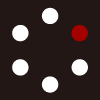
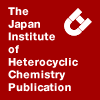
HETEROCYCLES
An International Journal for Reviews and Communications in Heterocyclic ChemistryWeb Edition ISSN: 1881-0942
Published online by The Japan Institute of Heterocyclic Chemistry
e-Journal
Full Text HTML
Received, 30th September, 2008, Accepted, 17th November, 2008, Published online, 20th November, 2008.
DOI: 10.3987/COM-08-S(D)49
■ Total Synthesis of (–)-Flustramine B via One-Pot Intramolecular Ullmann Coupling and Claisen Rearrangement
Tomohiro Hirano, Kanako Iwakiri, Hiroshi Miyamoto, Atsuo Nakazaki, and Susumu Kobayashi*
Faculty of Pharmaceutical Sciences, Science University of Tokyo, 2641 Yamazaki, Noda, Chiba 278-8510, Japan
Abstract
Total synthesis of (–)-flustramine B was achieved via one-pot intramolecular Ullmann coupling and Claisen rearrangement. A striking feature of this method of synthesis is that the sequential intramolecular Ullmann coupling and Claisen rearrangement reactions proceeds with concomitant deprotection of the methoxymethyl (MOM) group to afford spirocyclic oxindole with perfect asymmetric transmission in good overall yield.INTRODUCTION
(–)-Flustramine B (1), isolated from the marine bryozoan flustra foliacea,1 is an example of pyrrolidinoindoline alkaloids with a prenyl group at the C-3a quaternary carbon center (Figure 1). Although (–)-1 has a simple scaffold structure, it displays interesting biological activity; for example, it exhibits muscle-relaxant activity both in vivo and in vitro.2 Some natural compounds related to (–)-1, such as debromoflustramine B1c and pseudophrynaminol,3 have been reported to show butyrylcholinesterase inhibition activity4 and an antibacterial activity,5 respectively. Because of their structural features and the important biological activities exhibited by them, pyrrolidinoindoline alkaloids have attracted considerable attention, and a number of studies on the total synthesis of a series of pyrrolidinoindoline alkaloids have been reported.6
Recently, we developed an efficient approach for the synthesis of spirocyclic oxindoles via one-pot intramolecular Ullmann coupling and Claisen rearrangement (Scheme 1).7 This sequential reaction proceeds in the following two steps: (1) intramolecular Ullmann coupling of 2-iodoindole (A) bearing an allyl alcohol moiety in the presence of catalytic amount of CuCl and 2-aminopyridine in a 2-equiv solution of NaOMe to yield a pyranoindole intermediate (B) and (2) subsequent thermal Claisen rearrangement of B to afford spirocyclic oxindole (C). In order to demonstrate the synthetic utility of the obtained spirocyclic oxindole, we used it in the total synthesis of racemic debromoflustramine B.6e In this paper, we describe the asymmetric total synthesis of flustramine B by carrying out our previously developed method using enantioenriched 2-iodoindole bearing a 6-bromo group.
RESULTS AND DISCUSSION
The synthetic strategy of (–)-flustramine B (1) is depicted in Scheme 2. According to literature,6a (–)-1 can be synthesized from the known amide 16, which in turn can be derived from keto carboxylic acid 12 using conventional transformations. Enantioenriched spirocyclic oxindole 11 could be a potential precursor of keto carboxylic acid 12 after oxidative cleavage. We believe that the asymmetryic quaternary carbon center located at C-3a in 11 could be controlled by selecting one-pot intramolecular Ullmann coupling and Claisen rearrangement as the key reactions, which causes an asymmetric transmission of the tertiary alcohol of 2-iodoindole 10. However, the Ullmann coupling of 10 may occur intermolecularly because of the presence of the aryl bromide in 10, which is a potential reactive site; this is different from the structure of the debromo analogue used in the synthesis of debromoflustramine B.6e The deprotection of the MOM group in oxindoles should be carried out at a particular step once the one-pot intramolecular Ullmann coupling and Claisen rearrangement are complete.8 The iodo-desilylation of 9 should be carried out in a mild medium because of the acid-labile nature of the tertiary allylic alcohol present in its side-chain. The 2-silylindole 9 can be synthesized by the Larock indole synthesis9 of monobrominated o-iodoaniline 4 and silyl acetylene 7, which possesses an indispensable chiral center and is a derivative of (–)-linalool. o-Iodoaniline 4 can be prepared from commercially available o-nitroaniline.
The synthetic route to enantioenriched spirocyclic oxindole 11 is summarized in Scheme 3. We first prepared the iodoaniline derivative 4 from o-nitroaniline. Regioselective monobromination of o-nitroaniline was achieved using aq. HBr and aq. H2O2 in MeOH, which afforded 2 in 75% yield.10 The bromoaniline 2 was then converted into iodonitrobenzene 3 through a diazoarene under conventional conditions.11 Refluxing of 3 with Fe in AcOH-EtOH led to the formation of the desired brominated iodoaniline 4 in excellent yield.12
Silyl acetylene 7 bearing a chiral center, which is the other reactant required for the Larock indole synthesis, was prepared from (–)-linalool by the following three steps. First, the ozonolysis of (–)-linalool (98% ee) at –78 °C in CH2Cl2 and pyridine and then the addition of Me2S gave lactol 5 in moderate yield.13 Lactol 5 was then treated with Ohira-Bestmann reagent 1814 to produce hydroxyacetylene 6 in 71% yield. Finally, the hydroxy group and acetylene terminus in 6 were protected by TMS groups, and the distillation of 6 (67 °C/3 mmHg) afforded the desired silyl acetylene 7 in 92% yield.
The Larock indole synthesis using iodoaniline derivative 4 and silyl acetylene 7 was performed under typical conditions (LiCl, K2CO3, PPh3, Pd(OAc)2 in DMF at 100 °C) to afford the corresponding 2-silylindole 9 in 57% yield. The resulting silyl indole 8 was protected by a MOM group and its iodo-desilylation was then examined. A solution of 1.2 equiv of ICl in CH2Cl2 was carefully added to silyl indole 9 at –78 °C, which resulted in the formation of the dispensable 2-iodoindole 10 in moderate yield (59%) after carrying out the deprotection of the TMS group on the tertiary alcohol. In contrast, when 8 was treated with a solution of 1.05 equiv of NIS in CH2Cl2 at rt and warmed in a gentle reflux, the yield of 10 improved significantly (94% yield of the two steps). 2-Iodoindole 10 was then subjected to the key transformation reactions under the conditions specified by in our previous study to give the corresponding spirocyclic oxindole 11 in 69% yield; deiodinated indole was also produced in trace amounts (6%). The formation of the corresponding intermolecular Ullmann adduct, 6-methoxy spirocyclic oxindole, was not observed. Interestingly, this reaction involves not only the expected intramolecular Ullmann coupling and Claisen rearrangement but also the deprotection of the MOM group under basic conditions.15 The chiral HPLC analysis of the known debromo spirocyclic oxindole7a derived from 11 revealed that 11 had an enantiomeric excess of 98%. This enantiomeric excess of the product indicates that both intramolecular Ullmann coupling and Claisen rearrangement reactions were stereospecific through a boat-like transition, as shown in Scheme 4.
Scheme 5 summarizes the final transformation of the obtained spirocyclic oxindole 11 into (–)-flustramine B (1) by our previously reported method6e but with a few modifications. The oxidative cleavage of the cyclohexene ring in spirocyclic oxindole 11 occurred smoothly in the presence of a catalytic amount of OsO4 with N-methyl morpholine N-oxide (NMO) as the co-oxidant; the resulting diol was treated with NaIO4 to give ketoaldehyde. The addition of ketoaldehyde to a buffered solution of NaClO216 afforded keto carboxylic acid 12 in good overall yield (84% from 11). The ketone carbonyl group in 12 was converted into an exo-olefin by using the Wittig reaction in excess amount of triphenylphosphonium methylide. The resulting alkene was then subjected to acid-catalyzed isomerization using H2SO4 and MgSO4 to obtain carboxylic acid 14 bearing the desired prenyl group at C-3a. Prenylations of this carboxylic acid and amide resulted in the formation of the corresponding ester 15 having three prenyl groups in 18% yield in three steps. Treatment of the prenyl ester 15 with aq. MeNH2 in MeOH gave N-methyl amide 16 in 67% yield.
To complete the synthesis of 1 from N-methyl amide 16, we used the method reported by Kawasaki and co-workers.6a First, we attempted the direct conversion of 16 to 1 using 5 equiv of AlH3·EtNMe2 between –15 °C and rt. However, this resulted in the formation of a mixture of amide 16 and desired 1.17 Thus, the following reductions were carried out stepwise. The addition of amide 16 to 5 equiv of AlH3·EtNMe2 at –20 °C afforded (–)-flustramide B (17) in 96% yield as a single diastereomer. The resultant 17 was treated with 1.5 equiv of AlH3·EtNMe2 at rt to reduce the unreacted lactum carbonyl group, and (–)-flustramine B (1) was obtained in 93% yield. The 1H and 13C NMR spectra and the specific rotation of 1 synthesized by our method were consistent with those of the corresponding naturally occurring compounds1c and of that synthesized by a previous method.6a
In conclusion, we have achieved total synthesis of (–)-flustramine B via sequential intramolecular Ullmann coupling and Claisen rearrangement, which spirocyclic oxindole without a loss of its stereochemical integrity. This approach offers a potentially useful synthetic route to pyrrolidinoindoline alkaloids bearing a prenyl group at C-3a.
EXPERIMENTAL
General Techniques. 1H and 13C NMR spectra were recorded on a JEOL JNM-LD400 spectrometer operating at either 400 MHz (1H) or 100 MHz (13C). Chemical shifts are reported in δ units and are referenced to the solvent, i.e., 7.26/77.1 for CDCl3. Multiplicities are indicated as: br (broadened), s (singlet), d (doublet), t (triplet), q (quartet), quint (quintet), sept (septet), or m (multiplet). Coupling constants (J) are reported in Hertz (Hz). Infrared spectra were recorded on a Jasco FT-IR410 spectrometer. Melting points were recorded on a Yanaco MP-3S. Electrospray ionization mass spectra were recorded on an Applied Biosystems API QSTAR pulsar i as high resolution, using poly(ethylene glycol) as internal standard. Thin-layer chromatography (TLC) was performed on silica gel 60 F254 (Merck 1.05715.0009) plates. Flash column chromatography was performed on a PSQ100B silica gel (Fuji Silysia Co., Ltd., Japan) or on a Silica gel 60 N (63-210 μm; Kanto Chemical Co., Inc., Japan) and PSQ100B was usually used. THF and Et2O were purchased from Wako Pure Chemical Industries Ltd. in anhydrous grade. CH2Cl2 was distilled from CaH2 immediately before use. Diisopropylethylamine, pyridine, and triethylamine were distilled from CaH2 and stored over KOH. Toluene and DMF were distilled from CaH2 and stored over activated MS 4A. Triglyme was distilled from LiAlH4 and stored over activated MS 4A. All moisture sensitive reactions were performed under a static argon atmosphere in oven-dried of flame-dried glassware.
4-Bromo-2-nitroaniline (2). This compound was synthesized according to literature.10 To a solution of o-nitroaniline (6.2 g, 45 mmol) in MeOH (92 mL) was cooled to 0 °C and 47% aq. HBr (15.5 g, 90 mmol) was added slowly, and 30% aq. H2O2 (5.1 mL, 45 mmol) was added dropwise. The reaction mixture was left at rt for 21 h. The resulting mixture was concentrated under reduced pressure. The residue was added water and then extracted three times with AcOEt. The combined organic layer was washed brine, dried over Na2SO4, filtered, and concentrated under reduced pressure. The residue was purified two times by silica gel column chromatography (hexane/AcOEt = 9:1) to afford 2 (7.34 g, 75% yield) as an orange solid. Rf 0.42 (hexane/AcOEt = 2:1); 1H NMR (400 MHz, CDCl3) δ 8.28 (d, J = 2.2 Hz, 1H), 7.43 (dd, J = 8.8, 2.2 Hz, 1H), 6.73 (d, J = 8.8 Hz, 1H), 6.08 (br, 2H); 13C NMR (100 MHz, CDCl3) δ 143.6, 138.5, 132.4, 128.3, 120.4, 107.8.
4-Bromo-1-iodo-2-nitrobenzene (3). This compound was synthesized according to literature with a slight modification.11 BF3·OEt2 (16 mL, 126 mmol) was cooled –30 °C, and added dropwise a solution of 2 in dry THF (100 mL). The mixture was stirred for 10 min and added to dropwise a solution of t-BuONO (13 mL, 111 mmol) in THF (80 mL). The reaction mixture was allowed to warm to −10 °C at which time 160 mL of diethyl ether was added and mixture was allowed to stir at rt for 1 h until a pale solid precipitated. The solid was filtered and washed with ether to afford a pale solid which was then slowly added KI (7.1 g, 42.6 mmol), I2 (5.4 g, 21.3 mmol) and MeCN (120 mL). The reaction mixture was stirred at rt for 1 h 15 min. After addition of a saturated aqueous solution of Na2S2O3 (250 mL), the mixture was extracted two times with CH2Cl2. The combined organic layer was dried over Na2SO4, filtered, and concentrated under reduced pressure to afford 3 (8.61 g, 88% yield for the two steps) as a yellow solid. Rf 0.78 (hexane/AcOEt = 2:1); 1H NMR (400 MHz, CDCl3) δ 8.00 (d, J = 2.2, 1H), 7.89 (d, J = 8.3, 1H), 7.40 (dd, J = 8.5, 2.2 Hz, 1H); 13C NMR (100 MHz, CDCl3), δ 153.4, 142.9, 136.4, 128.4, 122.6, 84.3.
5-Bromo-2-iodoaniline (4). This compound was synthesized according to literature with a slight modification.12 To a mixture of 3 (8.36 g, 25.3 mmol) and Fe (6.13 g, 108 mmol) in AcOH (45 mL) and EtOH (45 mL) was refluxed for 1 h 15 min. The mixture was cooled to rt. After addition of a saturated aqueous solution of NaHCO3 (200 mL), the mixture was extracted four times with Et2O. The combined organic layer was dried over Na2SO4, filtered, and concentrated under reduced pressure. The residue was purified by silica gel column chromatography (hexane/AcOEt = 19:1) to afford 4 (6.89 g, 91% yield) as a cream solid. Rf 0.57 (hexane/AcOEt = 4:1); 1H NMR (400 MHz, CDCl3) δ 7.45 (d, J = 8.3 Hz, 1H), 6.88 (d, J = 2.2 Hz, 1H), 6.60 (dd, J = 8.3, 2.2 Hz, 1H), 4.14 (br, 2H); 13C NMR (100 MHz, CDCl3) δ 147.9, 139.8, 123.0, 122.7, 117.0, 81.9.
Lactol 5. This reaction was carried out according to literatures.13 A solution of (R)-(−)-linalool (11.8 mL, 66.1 mmol, 98% ee) in CH2Cl2 (540 mL) and pyridine (16 mL) was stirred and cooled to −78 °C. Ozone was bubbled through the reaction mixture for 6 h at that temperature. After purging with O2 and addition of Me2S (14.6 mL), the solution was allowed to warm slowly to rt overnight. The resulting mixture was washed successively with brine, aq. CuSO4, brine, and water. And then organic layer was dried over Na2SO4, filtered, and concentrated under reduced pressure. The residue purified by silica gel chromatography (hexane/AcOEt = 90:10 → 85/15 → 75/25) to afford lactol 5 (5.56 g, 66% yield) as a light yellow oil. Rf 0.29 (hexane/AcOEt = 3:1).
(R)-3-Methyl-1-hepten-6-yn-3-ol (6). To a suspension of lactol 5 and K2CO3 (62.3 g, 451 mmol) in MeOH (700 mL) was added a solution of diazophosphonate (32.1 g, 167 mmol) in MeOH (50 mL) via a cannula, and then the reaction mixture was stirred at rt for 33 h. The resulting mixture was filtrated, and concentrated under reduced pressure. The residue was diluted with brine. The mixture was extracted with ether. The combined organic layer was dried over MgSO4, filtered, and concentrated under reduce pressure. The residue purified by fractional distillation (53 °C/6 mmHg) to afford 6 (9.7 g, 71% yield) as a colorless oil. Rf 0.47 (hexane/AcOEt = 3:1); 1H NMR (400 MHz, CDCl3) δ 5.87 (dd, J = 17.4, 10.8 Hz, 1H), 5.24 (dd, J = 17.4, 1.2 Hz, 1H), 5.09 (dd, J = 10.8, 1.2 Hz, 1H), 2.33-2.19 (m, 3H), 1.98 (t, J = 2.7 Hz, 1H), 1.83 (ddd, J = 13.8, 9.0, 7.1 Hz, 1H), 1.76 (ddd, J = 13.8, 9.3, 6.1 Hz, 1H), 1.29 (s, 3H); 13C NMR (100 MHz, CDCl3) δ 143.9, 112.4, 84.6, 72.8, 68.5, 40.3, 27.8, 13.2; IR (neat, cm<συπ>−</συπ>1) 3420, 2118, 1638; HRMS (EI) calcd for C8H12O: 124.0888, found: 124.0882; [α]D24 −2.47 (c 1.14, CHCl3).
Silylacetylene 7. To a solution of 6 (14.7 g, 118 mmol) in dry THF (237 mL) was added dropwise n-BuLi (2.66 M in hexane, 102 mL, 272 mmol) at −78 °C. After the mixture was stirred for 1 h at that temperature, TMSCl (34.5 mL, 272 mmol) was then added dropwise. The reaction mixture was stirred for 3 h at −78 °C, and then allowed to warm slowly to rt over 9 h. The mixture was added to saturated aqueous NaHCO3, the resulting mixture was extracted with hexane. The organic layer was dried over MgSO4, filtered, and concentrated under reduced pressure. The residue purified by fractional distillation (67 °C/3 mmHg) to afford 7 (29.2 g, 92% yield) as a colorless oil. Rf 0.89 (hexane/AcOEt = 10:1); 1H NMR (400 MHz, CDCl3) δ 5.80 (dd, J = 17.3, 10.7 Hz, 1H), 5.12 (dd, J = 17.3, 1.5 Hz, 1H), 5.01 (dd, J = 10.7, 1.5 Hz, 1H), 2.27 (ddd, J = 16.7, 9.8, 6.4 Hz, 1H), 2.20 (ddd, J = 16.7, 9.5, 6.6 Hz, 1H), 1.79-1.68 (m, 2H), 1.31 (s, 3H), 0.134 (s, 9H), 0.106 (s, 9H); 13C NMR (100 MHz, CDCl3) δ 144.4, 112.3, 108.0, 83.6, 75.1, 42.3, 27.1, 14.6, 2.25, 0.01; IR (neat, cm<συπ>−</συπ>1) 2176, 1251, 841; HRMS (EI) calcd for C14H28OSi2: 268.1679, found: 268.1684; [α]D23 −4.04 (c 1.57, CHCl3).
2-Silylindole 8. To a solution of o-iodoaniline 4 (8.31 g, 27.9 mmol) and silylacetylene 7 (12 g, 44.7 mmol) in DMF (140 mL) was successively added dry LiCl (1.18 g, 27.9 mmol) and K2CO3 (9.63 g, 69.9 mmol), PPh3 (366 mg, 1.39 mmol), and Pd(OAc)2 (341 mg, 1.39 mmol). Argon was bubbled through the resulting mixture for 15 min at rt, and then the reaction mixture was stirred vigorously at 100 °C for 8.5 h. After cooling to rt, the resulting mixture was filtered through a pad of Celite, and filtrate was concentrated under reduced pressure. The residue was added a saturated aqueous solution of NH4Cl and H2O. The mixture was extracted with hexane/AcOEt = 1:1 solvent, and organic layer was washed two times with H2O, washed with brine, dried over Na2SO4, filtered, and concentrated under reduced pressure. The residue was purified by silica gel chromatography (hexane/AcOEt = 20:1; hexane/diisopropyl ether = 50:1; hexane/toluene = 20:1) to afford 8 (6.93 g, 57% yield) as a light yellow solid. mp 60-61 °C; Rf 0.54 (hexane/AcOEt = 6:1); 1H NMR (400 MHz, CDCl3) δ 7.79 (br s, 1H), 7.48 (d, J = 1.7 Hz, 1H), 7.40 (d, J = 8.3 Hz, 1H), 7.16 (dd, J = 8.4, 1.6 Hz, 1H), 5.99 (dd, J = 17.2, 10.6 Hz, 1H), 5.21 (dd, J = 17.3, 1.7 Hz, 1H), 5.09 (dd, J = 10.7, 1.5 Hz, 1H), 2.90-2.75 (m, 2H), 1.80-1.71 (m, 2H), 1.42 (s, 3H), 0.30 (s, 9H), 0.16 (s, 9H) 13C NMR (100 MHz, CDCl3) δ −0.7 (CH3), 2.6 (CH3), 20.3 (CH2), 26.9 (CH3), 45.9 (CH2), 75.8 (C), 112.2 (CH2), 113.7 (CH), 115.8 (C), 120.0 (CH), 122.2 (CH), 125.9 (C), 127.7 (C), 133.7 (C), 139.0 (C), 145.3 (CH); IR (KBr, cm<συπ>−</συπ>1) 3451, 2954, 1604, 1568, 1514, 1250, 1049, 840; HRMS (ESI) calcd for C20H32NONaSi2Br: 460.1098, found: 460.1097; [α]D24 –0.233 (c 0.99, CHCl3).
N-MOM indole 9. To a suspension of NaH (55w%, 382 mg, 8.75 mmol) in THF (8.9 mL) and DMF (4.2 mL) at 0 °C was added dropwise a solution of 2-silylindole 8 (2.74 g, 6.25 mmol) in THF (12 mL) over 20 min. After the resulting mixture was stirred at that temperature for 1 h, MOMCl (0.55 mL, 7.19 mmol) was added dropwise via syringe. The reaction mixture was stirred at 0 °C for 2 h, and H2O was added. The resulting mixture was extracted with ether, and the organic layer was washed with brine, dried over Na2SO4, filtrated, and concentrated under reduced pressure. The residue was purified by silica gel chromatography (hexane/AcOEt = 95:1) to afford 9 (2.93 g, 97% yield) as a yellow oil. Rf 0.61 (hexane/AcOEt = 6:1); 1H NMR (400 MHz, CDCl3) δ 7.54 (d, J = 1.7 Hz, 1H), 7.38 (d, J = 8.3 Hz, 1H), 7.18 (dd, J = 8.4, 1.6 Hz, 1H), 5.99 (dd, J = 17.3, 10.7 Hz, 1H), 5.40 (s, 2H), 5.20 (dd, J = 17.3, 1.5 Hz, 1H), 5.09 (dd, J = 10.7, 1.5 Hz, 1H), 3.19 (s, 3H), 2.93-2.70 (m, 2H), 1.73 (br t, J = 8.6 Hz, 2H), 1.42 (s, 3H), 0.43 (s, 9H), 0.16 (s, 9H); 13C NMR (100 MHz, CDCl3); δ 1.2 (CH3), 2.6 (CH3), 20.0 (CH2), 26.8 (CH3), 46.0 (CH2), 55.5 (CH3), 75.8 (C), 75.9 (CH2), 112.2 (CH2), 112.4 (CH), 116.6 (C), 120.2 (CH), 122.6 (CH), 128.0 (C), 128.1 (C), 135.8 (C), 141.0 (C), 145.3 (CH); IR (neat, cm<συπ>−</συπ>1) 2954, 1595, 1566, 1249, 1052, 839; HRMS (ESI) calcd for C22H36NO2NaSi2Br: 504.1360, found: 504.1340; [α]D24 +1.21 (c 0.933, CHCl3).
2-Iodoindole 10. To a solution of 2-silylindole 9 (679 mg, 1.41 mmol) in CH2Cl2 (28 mL) was added NIS (334 mg, 1.48 mmol). The reaction mixture was refluxed for 8 h in the dark. After the reaction mixture was cooled to rt, a saturated aqueous solution of Na2S2O3 was then added. The resulting mixture was extracted two times with CH2Cl2, and the combined organic layer was dried over Na2SO4, filtrated, and concentrated under reduced pressure to afford the crude iodide.
To a solution of the crude iodide in dry THF (28 mL) was added TBAF (1 M in THF, 2.4 mL, 2.8 mmol) at 0 °C. After the reaction mixture was stirred for 8 h at 0 °C, a saturated aqueous solution of NaHCO3 was added. The resulting mixture was extracted two times with AcOEt and the combined organic layer was dried over Na2SO4, filtrated, and concentrated under pressure. The residue was purified by silica gel chromatography (hexane/AcOEt = 8:1) to afford 10 (618 mg, 94% yield) as a white solid. mp 69-72 °C; Rf 0.46 (hexane/AcOEt = 2:1); 1H NMR (400 MHz, CDCl3) δ 7.61 (d, J = 1.7 Hz, 1H), 7.38 (d, J = 8.3 Hz, 1H), 7.22 (dd, J = 8.3, 1.7 Hz, 1H), 6.03 (dd, J = 17.5, 10.9 Hz, 1H), 5.45 (s, 2H), 5.32 (dd, J = 17.3, 1.0 Hz, 1H), 5.16 (dd, J = 17.3, 1.0 Hz, 1H), 3.29 (s, 3H), 2.78 (ddd, J = 14.2, 10.8, 6.4 Hz, 1H), 2.73 (ddd, J = 14.2, 10.8, 6.4 Hz, 1H), 18.2 (ddd, J = 13.6, 10.8, 6.4 Hz, 1H), 1.75 (ddd, J = 13.6, 10.8, 6.4 Hz, 1H), 1.39 (s, 3H); 13C NMR (100 MHz, CDCl3) δ 21.9 (CH2), 28.0 (CH3), 42.0 (CH2), 55.9 (CH3), 73.1 (C), 77.3 (CH2), 86.1 (C), 112.2 (CH2), 113.1 (CH), 116.3 (C), 119.4 (CH), 123.1 (C), 123.5 (CH), 127.2 (C), 139.3 (C), 144.6 (CH); IR (KBr, cm<συπ>−</συπ>1) 3444, 2928, 1600, 1459, 1336, 1089, 916, 845, 804; HRMS (ESI) calcd for C16H19NO2NaBrI: 485.9536, found: 485.9536; [α]D24 −10.87 (c 0.90, CHCl3).
Enantiomeric excess was determined by HPLC using a chiral column (DAICEL Chiralpak® AD-H; hexane/i-PrOH = 98:2; flow rate 1.0 mL/min; oven 30 °C; 10 Rt = 39.0 min, enantiomer Rt = 33.4 min; monitoring 254 nm; 98% ee). HRMS (ESI) calcd for 10 C16H19NO2NaBrI: 485.9536, found: 485.9526, HRMS (ESI) calcd for enantiomer C16H19NO2NaBrI: 485.9536, found: 485.9516.
Spirocyclic oxindole 11. A dry two-necked round-bottomed flask was charged with CuCl (24 mg, 0.24 mmol), 2-aminopyridine (23 mg, 0.24 mmol) and small amount of triglyme. The flask was evacuated and backfilled with argon. 2-Iodoindole 10 (564 mg, 1.2 mmol) in triglyme (24 mL) was added. After bubbling with argon for 3 min, NaOMe solution in MeOH (25w/w%, 1.1 mL, 4.8 mmol) was added. The reaction mixture was stirred vigorously at 100 °C for 26 h. After cooling to rt, the reaction mixture was filtered through Celite, and filtrate was concentrated under reduced pressure. The residue was added a saturated aqueous solution of NH4Cl and H2O. The mixture was extracted two times a solvent of hexane/AcOEt = 5:1. The combined organic layer was washed three times with H2O, dried over Na2SO4, filtered, and concentrated under reduced pressure. The residue purified by silica gel chromatography (hexane/AcOEt = 8:1) to afford spirocyclic oxindole 11 (219 mg, 69% yield) as a white solid. mp 150-155 °C; Rf 0.39 (hexane/AcOEt = 2:1); 1H NMR (400 MHz, CDCl3) δ 8.08 (br s, 1H), 7.11 (dd, J = 7.9, 1.8 Hz, 1H) 7.08 (d, J = 1.7 Hz, 1H), 7.02 (d, J = 8.1 Hz, 1H), 5.55-5.54 (m, 1H), 2.26-2.59 (m, 1H), 2.20-2.19 (m, 2H), 2.07 (ddd, J = 12.4, 9.6, 7.6 Hz, 1H), 2.00-1.93 (m, 1H), 1.80 (s, 3H); 13C NMR (400 MHz, CDCl3) δ 23.5 (CH3), 26.5 (CH2), 29.5 (CH2), 31.9 (CH2), 46.1 (C), 112.9 (CH), 118.5 (CH), 121.1 (C), 125.2 (CH), 125.6 (CH), 133.8 (C, 2 carbons), 141.1 (C), 182.6 (C); IR (KBr, cm<συπ>−</συπ>1) 3112, 2905, 2837, 1715, 1602, 1455, 1322, 1228, 806; HRMS (ESI) calcd for C14H14NONaBr: 314.0150, found: 314.0156; [α]D24 +132.3 (c 0.293, CHCl3).
Keto carboxylic acid 12. To a solution of spirocyclic oxindole 11 (457 mg, 1.56 mmol) in acetone (13 mL) and H2O (3 mL) was added OsO4 (2.35 mL, 0.02 M in t-BuOH, 0.047 mmol) and NMO (274 mg, 2.34 mmol). After the reaction mixture was stirred at rt for 4 h, a saturated aqueous solution of Na2S2O3 was added. The resulting mixture was extracted two times with AcOEt, and the combined organic layer was dried over Na2SO4, filtrated, and concentrated under reduced pressure to afford the crude diol.
To the mixture of the crude diol in THF (14 mL) and H2O (14 mL) was added NaIO4 (1.3 g, 6.24 mmol). After the reaction mixture was stirred at rt for 4 h, a saturated aqueous solution of Na2S2O3 was added. The resulting mixture was extracted two times with AcOEt. The combined organic layer was dried over Na2SO4, filtrated, and concentrated under reduced pressure to afford the crude ketoaldehyde.
To the solution of the crude ketoaldehyde in THF (10 mL), t-BuOH (20 mL), and H2O (20 mL) was added 2-methy-2-butene (1.6 mL, 15.6 mmol) and NaH2PO4·2H2O (733 mg, 4.7 mmol) and NaClO2 (425 mg, 4.7 mmol). The reaction mixture was stirred at rt for 11 h. The resulting mixture was concentrated under reduced pressure. The residue was diluted with 1 N HCl and extracted five times with CHCl3. The combined organic layer was dried over Na2SO4, filtered, and concentrated under reduced pressure. The residue purified by silica gel chromatography (hexane/AcOEt = 1:1 → CHCl3/MeOH = 6:1) to afford 12 (448 mg, 84% yield) as a white solid. mp 58-63 °C; Rf 0.36 (CHCl3/MeOH = 3:1); 1H NMR (400 MHz, CDCl3) δ 9.45 (s 1H), 7.17 (dd, J = 7.8, 1.5 Hz, 1H), 7.06 (d, J = 1.5 Hz, 1H), 6.98 (d, J = 8.1 Hz), 3.03 (d, J = 16.6 Hz, 1H), 2.82 (d, J = 16.3 Hz, 1H), 2.31-2.23 (m, 1H), 2.05-1.91 (m, 3H), 1.98 (s, 3H); 13C NMR (100 MHz, CDCl3) δ 29.9 (CH3), 31.2 (CH2), 37.3 (CH2), 40.9 (CH2), 49.3 (C), 114.0 (CH), 122.1 (C), 124.2 (CH), 125.8 (CH), 129.6 (C), 142.8 (C), 173.5 (C), 182.1 (C), 207.3 (C); IR (KBr, cm<συπ>−</συπ>1) 3263, 1717, 1611, 1482, 1187; HRMS (ESI) calcd for C14H14NO4NaBr: 361.9998, found: 362.0002; [α]D24 −36.27 (c 0.46, CHCl3).
exo-Methylene 13. To a solution of methyltriphenylphosphonium bromide (1.76 g, 4.94 mmol), dried at 70-80 °C for 20 h under reduced pressure before use, in THF (12 mL) was added n-BuLi (2.95 mL, 1.59 M in hexane, 2.95 mmol) at −25 °C. After stirring at −25 °C for 1 h, a solution of 12 (160 mg, 0.47 mmol) in THF (4 mL) was added at −25 °C. After stirring at −25 °C for 7 h, the reaction mixture was allowed to warm −5 °C and stirred for 15 h. The reaction mixture was allowed to warm rt and stirred for 4 h .The reaction mixture was added to a saturated aqueous NH4Cl and 1 N HCl. The resulting mixture was extracted four times with CH2Cl2. The combined organic layer was dried over Na2SO4, filtered, and concentrated under reduced pressure. The residue purified by silica gel chromatography (CHCl3/MeOH = 40:1 → 25:1 → 5:1; CHCl3/i-PrOH = 10:1) to afford 13 (~92 mg, ~57% yield) as a white solid; Rf 0.36 (CHCl3/MeOH = 5:1); 1H NMR (400 MHz, CDCl3) δ 8.68 (br s, 1H), 7.19 (dd, J = 7.8, 1.6 Hz, 1H), 7.08 (d, J = 1.6 Hz, 1H), 7.02 (d, J = 7.8 Hz, 1H), 4.65 (s, 1H), 4.56 (s, 1H), 3.05 (d, J = 16.1 Hz, 1H), 2.83 (d, J = 16.1 Hz, 1H), 2.02-1.96 (m, 1H), 1.88-1.77 (m, 2H), 1.61-1.53 (m, 1H), 1.61 (s, 3H).
endo-Alkene 14. A solution of 13 (~92 mg, ~0.27 mmol) in 1,4-dioxane (2.7 mL) was added to H2SO4 (165 mg, 95%, 1.6 mmol). The mixture was stirred at 60 °C for 6 h and MgSO4 (920 mg, 10w/w based on 97) was added. After 10 h of additional stirring at 60 °C, the reaction mixture was cooled to rt and then poured into ice water. This mixture was extracted four times with CHCl3 and the combined organic layer was dried over Na2SO4, filtered, and concentrated under reduced pressure. The residue was purified by silica gel column chromatography (CHCl3/MeOH = 10:1) to afford 14 (~46.5 mg, ~50% yield) as a white solid. Rf 0.36 (CHCl3/MeOH = 5:1); 1H NMR (400 MHz, CDCl3) δ 8.39 (br s, 1H), 7.15 (dd, J = 7.8, 1.6 Hz, 1H), 7.05 (d, J = 1.7 Hz, 1H), 7.00 (d, J = 8.0 Hz, 1H), 4.88 (t, J = 7.8 Hz, 1H), 3.04 (d, J = 16.6 Hz, 1H), 2.86 (d, J = 16.6 Hz, 1H), 2.42 (t, J = 7.8 Hz, 2H), 1.62 (s, 3H), 1.48 (s, 3H).
Prepnyl ester 15. To a solution of 14 (~50.4 mg, ~0.15 mmol) in acetone (3 mL) was added K2CO3 (243 mg, 1.76 mmol) and prenyl bromide (0.18 mL, 1.5 mmol). The reaction mixture was refluxed for 19 h. After the reaction mixture was cooling to rt, the reaction mixture was filtered through a pad of Celite, and filtrate was concentrated under reduced pressure. The residue was added AcOEt. The mixture was washed two times with brine, dried over Na2SO4, filtered, and concentrated under reduced pressure. The residue was purified by silica gel column chromatography (hexane/AcOEt = 30:1) to afford prenyl ester 15 (41 mg, 18% yield from keto carboxylic acid 12) as a colorless oil. Rf 0.55 (hexane/AcOEt = 3:1); 1H NMR (400 MHz, CDCl3) δ 7.11 (dd, J = 7.8, 1.7 Hz, 1H), 7.01 (d, J = 7.8 Hz, 1H), 6.88 (d, J = 1.7 Hz, 1H), 5.10 (tt, J = 6.6, 1.3 Hz, 1H), 4.99 (tt, J = 7.3, 1.3 Hz, 1H), 4.80 (tt, J = 7.6, 1.3 Hz, 1H), 4.45 (dd, J = 15.6, 6.6 Hz, 1H), 4.39 (dd, J = 12.3, 7.3 Hz, 1H), 4.25 (dd, J = 12.3, 7.3 Hz, 1H), 4.12 (dd, J = 15.6, 6.6 Hz, 1H), 3.02 (d, J = 16.3, 1H), 2,85 (d, J = 16.3 Hz, 1H), 2.42 (dd, J = 14.4, 7.6 Hz, 1H), 2.37 (dd, J = 14.0, 8.0 Hz, 1H), 1.83 (s, 3H), 1.74 (s, 3H), 1.68 (s, 3H), 1.58 (s, 3H), 1.55 (s, 3H), 1.47 (s, 3H); 13C NMR (100 MHz, CDCl3) δ 178.6 (C), 169.7 (C), 145.0 (C), 139.2 (C), 136.6 (C), 136.5 (C), 130.2 (C), 124.5 (CH), 124.1 (CH), 121.5 (C), 118.3 (CH), 118.0 (CH), 116.6 (CH), 111.9 (CH), 61.4 (CH2), 49.7 (C), 40.0 (CH2), 38.2 (CH2), 36.4 (CH2), 25.9 (CH3), 25.7 (CH3), 25.6 (CH3), 18.2 (CH3), 18.0 (CH3), 17.8 (CH3); IR (neat, cm<συπ>−</συπ>1) 2925, 1730, 1604, 1171, 594; HRMS (ESI) calcd for C25H32NO3NaBr: 496.1457, found: 496.1460; [α]D23 −11.9 (c 1.36, CHCl3).
Methyl amide 16. To a solution of prenyl ester 15 (20.4 mg, 0.043 mmol) in MeOH (0.5 mL) was added 40% aqueous MeNH2 (0.5 mL). The reaction mixture was stirred at rt for 35 h. The mixture was diluted with AcOEt and the organic layer was washed two times with a saturated aqueous NH4Cl and one time with brine, dried over Na2SO4, filtrated, and concentrated under reduced pressure. The residue was purified by silica gel column chromatography (hexane/AcOEt = 2:1 → CHCl3/MeOH = 12:1) to afford methyl amide 16 (12.1 mg, 67% yield) as a white solid. mp 155-159 °C; Rf 0.45 (CHCl3/MeOH = 12:1); 1H NMR (400 MHz, CDCl3) δ 7.16 (dd, J = 7.8, 1.7 Hz, 1H), 7.09 (d, J = 7.8 Hz, 1H), 6.90 (d, J = 1.7 Hz, 1H), 5.99 (s, 1H), 5.06 (tt, J = 6.6, 1.3 Hz, 1H), 4.75 (tt, J = 7.6, 1.3 Hz, 1H), 4.39 (dd, J = 15.6, 6.6 Hz, 1H), 4.19 (dd, J = 15.6, 6.6 Hz, 1H), 2.80 (d, J = 14.9 Hz, 1H), 2.68 (d, J = 14.2 Hz, 1H), 2.65 (d, J = 4.9 Hz, 3H), 2.48 (d, J = 7.6 Hz, 2H), 1.82 (s, 3H), 1.73 (s, 3H), 1.57 (s, 3H), 1.46 (s, 3H); 13C NMR (100 MHz, CDCl3) δ 179.3 (C), 169.3 (C), 144.2 (C), 136.9 (C), 136.4 (C), 130.4 (C), 125.1 (CH), 124.6 (CH), 121.5 (C), 118.0 (CH), 116.7 (CH), 112.1 (CH), 50.5 (C), 42.2 (CH2), 38.2 (CH2), 36.0 (CH2), 26.2 (CH3), 25.8 (CH3), 25.7 (CH3), 18.2 (CH3), 18.0 (CH3); IR (KBr, cm<συπ>−</συπ>1) 3272, 3096, 2973, 2916, 1722, 1630, 1603, 1160; HRMS (ESI) calcd for C21H27N2O2NaBr: 441.1148, found: 441.1149; [α]D23 −14.34 (c 0.81, CHCl3).
(−)-Flustramide B (17). To a solution of 16 (5.3 mg, 0.0126 mmol) in THF was added dropwise AlH3·EtNMe2 (0.5 M in toluene, 0.13 mL, 0.065 mmol) at −20 °C. After the reaction mixture was stirred for 40 min at −20 °C, THF-H2O (v/v = 1:1) was added dropwise. The resulting mixture was added to a saturated aqueous NaHCO3, and extracted two times with AcOEt. The organic layer was dried over Na2SO4, filtrated, and concentrated under reduced pressure. The residue was purified by silica gel column chromatography (hexane/AcOEt = 2:1) to afford (−)-flustramide B (17) (4.9 mg, 96% yield) as a colorless oil. Rf 0.37 (hexane/AcOEt = 1:3); 1H NMR (400 MHz, CDCl3) δ 6.85 (br s, 2H), 6.60 (br s, 1H), 5.20-5.16 (m, 1H), 4.98-4.91 (m, 1H), 4.72 (s, 1H), 3.96 (dd, J = 16.0, 6.3 Hz, 1H), 3.89 (dd, J = 15.9, 7.1 Hz, 1H), 2.87 (s, 3H), 2.64 (s, 2H), 2.37 (dd, J = 14.7, 7.8 Hz, 1H), 2.32 (dd, J = 15.0, 6.8 Hz, 1H), 1.75 (s, 3H), 1.74 (s, 3H), 1.70 (s, 3H), 1.56 (s, 3H); 13C NMR (100 MHz, CDCl3) δ 172.8, 150.6, 136.1, 136.0, 134.3, 124.4, 122.2, 121.5, 120.1, 118.2, 111.7, 87.4, 49.6, 46.6, 41.7, 37.4, 28.0, 26.0, 25.7, 18.1(4), 18.1(3); IR (neat, cm<συπ>−</συπ>1) 2967, 2913, 1696, 1597, 668; HRMS (ESI) calcd for C21H27N2ONaBr: 425.1198, found: 425.1205; [α]D23 −117.37 (c 0.30, CHCl3), [lit.,6a [α]D18 −116.1 (c 1.72, CHCl3)].
(−)-Flustramine B (1). To a solution of 17 (4.9 mg, 0.0117 mmol) in THF was added dropwise AlH3·EtNMe2 (0.052 mL, 0.5 M in toluene, 0.026 mmol) at rt. After the reaction mixture was stirred at rt for 10 min, THF-H2O (v/v = 1:1) was added dropwise. The resulting mixture was added to a saturated aqueous NaHCO3, and extracted two times with AcOEt. The organic layer was dried over Na2SO4, filtrated, and concentrated under reduced pressure. The residue was purified by silica gel column chromatography (hexane/AcOEt = 2:1) to afford (−)-flustramine B (1) (4.2 mg, 93% yield) as a colorless oil. Rf 0.32 (hexane/AcOEt = 1:3); 1H NMR (400 MHz, CDCl3) δ 6.80 (d, J = 7.8 Hz, 1H), 6.73 (dd, J = 7.8, 1.7 Hz, 1H), 6.49 (d, J = 1.7 Hz, 1H), 5.14-5.11 (m, 1H), 4.95-4.91 (m, 1H), 4.28 (s, 1H), 3.88 (dd, J = 16.1, 5.6 Hz, 1H), 3.79 (dd, J = 16.1, 7.3 Hz, 1H), 2.67 (ddd, J = 9.4, 6.6, 3.4 Hz, 1H), 2.55 (td, J = 9.3, 5.8 Hz, 1H), 2.47 (s, 3H), 2.83 (d, J = 7.6 Hz, 2H), 2.03 (ddd, J = 11.7, 9.0, 6.6 Hz, 1H), 1.86 (ddd, J = 12.0, 5.8, 3.4 Hz, 1H), 1.71 (br s, 6H), 1.65 (br s, 3H), 1.57 (br s, 3H); 13C NMR (100 MHz, CDCl3) δ 153.1, 134.78, 134.76, 133.8, 124.0, 121.3, 120.7, 120.4, 119.9, 110.0, 91.6, 56.8, 52.8, 46.2, 39.0, 38.3, 38.1, 25.9, 25.7, 18.15, 18.09; IR (neat, cm<συπ>−</συπ>1) 2965, 2928, 2792, 1599, 1485, 915; HRMS (ESI) calcd for C21H29N2Br+H: 389.1586, found: 389.1589; [α]D23 −115.3 (c 0.27, CHCl3), [lit.,6a [α]D18 −105.9 (c 0.77, CHCl3)].
ACKNOWLEDGEMENTS
This work was supported by a Grant-Aid for Young Scientists (B) (KAKENHI 19790026) from the Ministry of Education, Culture, Sports, Science and Technology of Japan, NOVARTIS Foundation (Japan) for the Promotion of Science, and Chugai Pharmaceutical Co., Ltd (A.N.). We thank Takasago International Corporation for a generous gift of (–)-linalool.
References
1. (a) J. S. Carlé and C. Christophersen, J. Am. Chem. Soc., 1979, 101, 4012; CrossRef (b) J. S. Carlé and C. Christophersen, J. Org. Chem., 1980, 45, 1586; CrossRef (c) P. B. Holst, U. Anthoni, C. Christophersen, and P. H. Nielsen, J. Nat. Prod., 1994, 57, 997. CrossRef
2. T. Sjõblom, L. Bohlin, and C. Christophersen, Acta Pharm. Suec., 1983, 20, 415.
3. T. F. Spande, M. W. Edwards, L. K. Pannell, and J. W. Daly, J. Org. Chem., 1988, 53, 1222. CrossRef
4. E. Rivera-Becerril, P. Joseph-Nathan, V. M. Pérez-Álvarez, and M. S. Morales-Ríos, J. Med. Chem., 2008, 51, 5271. CrossRef
5. J. W. Hodgson, M. O. Mitchell, M. L. Thomas, K. F. Waters, and D. Powell, Bioorg. Med. Chem. Lett., 1995, 5, 2527. CrossRef
6. For synthesis of enantiomerically pure pyrrolidinoindolines, see: (a) T. Kawasaki, M. Shinada, D. Kamimura, M. Ohzono, and A. Ogawa, Chem. Commun., 2006, 420; CrossRef (b) M. Bruncko, D. Crich, and R. Samy, J. Org. Chem., 1994, 59, 5543; CrossRef (c) M. S. Morales-Ríos, E. Rivera-Becerril, and P. Joseph-Nathan, Tetrahedron: Asymmetry, 2005, 16, 2493; CrossRef (d) A. S. Cardoso, N. Srinivasan, A. M. Lobo, and S. Prabhakar, Tetrahedron Lett., 2001, 42, 6663; CrossRef for racemic synthesis, see: (e) H. Miyamoto, Y. Okawa, A. Nakazaki, and S. Kobayashi, Tetrahedron Lett., 2007, 48, 1805; CrossRef (f) P. López-Alvarado, E. Caballero, C. Avendaño, and J. C. Menéndez, Org. Lett., 2006, 8, 4303; CrossRef (g) G. H. Tan, X. Zhu, and A. Ganesan, Org. Lett., 2003, 5, 1801; CrossRef (h) M. S. Morales-Ríos, O. R. Suárez-Castillo, J. J. Trujillo-Serrato, and P. Joseph-Nathan, J. Org. Chem., 2001, 66, 1186; CrossRef (i) M. S. Morales-Ríos, O. R. Suárez-Castillo, and P. Joseph-Nathan, Tetrahedron, 2002, 58, 1479; CrossRef (j) M. Somei, F. Yamada, T. Izumi, and M. Nakajou, Heterocycles, 1997, 45, 2327; (k) J. Jensen, U. Anthoni, C. Christophersen, and P. H. Nielsen, Acta Chem. Scand., 1995, 49, 68; CrossRef (l) M. O. Mitchell and P. Dorroh, Tetrahedron Lett., 1991, 32, 7641. CrossRef
7. (a) H. Miyamoto, Y. Okawa, A. Nakazaki, and S. Kobayashi, Angew. Chem. Int. Ed., 2006, 45, 2274, and references therein; CrossRef (b) A. Nakazaki and S. Kobayashi, J. Synth. Org. Chem. Jpn., 2008, 66, 124.
8. We initially attempted a synthesis of (–)-1 from the PMB derivative of 10; however, the PMB-protected oxindoles were found to decompose during their deprotection. Thus, we decided to select an alternative protective group that would be more labile .
9. (a) R. C. Larock and E. K. Yum, J. Am. Chem. Soc., 1991, 113, 6689; CrossRef (b) R. C. Larock, E. K. Yum, and M. D. Refvik, J. Org. Chem., 1998, 63, 7652. CrossRef
10. P. V. Vyas, A. K. Bhatt, G. Ramachandraiah, and A. V. Bedekar, Tetrahedron Lett., 2003, 44, 4085. CrossRef
11. A. K. Flatt, Y. Yao, M. Francisco, and J. M. Tour, J. Org. Chem., 2004, 69, 1752. CrossRef
12. R. D. Fabio, G. Alvaro, B. Bertani, and S. Giacobbe, Can. J. Chem., 2000, 78, 809. CrossRef
13. (a) M. D. Lord, J. T. Negri, and L. A. Paquette, J. Org. Chem., 1995, 60, 191; CrossRef (b) G. Slomp and J. L. Johnson, J. Am. Chem. Soc., 1958, 80, 915. CrossRef
14. (a) S. Ohira, Synth. Commun., 1989, 19, 561; CrossRef (b) S. Muller, B. Liepold, G. J. Roth, and H. J. Bestmann, Synlett, 1996, 521. CrossRef
15. The investigation of the scope and limitation of the present method of deprotection is currently being carried out and its results will be described in due course .
16. (a) B. S. Bal, W. E. Childers, Jr., and H. W. Pinnick, Tetrahedron, 1981, 37, 2091; CrossRef (b) B. O. Lindgren and T. Nilsson, Acta Chem. Scand., 1973, 27, 888. CrossRef
17. Kawasaki and co-workers have also reported a similar result in which the yield of 1 synthesized using 5 equiv of AlH3·EtNMe2 at rt was low (34%).