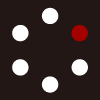
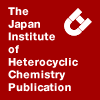
HETEROCYCLES
An International Journal for Reviews and Communications in Heterocyclic ChemistryWeb Edition ISSN: 1881-0942
Published online by The Japan Institute of Heterocyclic Chemistry
e-Journal
Full Text HTML
Received, 29th September, 2008, Accepted, 10th November, 2008, Published online, 14th November, 2008.
DOI: 10.3987/COM-08-S(D)47
■ Use of Diketopiperazines for Determining Absolute Configurations of α-Substituted Serines by 1H-NMR Spectroscopy
Shigeki Sano,* Michiyasu Nakao, Masanori Takeyasu, Syuji Kitaike, Yasuko Yoshioka, and Yoshimitsu Nagao
Molecular Medicinal Chemistry, Faculty of Pharmaceutical Sciences, The University of Tokushima, Sho-machi, Tokushima 770-8505, Japan
Abstract
A chiral α-substituted serine of interest was transformed into both of the corresponding diastereomers of diketopiperazines utilizing L- and D-phenylalanine methyl ester hydrochloride. The absolute configuration of the original chiral α-substituted serine was determined by 1H-NMR analyses of the resulting diastereomers.INTRODUCTION
Since non-proteinogenic α-substituted serine moieties were identified as key structures in various biologically active compounds, such as myriocin (ISP-I, thermozymocidin),1 sphingofungin E,2 (+)-conagenin,3 and (+)-lactacystin,4 interest in developing novel synthetic methods for α-substituted serines has intensified (Figure 1). Within this area, we developed several non-enzymatic and enzymatic asymmetric syntheses of α-substituted serines.5 However, the absolute configurations of some of these α-substituted serines could not be determined because of the difficulty of chemically converting them into known compounds. Accordingly, we examined the 1H-NMR spectroscopic behavior of both diastereomers of diketopiperazines obtained by the condensation of a chiral α-substituted N-Cbz-serine with L- and D-phenylalanine methyl ester hydrochloride. In this paper, we determine the absolute configurations of α-substituted serines utilizing 1H-NMR spectra of the structurally correlated chiral diketopiperazines.
†Dedicated to the memory of the late Professor John W. Daly.
RESULTS AND DISCUSSION
Condensation of the optically active α-substituted N-Cbz-serines 1a-d with L- and D-phenylalanine methyl ester hydrochloride [(S)- and (R)-2] using 1-ethyl-3-(3-dimethylaminopropyl)carbodiimide hydrochloride (EDC•HCl)—1-hydroxybenzotriazole (HOBt) as coupling reagents afforded the diastereomers of dipeptides 3a-d in 51-98% yields. Subsequent catalytic hydrogenolysis of 3a-d with Pd-C under hydrogen at rt, followed by heating in a sealed tube at 180-190 °C, furnished both diastereomers of diketopiperazines 4a-c,d’ in 16-43% yields without any isomerization (Scheme 1). The descriptors (S,S)- or (R,S)- were employed for 3a,b and 4a,b, since the absolute configuration of 1a,b was already determined to be S by their structural correlation to the known chiral compounds.5
Figure 2 shows selected data on the chemical shifts in the 1H-NMR (400 MHz, DMSO-d6) spectra of diketopiperazines (S,S)- and (R,S)-4a,b derived from (S)-α-substituted N-Cbz-serines (S)-1a,b. In the case of (S,S)-4a,b, the CH2 and OH protons of the hydroxymethyl group appeared at a higher magnetic field than the corresponding protons of (R,S)-4a,b due to the shielding effect of the benzyl group originated from L-phenylalanine. That is to say, the benzyl group and the hydroxy group of (S,S)-4a,b are in a cis relationship to the plane of the diketopiperazine ring. As a result, the chemical shift difference in ppm (ΔδSR) of the 4a,b hydroxy group showed large negative values. On the other hand, the CH2 protons of the benzyl group (originated from the α-benzylserine moiety) of (R,S)-4a and the CH3 protons of the methyl group of (R,S)-4b were observed at a higher magnetic field than that of (S,S)-4a,b, for the same reason. Based on the unambiguous spectroscopic results described above, we propose a plausible method (the DKP method) for determining the absolute configuration of α-substituted serines. Thus, an upfield shift of the CH2 and OH protons of the hydroxymethyl group of a diketopiperazine furnished from chiral α-substituted serine and L-phenylalanine methyl ester hydrochloride [(S)-2] suggests that the absolute configuration of the α-substituted serine is S. Whereas, an upfield shift of the protons (CH3, CH2, or CH) of the R group should be observed in the diketopiperazine obtained from the (S)-α-substituted serine with D-phenylalanine methyl ester hydrochloride [(R)-2].
Next, we attempted to determine the absolute configuration of N-Cbz-α-n-hexylserine 1c and N-Cbz-α-allylserine 1d by the DKP method. Chemical shifts in the 1H-NMR spectra of diketopiperazine 4c,d’ derived from 1c,d are shown in Figure 3. The CH2 and OH protons of the hydroxymethyl group of (S)-4c,d’ were distinctly apparent at a higher magnetic field. An upfield shift of the protons (CH3 or CH2) of the R group were also observed in the 1H-NMR spectra of diketopiperazine (R)-4c,d’. As a result, the absolute configuration of diketopiperazines 4c,d’ was found to be S,S or R,S, similar to those of 4a,b. Consequently, the absolute configuration of 1c,d was determined to be S based on the DKP method.
Compared to the conventional methods for determining the absolute configurations of chiral compounds by 1H-NMR spectroscopy, such as the modified Mosher’s method,6 extremely large differences in chemical shifts (ΔδSR values) were observed with the DKP method, as shown in Figures 2 and 3. For the most part, ΔδSR values of more than 0.2 ppm were obtained in diketopiperazines 4a-c,d’ derived form chiral α-substituted N-Cbz-serines 1a-d. In particular, the largest ΔδSR value (0.71 ppm) was obtained in the case of α-methyl serine derivative 4b. These results are attributed to the strong shielding effect of the benzyl group came from L- and D-phenylalanine methyl ester hydrochloride [(S)- and (R)-2]. The diketopiperazine probably adopts a folded conformation, as shown in Figure 4.7 Needless to say, opposite signs of ΔδSR values were obtained by the distinct differentiation of side chains of diketopiperazines, depending on the locations of their protons relative to the ring plane. The novel DKP method is expected to be further applicable for determining the absolute configurations of various α-amino acid derivatives.
In conclusion, the absolute configurations of the optically active α-substituted N-Cbz-serines 1a-d have been successfully determined by 1H-NMR analyses of both of the corresponding diastereomers of diketopiperazines 4a-c,d’ derived from L- and D-phenylalanine methyl ester hydrochloride [(S)- and (R)-2]. Further studies are under way to evaluate the practicality, efficacy, and accuracy of this novel DKP method for determining the absolute configuration of various α-amino acids.
EXPERIMENTAL
All melting points were determined on a Yanaco micro melting point apparatus and are uncorrected. IR spectra were obtained using a JASCO FT/IR-420 IR Fourier transform spectrometer. 1H-NMR (400 MHz) and 13C-NMR (100 or 75 MHz) spectra were recorded on JEOL JNM-AL400 and JEOL JNM-AL300 spectrometers, respectively. Chemical shifts are given in δ values (parts per million) using tetramethylsilane (TMS) as an internal standard. Electron spray ionization mass spectra (ESIMS) were recorded on a Waters LCT Premier spectrometer. All reactions were monitored by TLC employing 0.25-mm silica gel plates (Merck 5715; 60 F254). Column chromatography was carried out on silica gel [Kanto Chemical 60N (spherical, neutral); 63-210 μm]. Anhydrous CH2Cl2 was commercially obtained from Kanto Chemical. Triethylamine was distilled prior to use. All other reagents were used as purchased.
Typical Procedure for Preparation of Dipeptides 3
To a solution of 1a (36 mg, 0.109 mmol), triethylamine (17 μL, 0.120 mmol), and (S)-2 (26 mg, 0.120 mmol) in anhydrous CH2Cl2 (3 mL) were added HOBt (18 mg, 0.131 mmol) and EDC•HCl (23 mg, 0.120 mmol) at 0 °C under argon. After being stirred at 0 °C for 15 min, the reaction mixture was allowed to warm to rt and then was stirred for 16 h. The reaction mixture was treated with 1 N HCl (2 mL) and then extracted with CHCl3 (5 mL x 3). The extract was dried over anhydrous MgSO4, filtered, and concentrated in vacuo. The residue was purified by silica gel column chromatography [n-hexane—AcOEt (1:1)] to afford (S,S)-3a (53 mg, 98%) as a white solid.
Typical Procedure for Preparation of Diketopiperazine 4
The mixture of (S,S)-3a (36 mg, 0.0734 mmol) and 10% Pd–C (3.9 mg, 0.00367 mmol) in MeOH (2 mL) was stirred at rt for 7 h under hydrogen. The reaction mixture was filtered and concentrated in vacuo. The residue was dissolved in MeOH (2 mL) and heated at 190 °C in a sealed tube for 24 h. After cooling to rt, the reaction mixture was concentrated in vacuo and washed with MeOH to furnish (S,S)-4a (10 mg, 42%) as a white solid.
(3S,6S)-3,6-Dibenzyl-3-(hydroxymethyl)piperazine-2,5-dione [(S,S)-4a] White solid; mp >245 °C (dec); [α]D28 –30.3° (c 0.05, DMSO); 1H-NMR (400 MHz, DMSO-d6) δ 2.59-2.68 (m, 1H), 2.74-2.82 (m, 1H), 2.87-2.98 (m, 2H), 3.12-3.30 (m, 3H), 4.93-5.01 (m, 1H), 7.32-7.04 (m, 10H), 7.82 (s, 1H), 7.91 (s, 1H); 13C-NMR (75 MHz, DMSO-d6) δ 54.5, 64.7, 67.0, 126.2, 126.5, 127.7, 127.8, 129.7, 130.3, 135.8, 136.4, 166.5, 167.4; IR (KBr) 2358, 1675, 1454 cm–1; ESIMS calcd for C19H20N2NaO3 MW 347.1372, found m/z 347.1378 (M+ + Na).
(3S,6S)-6-Benzyl-3-(hydroxymethyl)-3-methylpiperazine-2,5-dione [(S,S)-4b] White solid; mp >240 °C (dec); [α]D27 –50.5° (c 0.06, DMSO); 1H-NMR (400 MHz, DMSO-d6) δ 1.17 (s, 3H), 3.00-3.14 (m, 4H), 4.04-4.12 (m, 1H), 4.98-5.04 (m, 1H), 7.15-7.32 (m, 5H), 7.79 (s, 1H), 7.86 (s, 1H); 13C-NMR (75 MHz, DMSO-d6) δ 22.6, 40.1, 55.7, 59.7, 67.3, 126.3, 128.0, 129.7, 136.9, 166.6, 167.0; IR (KBr) 2360, 1664, 1454 cm–1; ESIMS calcd for C13H16N2NaO3 MW 271.1059, found m/z 271.1046 (M+ + Na).
(3S,6S)-6-Benzyl-3-hexyl-3-(hydroxymethyl)piperazine-2,5-dione [(S,S)-4c] White solid; mp 213-216 °C; [α]D28 –32.7° (c 0.81, DMSO); 1H-NMR (400 MHz, DMSO-d6) δ 0.72-0.93 (m, 3H), 0.95-1.41 (m, 9H), 1.52-1.68 (m, 1H), 2.95-3.14 (m, 4H), 4.02-4.12 (m, 1H), 4.85-4.93 (m, 1H), 7.08-7.37 (m, 5H), 7.75 (s, 1H), 7.85 (s, 1H); 13C-NMR (75 MHz, DMSO-d6) δ 13.8, 21.9, 22.8, 28.5, 31.0, 34.5, 55.5, 63.5, 67.3, 126.3, 128.0, 129.7, 136.7, 166.9, 168.0; IR (KBr) 2358, 1671, 1455 cm–1; ESIMS calcd for C18H26N2NaO3 MW 341.1841, found m/z 341.1824 (M+ + Na).
(3S,6S)-6-Benzyl-3-(hydroxymethyl)-3-propylpiperazine-2,5-dione [(S,S)-4d’] White solid; mp >251 °C (dec); [α]D27 –43.1° (c 0.04, DMSO); 1H-NMR (400 MHz, DMSO-d6) δ 0.77-0.86 (m, 3H), 1.02-1.14 (m, 1H), 1.20-1.38 (m, 2H), 1.51-1.63 (m, 1H), 2.98-3.12 (m, 4H), 4.04-4.10 (m, 1H), 4.85-4.91 (m, 1H), 7.16-7.33 (m, 5H), 7.74 (s, 1H), 7.85 (s, 1H); 13C-NMR (75 MHz, DMSO-d6) δ 13.8, 16.3, 36.7, 55.5, 63.5, 67.2, 126.3, 128.0, 129.7, 136.7, 166.9, 168.0; IR (KBr) 1673, 1454 cm–1; ESIMS calcd for C15H20N2NaO3 MW 299.1372, found m/z 299.1373 (M+ + Na).
(3S,6R)-3,6-Dibenzyl-3-(hydroxymethyl)piperazine-2,5-dione [(R,S)-4a] White solid; mp >263 °C (dec); [α]D28 +67.4° (c 0.05, DMSO); 1H-NMR (400 MHz, DMSO-d6) δ 2.03 (m, 1H), 2.41-2.59 (m, 2H), 2.80-2.96 (m, 1H), 3.32-3.39 (m, 1H), 3.70-3.80 (m, 1H), 3.89-3.97 (m, 1H), 5.22-5.30 (m, 1H), 6.85-6.96 (m, 2H), 7.04-7.34 (m, 8H), 7.69 (s, 1H), 7.94 (s, 1H); 13C-NMR (75 MHz, DMSO-d6) δ 55.1, 65.2, 68.5, 126.1, 126.5, 127.97, 128.01, 129.4, 130.4, 135.9, 136.8, 167.4, 167.9; IR (KBr) 1666, 1444 cm–1; ESIMS calcd for C19H20N2NaO3 MW 347.1372, found m/z 347.1376 (M+ + Na).
(3S,6R)-6-Benzyl-3-(hydroxymethyl)-3-methylpiperazine-2,5-dione [(R,S)-4b] White solid; mp >272 °C (dec); [α]D27 –7.6° (c 0.09, DMSO); 1H-NMR (400 MHz, DMSO-d6) δ 0.46 (s, 3H), 2.86 (dd, J = 4.9, 13.4 Hz, 1H), 3.06 (dd, J = 4.4, 10.1 Hz, 1H), 3.15 (dd, J = 4.9, 13.9 Hz, 1H), 3.46 (dd, J = 5.4, 10.0 Hz, 1H), 4.14-4.20 (m, 1H), 5.03-5.10 (m, 1H), 7.14-7.30 (m, 5H), 7.80 (s, 1H), 8.03 (s, 1H); 13C-NMR (75 MHz, DMSO-d6) δ 21.7, 37.9, 55.3, 59.9, 67.9, 126.4, 127.8, 130.2, 136.1, 166.4, 168.9; IR (KBr) 1673, 1463 cm–1; ESIMS calcd for C13H16N2NaO3 MW 271.1059, found m/z 271.1046 (M+ + Na).
(3S,6R)-6-Benzyl-3-hexyl-3-(hydroxymethyl)piperazine-2,5-dione [(R,S)-4c] White solid; mp 274-276 °C; [α]D27 +19.5° (c 0.04, DMSO); 1H-NMR (400 MHz, DMSO-d6) δ 0.16-0.45 (m, 2H), 0.74-1.04 (m, 8H), 1.06-1.19 (m, 2H), 1.22-1.33 (m, 1H), 2.81 (dd, J = 4.6, 13.2 Hz, 1H), 3.05 (dd, J = 4.9, 10.0 Hz, 1H), 3.21 (dd, J = 2.2, 13.4 Hz, 1H), 3.46 (dd, J = 5.6, 10.0 Hz, 1H), 4.12-4.21 (m, 1H), 5.08-5.17 (m, 1H), 7.12-7.28 (m, 5H), 7.69 (s, 1H), 8.08 (s, 1H); 13C-NMR (75 MHz, DMSO-d6) δ 13.8, 21.4, 21.8, 28.5, 30.9, 33.0, 36.9, 54.9, 64.1, 68.3, 126.3, 127.5, 130.3, 136.0, 167.1, 168.4; IR (KBr) 1668, 1455 cm–1; ESIMS calcd for C18H26N2NaO3 MW 341.1841, found m/z 341.1824 (M+ + Na).
(3S,6R)-6-Benzyl-3-(hydroxymethyl)-3-propylpiperazine-2,5-dione [(R,S)-4d’] White solid; mp >280 °C (dec); [α]D27 +17.0° (c 0.11, DMSO); 1H-NMR (400 MHz, DMSO-d6) δ 0.17-0.43 (m, 2H), 0.45-0.54 (m, 3H), 0.81-0.93 (m, 1H), 1.19-1.28 (m, 1H), 2.82 (dd, J = 4.6, 13.2 Hz, 1H), 3.05 (dd, J = 4.9, 10.3 Hz, 1H), 3.21 (dd, J = 2.9, 13.2 Hz, 1H), 3.46 (dd, J = 5.6, 10.3 Hz, 1H), 4.14-4.20 (m, 1H), 5.07-5.13 (m, 1H), 7.12-7.32 (m, 5H), 7.62 (s, 1H), 8.08 (s, 1H); 13C-NMR (75 MHz, DMSO-d6) δ 13.8, 14.9, 35.2, 37.1, 55.0, 64.1, 68.2, 126.2, 127.6, 130.4, 136.1, 167.0, 168.2; IR (KBr) 1670, 1455 cm–1; ESIMS calcd for C15H20N2NaO3 MW 299.1372, found m/z 299.1368 (M+ + Na).
References
1. G. Just and D. R. Payette, Tetrahedron Lett., 1980, 21, 3219; CrossRef L. Banfí, M. G. Beretta, L. Colombo, C. Gennari, and C. Scolastico, J. Chem. Soc., Perkin Trans. 1, 1983, 1613; CrossRef M. Yoshikawa, Y. Yokokawa, Y. Okuno, N. Yagi, and N. Murakami, Chem. Pharm. Bull., 1994, 42, 2662; M. Yoshikawa, Y. Yokokawa, Y. Okuno, and N. Murakami, Tetrahedron, 1995, 51, 6209; CrossRef S. Sano, Y. Kobayashi, T. Kondo, M. Takebayashi, S. Maruyama, T. Fujita, and Y. Nagao, Tetrahedron Lett., 1995, 36, 2097; CrossRef S. Hatakeyama, M. Yoshida, T. Esumi, Y. Iwabuchi, H. Irie, T. Kawamoto, H. Yamada, and M. Nishizawa, Tetrahedron Lett., 1997, 38, 7887; CrossRef A. V. R. Rao, M. K. Gurjar, T. R. Devi, and K. R. Kumar, Tetrahedron Lett., 1993, 34, 1653; CrossRef S. Deloisy, T. T. Thang, A. Olesker, and G. Lukacs, Tetrahedron Lett., 1994, 35, 4783; CrossRef T. Oishi, K. Ando, K. Inomiya, H. Sato, M. Iida, and N. Chida, Bull. Chem. Soc. Jpn., 2002, 75, 1927; CrossRef J. Gonda, M. Martinková, J. Raschmanová, and E. Balentová, Tetrahedron Lett., 2006, 17, 1875.
2. S. Kobayashi, T. Hayashi, S. Iwamoto, T. Furuta, and M. Matsumura, Synlett, 1996, 672; CrossRef S. Kobayashi, M. Matsumura, T. Furuta, T. Hayashi, and S. Iwamoto, Synlett, 1997, 301; CrossRef S. Kobayashi, T. Furuta, T. Hayashi, M. Nishijima, and K. Hanada, J. Am. Chem. Soc., 1998, 120, 908; CrossRef S. Kobayashi and T. Furuta, Tetrahedron, 1998, 54, 10275; CrossRef B. Wang, X. Yu, and G. Lin, Synlett, 2001, 904; CrossRef T. Oishi, K. Ando, K. Inomiya, H. Sato, M. Iida, and N. Chida, Org. Lett., 2002, 4, 151. CrossRef
3. S. Hatakeyama, H. Fukuyama, Y. Mukugi, and H. Irie, Tetrahedron Lett., 1996, 37, 4047; CrossRef S. Sano, T. Miwa, K. Hayashi, K. Nozaki, Y. Ozaki, and Y. Nagao, Tetrahedron Lett., 2001, 42, 4029; CrossRef Y. Matsukawa, M. Isobe, H. Kotsuki, and Y. Ichikawa, J. Org. Chem., 2005, 70, 5339; CrossRef T. K. Chakraborty and G. Sudhakar, Tetrahedron Lett., 2006, 47, 5847; CrossRef T. Yakura, Y. Yoshimoto, C. Ishikawa, and S. Mabuchi, Tetrahedron, 2007, 63, 4429. CrossRef
4. H. Uno, J. E. Baldwin, and A. T. Russell, J. Am. Chem. Soc., 1994, 116, 2139; CrossRef N. Chida, J. Takeoka, N. Tsutsumi, and S. Ogawa, J. Chem. Soc., Chem. Commun., 1995, 793; CrossRef T. Nagamitsu, T. Sunazuka, H. Tanaka, S. Omura, P. A. Sprengeler, and A. B. Smith, III, J. Am. Chem. Soc., 1996, 118, 3584; CrossRef E. J. Corey, W. Li, and T. Nagamitsu, Angew. Chem. Int. Ed., 1998, 37, 1676; CrossRef J. S. Panek and C. E. Masse, Angew. Chem. Int. Ed., 1999, 38, 1093; CrossRef H. Ooi, N. Ishibashi, Y. Iwabuchi, J. Ishihara, and S. Hatakeyama, J. Org. Chem., 2004, 69, 7765; CrossRef N. Fukuda, K. Sasaki, T. V. R. S. Sastry, M. Kanai, and M. Shibasaki, J. Org. Chem., 2006, 71, 1220; CrossRef E. P. Balskus and E. N. Jacobsen, J. Am. Chem. Soc., 2006, 128, 6810. CrossRef
5. S. Sano, X.-K. Liu, M. Takebayashi, Y. Kobayashi, K. Tabata, M. Shiro, and Y. Nagao, Tetrahedron Lett., 1995, 36, 4101; CrossRef S. Sano, M. Takebayashi, T. Miwa, T. Ishii, and Y. Nagao, Tetrahedron: Asymmetry, 1998, 9, 3611; CrossRef S. Sano, T. Miwa, X.-K. Liu, T. Ishii, T. Takehisa, M. Shiro, and Y. Nagao, Tetrahedron: Asymmetry, 1998, 9, 3615; CrossRef S. Sano, K. Hayashi, T. Miwa, T. Ishii, M. Fujii, H. Miwa, and Y. Nagao, Tetrahedron Lett., 1998, 39, 5571; CrossRef S. Sano, T. Ishii, T. Miwa, and Y. Nagao, Tetrahedron Lett., 1999, 40, 3013; CrossRef S. Sano, M. Nakao, M. Takeyasu, T. Honjo, and Y. Nagao, Lett. Org. Chem., 2006, 3, 764. CrossRef
6. J. A. Dale and H. S. Mosher, J. Am. Chem. Soc., 1973, 95, 512; CrossRef B. M. Trost, J. L. Belletire, S. Godleski, P. G. McDougal, J. M. Balkovec, J. J. Baldwin, M. E. Christy, G. S. Ponticello, S. L. Varga, and J. P. Springer, J. Org. Chem., 1986, 51, 2370; CrossRef I. Ohtani, T. Kusumi, Y. Kashman, and H. Kakisawa, J. Am. Chem. Soc., 1991, 113, 4092; CrossRef J. M. Seco, Sh. K. Latypov, E. Quiñoá, and R. Riguera, Tetrahedron Lett., 1994, 35, 2921; CrossRef R. Chinchilla, L. R. Falvello, and C. Nájera, J. Org. Chem., 1996, 61, 7285; CrossRef J. M. Seco, E. Quiñoá, and R. Riguera, J. Org. Chem., 1999, 64, 4669; CrossRef T. Takahashi, A. Fukuishima, Y. Tanaka, Y. Takeuchi, K. Kabuto, and C. Kabuto, Chem. Commun., 2000, 787; CrossRef M. J. Ferreiro, Sh. K. Latypov, E. Quiñoá, and R. Riguera, J. Org. Chem., 2000, 65, 2658. CrossRef
7. K. D. Kopple and D. H. Marr, J. Am. Chem. Soc., 1967, 89, 6193; CrossRef L. J. Williams, B. Jagadish, M. G. Lansdown, M. D. Carducci, and E. A. Mash, Tetrahedron, 1999, 55, 14301; CrossRef M. Isaka, S. Palasarn, P. Rachtawee, S. Vimuttipong, and P. Kongsaeree,, Org. Lett., 2005, 7, 2257; CrossRef C. Becker, C. Hoben, D. Schollmeyer, G. Scherr, and H. Kunz, Eur. J. Org. Chem., 2005, 1497; CrossRef M. Tullberg, M. Grφtli, and K. Luthman, Tetrahedron, 2006, 62, 7484. CrossRef