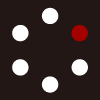
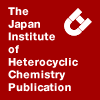
HETEROCYCLES
An International Journal for Reviews and Communications in Heterocyclic ChemistryWeb Edition ISSN: 1881-0942
Published online by The Japan Institute of Heterocyclic Chemistry
e-Journal
Full Text HTML
Received, 29th September, 2008, Accepted, 17th November, 2008, Published online, 20th November, 2008.
DOI: 10.3987/COM-08-S(D)46
■ An Efficient and Selective Synthetic Method for Fluorine-Containing Benzo[h]quinolines and 1H-Benzo[h]quinolin-2-ones from N-Propargyl-2,4-bis(trifluoroacetyl)-1-naphthylamine
Etsuji Okada,* Dai Shibata, Norikado Tsukushi, Masato Dohura, and Maurice Médebielle
Department of Chemical Science and Engineering, Kobe University, Rokkodai-cho, Nada-ku, Kobe 657-8501, Japan
Abstract
N-Propargyl-2,4-bis(trifluoroacetyl)-1-naphthylamine (3) underwent nitrogen-containing heterocyclic ring-formation reactions with active methylene compounds such as dialkyl malonates in the presence of sodium alkoxides. This ring closure reactions were very dependent on reaction temperature to give selectively the corresponding fluorine-containing benzo[h]quinolines (5) at high temperature and 1H-benzo[h]quinolin-2-ones (7 and 8) at low temperature.In recent years considerable attention has been paid to the development of new methodologies for the synthesis of many kinds of fluorine-containing heterocycles, since these compounds are now widely recognized as important organic materials showing interesting biological activities for their potential use in medicinal and agricultural scientific fields.1 Benzo[h]quinoline and the related derivatives are important heterocyclic systems, constituting the structure of many naturally occurring products and having interesting biological activities such as antimicrobial agents and antitumor drugs.2 They are also known to be applicable to potent and selective 5α-reductase inhibitors.3
Previously, we have found that N,N-dimethyl-2,4-bis(trifluoroacetyl)-1-naphthylamine (1) undergoes novel aromatic nucleophilic substitution with various amines, thiols and alcohols to give the corresponding N-N, N-S and N-O exchanged products (2) in excellent yields, respectively (Scheme 1).4
Furthermore, we succeeded in applying this type of aromatic nucleophilic substitution and the subsequent cyclizations to the simple syntheses of various naphthalene-fused heterocycles bearing trifluoromethyl groups.5 In continuation of these studies, it was found that N-propargyl-2,4-bis(trifluoroacetyl)-1-
naphthylamine (3), prepared by N-N exchange reaction of 1 with propargylamine, undergoes novel pyridine-ring formation reaction with various N-, S- and O-nucleophiles to give the corresponding fluorine-containing benzo[h]quinolines (4) in excellent yields (Scheme 2).6
In this communication we wish to report a facile and selective synthetic method for fluorine-containing benzo[h]quinolines (5) and 1H-benzo[h]quinolin-2-ones (7 and 8) by the ring closure reaction of 3 with C-nucleophiles, dialkyl malonates (active methylene compounds) in the presence of sodium alkoxides, which was highly dependent on reaction temperature.
The results of the pyridine-ring formation reaction of 3 with dialkyl malonates are depicted in Scheme 3 and summarized in Table 1.7 Reaction of 3 with dimethyl malonate (3 equiv) proceeded cleanly in the presence of sodium methoxide (1 equiv) in refluxing methanol to afford the corresponding fluorine-containing benzo[h]quinolines (5a) having di(methoxycarbonyl)ethyl group at the 3-position in 83% yield (entry 1). The same type of reaction with diethyl malonate in ethanol was completed within 5
min at reflux temperature to give the desired benzo[h]quinolines (5b) in 77% yield (entry 2). Reaction of 3 with diisopropyl malonate under the almost same conditions provided a mixture of benzo[h]quinolines (5c) and its reduced product (6c) in 43% and 44% yields, respectively (entry 3). Separation of the mixtures into 5c and 6c was easily performed by silica gel column chromatography. In the cases of less reactive di-t-butyl malonate and diethyl methylmalonate, heating at lower temperature (30 °C) and for longer time (18 h) was required for completion of the reaction without decomposition products and afforded benzo[h]quinolines (5d and 5e) in 75% and 95% yields, respectively (entries 4 and 5).
It is noteworthy that as the reaction temperature was lowered, the reaction pathway changed dramatically (Scheme 4).8 Reaction of 3 with diethyl malonate at 0 °C yielded 4-hydroxy-3,4-dihydro-1H-benzo[h]-quinolin-2-ones (7b) and its dehydrated product, 1H-benzo[h]quinolin-2-ones (8b), in 42% and 51% yields, respectively, without any formation of benzo[h]quinolines (5b). Moreover 3 underwent the lactam ring formation with diisopropyl malonate at -20 °C to give exclusively 4-hydroxy-3,4-dihydro-1H-benzo[h]quinolin-2-ones (7c) quantitatively. Di(isopropoxycarbonyl) derivative (7c) was hard to be dehydrated compared to di(ethoxycarbonyl) derivative (7b). Therefore,
conversion of 7c into the corresponding dehydrated product (8c) was achieved by formal dehydration, namely HO-Cl exchange and subsequent dehydrochlorination, with the use of thionyl chloride in the presence of pyridine.9
Possible mechanistic pathways for the formation of benzo[h]quinolines (5) and 1H-benzo[h]quinolin-2-ones (7 and 8) are depicted in Scheme 5. At high temperature, the addition of enolates (carbanions) from dialkyl malonates occurs onto the terminal acetylenic carbon to give the corresponding cyclization product, which leads to 5 via 1,3-H shift and the subsequent departure of hydroxide ion (Path A). On the other hand, at low temperature, the addition of enolates takes place on the carbonyl carbon of trifluoroacetyl group at the 2-position, followed by the intramolecular ester-amide exchange reaction (lactam ring formation) to afford 7 leading to 8 by dehydration (Path B). It is not certain at present why the interesting temperature-dependent chemoselectivity was clearly observed in this system. Further studies are underway to elucidate definitely the mechanism.
In conclusion, we succeeded in extending the ring formation reactions of 3 with N-, S- and O-nucleophiles to those with C-nucleophiles such as active methylene compounds, dialkyl malonates, and in providing an efficient and selective synthetic method for fluorine-containing benzo[h]quinolines and 1H-benzo[h]quinolin-2-ones, which are not easily accessible by other methods. Further works are currently continued in our laboratory and the results will be published in our forthcoming papers.
References
1. (a) R. Filler, ‘Organofluorine Chemicals and Their Industrial Applications’, Ellis Horwood, London, 1979; (b) R. Filler and Y. Kobayashi, ‘Biomedicinal Aspects of Fluorine Chemistry’, Kodansha & Elsevier Biomedical, Tokyo, 1982; (c) J. T. Welch, Tetrahedron, 1987, 43, 3123; CrossRef (d) R. Filler, Y. Kobayashi, and L. M. Yagupolskii, ‘Organofluorine Compounds in Medicinal Chemistry and Biomedical Applications’, Elsevier, Amsterdam, 1993; (e) K. Burger, U. Wucherpfennig, and E. Brunner, Adv. Heterocycl. Chem., 1994, 60, 1. CrossRef
2. (a) G. Jones, ‘Quinolines’, Wiley-Interscience, London, 1977; (b) R. P. Bahuguna, Y. C. Joshi, B. C. Dobhal, B. C. Joshi, and H. N. Mangal, Heterocycles, 1981, 16, 1955; CrossRef (c) W. A. Denny, G. J. Atwell, and B. C. Baguley, Anti-Cancer Drug Des., 1987, 2, 263; (d) R. P. Bahuguna and B. C. Joshi, Egypt. J. Chem., 1988, 31, 89; (e) R. P. Bahuguna and B. C. Joshi, J. Heterocycl. Chem., 1994, 3, 265; (f) T. Nakanishi, A. Masuda, M. Suwa, Y. Akiyama, N. Hoshino-Abe, and M. Suzuki, Bioorg. Med. Chem. Lett., 2000, 8, 2321. CrossRef
3. (a) C. D. Jones, J. E. Audia, D. E. Lawhorn, L. A. McQuaid, B. L. Neubauer, A. J. Pike, P. A. Pennington, N. B. Stamm, R. E. Toomey, and K. S. Hirsch, J. Med. Chem., 1993, 36, 421; CrossRef (b) E. C. R. Smith, L. A. McQuaid, R. L. Goode, A. M. McNulty, B. L. Neubauer, V. P. Rocco, and J. E. Audia, Bioorg. Med. Chem. Lett., 1998, 8, 395. CrossRef
4. (a) M. Hojo, R. Masuda, and E. Okada, Tetrahedron Lett., 1987, 28, 6199; CrossRef (b) M. Hojo, R. Masuda, E. Okada, and H. Miya, Synthesis, 1989, 870. CrossRef
5. (a) M. Hojo, R. Masuda, E. Okada, and H. Miya, Synthesis, 1989, 550; CrossRef (b) M. Hojo, R. Masuda, and E. Okada, Synthesis, 1990, 481; CrossRef (c) M. Hojo, R. Masuda, E. Okada, T. Tomifuji, and N. Imazaki, Synthesis, 1990, 1135; CrossRef (d) E. Okada, R. Masuda, M. Hojo, N. Imazaki, and H. Miya, Heterocycles, 1992, 34, 103; CrossRef (e) E. Okada, R. Masuda, M. Hojo, N. Imazaki, and K. Takahashi, Synthesis, 1992, 536; CrossRef (f) E. Okada, N. Tsukushi, N. Kunihiro, and Y. Tomo, Heterocycles, 1998, 49, 297. CrossRef
6. E. Okada, H. Tone, N. Tsukushi, Y. Otsuki, H. Takeuchi, and M. Hojo, Heterocycles, 1997, 45, 339. CrossRef
7. A typical experimental procedure for the synthesis of 5: Sodium (23 mg, 1.0 mmol) and diethyl malonate (481 mg, 3.0 mmol) were added to ethanol (8 mL) and the mixture was stirred at rt for 15 min. To the solution was added 34a (373 mg, 1.0 mmol) and then it was stirred for 5 min at reflux temperature. The reaction was quenched with 1M HCl and the solvent was removed under reduced pressure. The mixture was extracted with AcOEt, washed with water, and dried over Na2SO4. Evaporation of the solvent gave the crude mixture which was submitted to column chromatography on silica gel eluting with n-hexane/AcOEt (9/1) to give 5b (396 mg, 77%); mp 83-84 ℃ (n-hexane/CHCl3); 1H NMR (CDCl3): δ 9.34 (dd, 1H, J = 4.0, 7.0 Hz, H-7 or -10), 9.07 (s, 1H, H-2), 8.74 (br s, 1H, H-5), 8.56 (dd, 1H, J = 4.0, 7.0 Hz, H-10 or -7), 7.92-7.62 (m, 2H, H-8, H-9), 4.20 (q, 4H, J = 7.0 Hz, CH2CH3), 3.80-3.65 (m, 3H, CH2CH), 1.21 (t, 6H, J = 7.0 Hz, CH2CH3); 13C NMR (CDCl3): δ 182.1 (q, JCF = 34.2 Hz), 168.3 (s), 156.5 (d), 148.0 (s), 133.2 (q, JCF = 29.3 Hz), 131.5 (s), 130.7 (s), 130.4 (d), 128.9 (s), 128.5 (d), 128.2 (d), 127.6 (s), 125.3 (d), 124.9 (d), 124.6 (q, JCF = 277.9 Hz), 119.2 (s), 116.7 (q, JCF = 293.0 Hz), 62.2 (t), 53.5 (d), 31.1 (t), 14.1 (q); IR (KBr, cm-1): 1733, 1710; Anal. Calcd for C24H19F6NO5: C, 55.93; H, 3.72; N, 2.72. Found: C, 55.86; H, 3.92; N, 2.59.
8. A typical experimental procedure for the synthesis of 7: Sodium (23 mg, 1.0 mmol) and diisopropyl malonate (471 mg, 2.5 mmol) were added to isopropanol (8 mL) and the mixture was stirred at rt for 15 min. To the solution was added 3 (187 mg, 0.5 mmol) and then it was stirred at -20 ℃ for 72 h. The reaction was quenched with 1M HCl and the solvent was removed under reduced pressure. The mixture was extracted with AcOEt, washed with water, and dried over Na2SO4. Evaporation of the solvent gave the crude mixture which was submitted to column chromatography on silica gel eluting with n-hexane/AcOEt (4/1) to give 7c (251 mg, 100%); mp 174-175 °C (CHCl3); 1H NMR (CDCl3): δ 9.04-8.77 (m, 1H, H-7 or -10), 8.57-8.32 (m, 2H, H-5, H-10 or -7), 7.93-7.64 (m, 2H, H-8, H-9), 5.60 (br s, 1H, OH), 4.93 (hp, 1H, J = 6.0 Hz, OCH), 4.51 (dqAB, 2H, J = 2.5, 17.0 Hz, Δδ= 0.34 ppm, CH2), 4.13 (s, 1H, CH), 2.50 (t, 1H, J = 2.5 Hz, C≡CH), 1.18 (d, 3H, J = 6.0 Hz, CH3), 0.82 (d, 3H, J = 6.0 Hz, CH3); IR (KBr, cm-1): 3330, 3290, 2123, 1746, 1708, 1688; Anal. Calcd for C23H17F6NO5: C, 55.10; H, 3.42; N, 2.79. Found: C, 55.21; H, 3.43; N, 2.67.
9. Experimental procedure for the formal dehydration of 7c into 8c: To a solution of 7c (150 mg, 0.3 mmol) and pyridine (48 mg, 0.6 mmol) in CH2Cl2 (2.4 mL) was added thionyl chloride (71 mg, 0.6 mmol) with cooling and the stirring was continued at rt for 2 h. The mixture was washed with saturated aqueous Na2CO3, with 1M HCl and then with water, and dried over Na2SO4. Evaporation of the solvent gave the practically pure product 8c (129 mg, 89%); mp 189-190 ℃ (n-hexane/AcOEt); 1H NMR (CDCl3): δ 9.00-8.76 (m, 2H, H-7, H-10), 8.53 (br s, 1H, H-5), 7.95-7.59 (m, 2H, H-8, H-9), 5.32 (hp, 1H, J = 6.0 Hz, OCH), 4.93 (d, 2H, J = 2.5 Hz, CH2), 2.68 (t, 1H, J = 2.5 Hz, C≡CH), 1.40 (d, 6H, J = 6.0 Hz, CH3); IR (KBr, cm-1): 3260, 2100, 1735, 1713, 1698, 1659; Anal. Calcd for C23H15F6NO4: C, 57.15; H, 3.13; N, 2.90. Found: C, 57.01; H, 3.22; N, 2.81.