Boyall, and E. M. Carreira, Helv. Chim. Acta, 2001, 84, 964; CrossRef (d) D. Boyall, F. López, H. Sasaki, D. Frantz, and E. M. Carreira, Org. Lett., 2000, 2, 4233; CrossRef (e) D. E. Frantz, R. Fässler, C. S. Tomooka, and E. M. Carreira, Acc. Chem. Res., 2000, 33, 373; CrossRef (f) D. E. Frantz, R. Fässler, and E. M. Carreira, J. Am. Chem. Soc., 2000, 122, 1806. CrossRef
12. Absolute configurations of coupling products were determined by the modified Mosher’s method. See: (a) I. Ohtani, T. Kusumi, Y. Kashman, and H. Kakisawa, J. Am. Chem. Soc., 1991, 113, 4092; CrossRef (b) J. A. Dale and H. S. Mosher, J. Am. Chem. Soc., 1973, 95, 512; CrossRef (c) J. A. Dale, D. L. Dull, and H. S. Mosher, J. Org. Chem., 1969, 34, 2543. CrossRef
13. F. F. Knapp Jr., P. C. Srivastava, A. P. Callahan, E. B. Cunningham, G. W. Kabalka, and K. A. R. Sastry, J. Med. Chem., 1984, 27, 57. CrossRef
14. J. D. White, T. C. Somers, and G. N. Reddy, J. Org. Chem., 1992, 57, 4991. CrossRef
15. A. J. Valicenti, F. J. Pusch, and R. T. Holman, Lipids, 1985, 20, 234. CrossRef
16. Stereoselectivity of the coupling products was determined from the 1H NMR data of their acetyl derivatives.
17. The stereochemistry of 3 was determined by comparison with 13C NMR data of Fujimoto's model compounds. See: Y. Fujimoto, C. Murasaki, H. Shimada, S. Nishioka, K. Kakinuma, S. Singh, M. Singh, Y. Gupta, and M. Sahai, Chem. Pharm. Bull., 1994, 42, 1175.