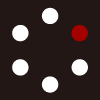
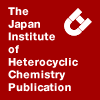
HETEROCYCLES
An International Journal for Reviews and Communications in Heterocyclic ChemistryWeb Edition ISSN: 1881-0942
Published online by The Japan Institute of Heterocyclic Chemistry
e-Journal
Full Text HTML
Received, 30th September, 2008, Accepted, 27th November, 2008, Published online, 2nd December, 2008.
DOI: 10.3987/COM-08-S(D)50
■ The Dakin-West Reaction of N-Acylprolines in the Presence of Inorganic Bases: Unexpected Formation of Enol Esters
Momoko Saeki, Yuri Hagimoto, Hidemitsu Uno, and Masami Kawase*
Matsuyama University, 4-2 Bunkyo-cho, Matsuyama, Ehime 790-8578, Japan
Abstract
A novel transformation of N-acylprolines (1) to enol esters (2) was realized by utilizing chlorodifluoroacetic or trifluoroacetic anhydrides in the presence of inorganic bases, in which probable intermediates were mesoionic 1,3-oxazolium-5-olates. The structure of the enol ester (2a) was determined by single crystal X-ray analysis.INTRODUCTION
The Dakin-West (D-W) reaction of α-amino acids is originally carried out in acetic anhydride in the presence of pyridine to produce α-amino ketones.1 Recent application of the D-W reaction on α-amino acid derivatives has been used successfully in the preparation of biologically active molecules.2-6 Secondary amino acids are likewise known to undergo the D-W reaction and its mechanism has been studied in detail.1 It is generally accepted that an essential first step is the formation of mesoionic 1,3-oxazolium-5-olates. However, the D-W reaction of proline or its N-acyl derivatives with acetic anhydride are known not to afford any ketonic products.1 However, a generation of mesoionic oxazolium-5-olates A (Scheme 1) from N-acylprolines has been shown to react with dimethyl acetylenedicarboxylate. Thus, 2,3-dihydropyrrolo[2,1-b]thiazole derivatives were obtained through the 1,3-dipolar cycloaddition reaction of the mesoionic intermediates, formed through the dehydration of N-acylprolines with acetic anhydride.7-9 In previous communications, we have reported on the reactivity of A derived from N-acyl or N-alkoxycarbonylprolines.10-12 These substrates were cyclodehydrated with TFAA to the mesoionic compounds (münchnone intermediates) which reacted with TFAA and afforded 5-trifluoromethyloxazoles,10 trifluoromethylated acyloins11 or 3-trifluoroacetyl-4,5-dihydropyrrolidines,12 depending on the nature of N-acyl groups and the experimental conditions. Organic bases, such as pyridine, DMAP or 1-methylimidazole, have been widely used as catalysts for the D-W reaction.13 On the other hand, the efficiency of inorganic bases as catalysts for the D-W reaction has not been explored much. In our continuous studies in this field, we examined in more detail a D-W reaction of N-acylprolines with chlorodifluoroacetic anhydride (CFAA) or TFAA in the presence of inorganic bases as catalysts, and a new enol ester (2a-d) was unexpectedly obtained as a main product. Now we wish to report the results.
RESULTS AND DISCUSSION
As the first attempt, a mixture of N-pivaloylproline (1a) (1 mmol) and CFAA (3 mmol) was treated with K2CO3 (5 mmol) in benzene. The reaction provided the enol ester, (E)-(1-(2-Chloro-2,2-difluoroacetyl)- pyrrolidin-2-ylidene)methyl pivalate (2a) in 40% isolated yield (Table 1, entry 2). As the enol ester was so unstable to decompose gradually during chromatography on a column of silica gel, we estimated the yield of the enol ester based on 1H-NMR calibration with piperonal as an internal standard. During the optimization of reaction conditions, we found that, even without inorganic base, the enol ester formation took place to afford 2a in low yield, 8% yield (Table 1, entry 1). Three equiv. of CFAA and five equiv. of K2CO3 against 1a were needed to obtain a satisfactory yield of 2a. The use of five equiv. of CFAA did not improve the yield. A high temperature was needed to obtain a better yield of 2a, the rt reducing the yield (19%). It is noteworthy that no reaction was observed for 1a with acetic anhydride in the presence of K2CO3 and little or no enol ester was detected in the 1H NMR spectra of the reaction mixture. In the presence of pyridine (six equiv.), 2-tert-butyl-5-chlorodifluoromethyl-4- (3-hydroxypropyl)oxazole was obtained in 25% isolated yield as a main product in the reaction of 1a and CFAA.14 The enol ester (2a) was only formed in 0.6% yield. Therefore, combination of CFAA and K2CO3 has been proved to promote the formation of the enol ester (2).
Next we have screened the reactions of inorganic solid bases, such as K2CO3, Na2CO3, NaHCO3, KOBut, Cs2CO3, CaCO3, Li2CO3, NaOCOCClF2, KH2PO4, and K3PO4 (Table 1). Na2CO3 and K3PO4 also worked with a slightly lower catalytic activity (Table 1, entries 3 and 5). Cs2CO3, Li2CO3 and CaCO3 were inferior to K2CO3. Use of other inorganic bases such as NaHCO3, KOBut, NaOCOCClF2, and KH2PO4 resulted in poorer conversions. K2CO3 is found to be the most effective inorganic base catalyst among these we have been evaluated, as shown in Table 1. Among the solvents investigated (Table 2), benzene and toluene have proven to be the solvent of choice for the transformation (Table 2, entries 1 and 2). Only a trace amount of the enol ester was detected when CHCl3 and t-BuOMe were used (Table 2, entries 3 and 4). When solvents such as THF, MeCN and MeNO2 were used, the enol ester was not detected in the reaction mixture (Table 2, entries 5, 6 and 7).
The reaction of N-acylprolines (1a, b) with TFAA under the optimized conditions afforded the enol esters (2c, d) in 14-18% yield (Table 3, entries 6 and 8). Also of interest to us was the formation of a trifluoromethyl ketone 3 which is a D-W reaction product employing TFAA. It is reported that the trifluoromethyl ketones are converted to the hydrates on silica gel during chromatography and the formation of the hydrates is responsible for the lowering of the yield.15 Therefore, we decided to isolate the trifluoromethyl ketones as alcohols after the reduction of the reaction mixture. Thus, after the reactions of 1 with TFAA, the reduction of the reaction mixture with NaBH4 afforded the trifluoromethyl alcohols (4c, d) in 8-16% isolated yields (Table 3, entries 6 and 8). Also, the yields of 4c and d were slightly improved by the NaBH4 reduction of the mixture which was obtained by performing the reaction of 1 with TFAA at rt for 15 h (Table 3, entries 5 and 7). However, in the reaction of 1a with CFAA, the alcohol was never observed or isolated (Table 3, entries 1 and 2). Only the reduction of the mixture obtained by the reaction of 1b with CFAA at rt afforded the corresponding trifluoromethyl alcohol (4b) in 4% isolated yield (Table 3, entry 3).
Unfortunately, all our attempts to run the reaction with derivatives of N-butoxycarbonylproline, N-phenoxycarbonylproline, N-benzoyl-N-methylalanine and N-benzoyl-N-benzylalanine failed. Thus, boiling at reaction mixture of the N-carbamoylproline or alanine derivatives with CFAA in benzene in the presence of K2CO3 for 5 h did not give any isolable reaction products. In the 1H NMR spectrum of the reaction mixture, no signal of the enol ester was observed. Thus, the enol ester formation reaction is specific to N-acylproline derivatives.
The structure of 2a was unequivocally confirmed by single-crystal X-Ray analysis (Figure 1). The X-Ray structure analysis indicates that the methylene moiety has the (E)-stereochemistry. The 1H NMR spectrum of 2a exhibited the signal of vinyl proton at δ 8.37 (singlet) and other products (2b-d) also had the characteristic vinyl protons at the almost same position. The carbons of CF3 groups in 2c and d appeared at around δ 116 ppm (JC-F = 286 Hz). On the other hand, the carbons of CClF2 groups in 2a and b were shown in an up-field shift of their NMR signals at around δ 119 ppm (JC-F = 302 Hz). The amide carbons of 2a-d appeared at around δ 155 ppm (JC-F = 30-38 Hz). The IR spectra of 2a-d exhibited an absorption band due to the ester carbonyl (around 1735 cm-1) and amido carbonyl (around 1685 cm-1) groups.
Although it is premature to discuss the precise mechanism at the present stage, the most plausible reaction pathway is presented as follows (Scheme 1). Initially N-acylprolines (1) react with acid anhydride to form mixed acid anhydrides. The reaction involves a mesoionic 1,3-oxazolium-5-olates (A) formed through the cyclodehydration of 1 by acid anhydride. Intermediates (A) are in equilibrium with the ketenes (B), whose formation could be facilitated by the elevated temperature of refluxing benzene. The ketenes (B) isomerize to the aldehyde (C) which undergo the cyclization converting to the intermediates (D). Ring opening of D followed by N-acylation leads to the formation of intermediates (E). Finally, the highly oxygenated E affords the enol esters 2.
In conclusion, we have demonstrated that the unique enol ester formation from N-acylprolines was not anticipated but the reaction is general as evidenced by the examples indicated in Table 3. In all cases the yields are modest, however this is offset by the fact that these compounds are obtained in one step from cheap and readily-available proline derivatives.
EXPERIMENTAL
The melting points were determined using a Yanagimoto hot-stage melting point apparatus and are uncorrected. NMR spectra were measured on either a Varian VXR-500, Bruker AVANCE500 (500 MHz for 1H and 126 MHz for 13C) or a JEOL JNM-PMX60SI (60 MHz for 1H) with tetramethylsilane (Me4Si) as an internal reference and CDCl3 as the solvent. Both 1H- and 13C-NMR spectral data are reported in parts per million (δ) relative to Me4Si. Infrared (IR) spectra were recorded on either a JASCO FT/IR-4100 or a JASCO IR-810 spectrometer. Low and HR MS were obtained with either a JEOL JMS-GCmate, a JEOL JMS-DX300, or a microTOF-Q (Bruker Daltonics) spectrometer with a direct inlet system at 70 eV. Elemental analyses were carried out in the microanalytical laboratory of Ehime University and Josai University.
Materials The following compounds were prepared by reported procedures16: N-pivaloylproline (1a): mp 131-132 °C (lit.,10 mp 128.3-129.6 °C). N-benzoylproline (1b): mp 155-157 °C (lit.,10 mp 153.9-154.3 °C). N-benzoyl-N-methylphenylalanine: mp 137-138°C (lit.,10 mp 137-138 °C). N-benzoyl-N-benzylphenylalanine: mp 114-116 °C (lit.,10 mp 114-116 °C). N-butoxycarbonylproline: Yield 96%. 1H-NMR (60 MHz) δ: 0.90 (t, 3H, J = 6.0 Hz), 1.15-1.87 (m, 4H), 1.87-2.55 (m, 4H), 3.48 (t, 2H, J = 6.0 Hz)¸4.08 (t, 2H, J = 6.0 Hz), 3.83-4.57 (m, 1H), 9.18 (s, 1H). IR (oil) cm-1: 1700, 1745, 2400-3700. MS m/z: 215 (M+, 1%), 70 (100%). High-resolution MS: Calcd for C10H17NO4: 215.1158. Found: 215.1160. N-phenoxycarbonylproline: Yield 75%. mp 95-96 °C (AcOEt-hexane). IR (Nujol) cm-1: 1655, 1725, 3320-2750. 1H-NMR (60 MHz) δ: 1.67-2.48 (m, 4H), 3.35-3.83 (m, 2H), 4.22-4.67 (m, 1H), 7.17 (s, 5H), 8.35 (s, 1H); MS m/z: 235 (M+, 1%), 94 (100%). Anal. Calcd for C12H13NO4: C, 61.27; H, 5.57; N, 5.95. Found: C, 61.31; H, 5.55; N, 5.99.
General Procedure for the Reaction of N-Acylproline (1a, b) with TFAA or CFAA: N-Acylproline (1)(1 mmol) was added to a solution of TFAA or CFAA (3 mmol) and K2CO3 (5 mmol) in benzene at 0 °C and the mixture was stirred for 2h at rt, then refluxed for 5h. The reaction mixture was diluted with 3% aqueous NaHCO3 (30 mL) with cooling and extracted with EtOAc (30 mL x 2). The combined extracts were washed with brine (30 mL). The organic layer was dried over Na2SO4 and concentrated under reduced pressure at 40°C using a rotary evaporator. The residue was chromatographed on a column of silica gel with EtOAc-hexane as the eluent to give the enol ester (2).
(E)-(1-(2-Chloro-2,2-difluoroacetyl)pyrrolidin-2-ylidene)methyl pivalate (2a): mp 60-62 °C (hexane); IR (KBr) cm-1: 1683, 1737, 3168; FAB-MS m/z: 296 (M++1, 80%), 298 (30%); 1H NMR (500 MHz) δ: 1.26 (s, 9H), 2.00-2.06 (m, 2H), 2.73-2.77 (m, 2H), 3.96-3.99 (m, 2H), 8.37 (s, 1H); 13C NMR (126 MHz) δ: 22.97 (CH2), 26.33 (CH2), 27.11 (CH3), 38.89 (C), 50.45 (CH2), 118,49 (CClF2, t, 1JC-F = 302 Hz), 127.17 (CH), 130.41 (C), 155.65 (C, t, 2 JC-F = 30.8 Hz), 174.93 (C). HRESIMS: Calcd for C12H1635ClF2NO3+Na (C12H1637ClF2NO3+Na): 318.06845 (320.0655). Found: 318.0674 (320.0639).
(E)-(1-(2-Chloro-2,2-difluoroacetyl)pyrrolidin-2-ylidene)methyl benzoate (2b): IR (KBr) cm-1: 1685, 1732, 3168. 1H NMR (500 MHz) δ: 2.05-2.11 (m, 2H), 2.89-2.93 (m, 2H), 4.01-4.04 (t, 2H), 7.46-7.49 (t, 2H, J = 7.8 Hz), 7.60 (m,1H), 8.08-8.10 (dd, 2H, J = 1.1, 8.2 Hz), 8.64(t, 1H, J = 2.2 Hz); 13C NMR (126 MHz) δ: 23.03 (CH2), 26.61 (CH2), 50.51 (CH2), 118.50 (CClF2, t, 1JC-F = 302 Hz), 127.10 (CH), 128.56 (CH), 129.23 (C), 129.74 (CH), 130.97 (C), 133.48 (CH), 155.74 (C, t, 2JC-F = 30.4 Hz), 163.09 (C). HRESIMS: Calcd for C14H1235ClF2NO3+Na (C14H1237ClF2NO3+Na): 338.0372 (340.0342). Found: 338.0353 (340.0324).
(E)-(1-(2,2,2-Trifluoroacetyl)pyrrolidin-2-ylidene)methyl pivalate (2c): IR (KBr) cm-1: 1685, 1739, 3158. 1H NMR (500 MHz) δ: 1.26 (s, 9H), 2.00-2.06 (m, 2H), 2.74-2.77 (m, 2H), 3.91-3.94 (m, 2H), 8.37 (t, 1H, J = 2.3 Hz); 13C NMR (126 MHz) δ: 22.82 (CH2), 26.39 (CH2), 27.09 (CH3), 38.87 (C), 49.63 (CH2), 115.92 (CF3, q, 1JC-F = 287 Hz), 127.20 (CH), 130.06 (C), 153.74 (C, t, 2JC-F = 37.2 Hz), 174.91 (C). HRESIMS: Calcd for C12H16F3NO3+Na: 302.0980, Found: 302.0996.
(E)-(1-(2,2,2-Trifluoroacetyl)pyrrolidin-2-ylidene)methyl benzoate (2d): IR (KBr) cm-1: 1690, 1730, 3172; 1H NMR (500 MHz) δ: 2.06-2.11 (m, 2H), 2.90-2.93 (m, 2H), 3.96-3.99 (t, 2H, J = 7.0 Hz), 7.44-7.49 (m, 2H), 7.60 (m, 1H), 8.05-8.11 (m, 2H), 8.66 (s, 1H); 13C NMR (126 MHz) δ: 22.89 (CH2), 26.69 (CH2), 49.64 (CH2), 115.93 (CF3, q, 1JC-F = 286 Hz), 127.14 (CH), 128.56 (CH), 129.19 (C), 129.56 (CH), 130.63 (C), 133.50 (CH), 153.85 (C, q, 2JC-F = 38.1 Hz), 163.07(C). HRESIMS: Calcd for C14H12F3NO3+Na: 322.0667, Found: 322.0653.
General Procedure for the Reduction with NaBH4: N-Acylproline (1)(1 mmol) was added to a solution of TFAA or CFAA (3 mmol) and K2CO3 (5 mmol) in benzene at 0 °C and the mixture was stirred for 15 h at rt. After the reaction mixture was diluted with EtOH (5 mL), NaBH4 (114 mg, 3 mmol) was added to a stirred solution of the mixture at 0 °C. The mixture was stirred for 1 h at rt, then at 60 °C for 5 h. The reaction mixture was diluted with 3% aqueous NaHCO3 (30 mL) with cooling and extracted with EtOAc (30 mL x 2). The combined extracts were washed with brine (30 mL). The organic layer was dried over Na2SO4 and concentrated under reduced pressure at 40 °C using a rotary evaporator. The residue was chromatographed on a column of silica gel with EtOAc-hexane as the eluent to give the alcohol (4).
(2-(2-Chloro-2,2-difluoro-1-hydroxyethyl)pyrrolidin-1-yl)(phenyl)methanone (4b): IR (KBr) cm-1: 1617, 3120-3550. 1H NMR (500 MHz) δ: 1.73-1.80 (m, 1H), 1.90-1.97 (m, 2H), 2.25 (q, 1H, J = 7.7 Hz), 3.47-3.52 (m, 1H), 3.61-3.65 (m, 1H), 3.94-4.00 (m, 1H), 4.71-4.76 (m, 1H), 6.04 (d, 1H, J = 6.7 Hz), 7.42-7.47 (m, 5H). 13C NMR (126 MHz) δ: 25.27 (CH2), 28.79 (CH2), 51.11 (CH2), 59.11 (CH), 79.37 (CH, 2JC-F = 25.3 Hz), 127.20 (CH), 128.50 (CH), 129.79 (CClF2, 1JC-F = 297.5 Hz), 130.74 (CH2), 135.65 (CH), 174.81 (C). FAB-MS m/z: 292 (M++1, 15%) + 290 (M++1, 32%)(1:3), 105 (100%). HR MS: Calcd for C13H1435ClF2NO2+Na (C13H1437ClF2NO2+Na): 312.0579 (314.0549). Found: 312.0600 (314.0536).
2,2-Dimethyl-1-(2-(2,2,2-trifluoro-1-hydroxyethyl)pyrrolidin-1-yl)propan-1-one (4c): mp 57-62 °C (AcOEt-hexane); IR (KBr) cm-1: 1612, 3130-3670. 1H NMR (500 MHz) δ: 1.27 (s, 9H), 1.85-1.93 (m, 2H), 1.97-2.04 (m, 2H), 3.52-3.57 (m, 1H), 3.76-3.82 (m, 1H), 3.85-3.90 (m, 1H), 4.61-4.65 (m, 1H), 5.71 (br s, 1H). 13C NMR (126 MHz) δ: 25.18 (CH2), 26.97 (CH2), 27.56 (CH3), 39.50 (C), 48.11 (CH2), 59.08 (CH), 74.53 (CH, 2JC-F = 27.7 Hz), 124.83 (CF3, 1JC-F = 284 Hz), 181.75 (C). GC-MS m/z: 154 (100%), 253 (M+, 0.7%). HR MS: Calcd for C11H18F3NO2+Na: 276.1187. Found: 276.1199. Anal. Calcd for C11H18F3NO2: C, 52.17; H, 7.16; N, 5.53. Found: C, 51.90; H, 7.16; N, 5.51.
Phenyl(2-(2,2,2-trifluoro-1-hydroxyethyl)pyrrolidin-1-yl)methanone (4d): mp 107-109 °C (AcOEt-hexane); IR (KBr) cm-1: 1624, 3120-3540. 1H NMR (500 MHz) δ: 1.73-1.81 (m, 1H), 1.91-1.98 (m, 2H), 2.22-2.27 (m, 1H), 3.47-3.52 (m, 1H), 3.59-3.64 (m, 1H), 3.97-4.01(m, 1H), 4.69 (q, 1H, J = 7.8Hz), 5.92 (d, 1H, J = 5.8Hz), 7.41-7.53 (m, 5H). 13C NMR (126 MHz) δ: 25.18 (CH2), 28.30 (CH2), 51.08 (CH2), 58.32 (CH), 75.02 (CH, 2JC-F = 28.7 Hz), 124.66 (CF3, 1JC-F = 284 Hz), 127.18 (CH), 128.49 (CH), 130.73 (CH), 135.65 (C), 174.62 (C). GC-MS: m/z=105 (100%), 273 (M+, 0.8%). HR MS: Calcd for C13H14F3NO2+Na: 296.0874. Found: 296.0898. Anal. Calcd for C13H14F3NO2: C, 57.14; H, 5.16; N, 5.13. Found: C, 57.05; H, 5.10; N, 5.16.
X-Ray structure determination
Crystal data of 2a: Determination of cell parameters and collection of reflection intensities were performed on Rigaku Mercury-8 (3-kW sealed tube) instrument equipped with graphite monochromated Mo Ka radiation. The data were corrected for Lorentz, polarization, and absorption effects. The structures were solved by SIR-9717 and expanded using the Fourier technique.18 Hydrogen atoms were placed in calculated positions and refined by using riding models. Calculations were performed by using the CrystalStructure crystallographic software package.19 Shelxl-9720 was used for the structure refinement. The structure data obtained were validated by the PLATON program21 on WinGX.22 The data in the CIF format were deposited to the Cambridge Crystallographic Data Centre (CCDC). Crystallographical data for compound 2a (CCDC-697097) are as follows: Crystal formula, C12H16ClF2NO3; 0.40 x 0.40 x 0.40 mm; monoclinic, space group P21/a; a = 9.5573(13), b = 11.4539(15), c = 12.9596(17) Å, β = 96.227(3)°, V = 1410.3(3) Å3; Mo K<συβ>α</συβ>, ¬T = 150 K, Z = 4, ρcalcd = 1.393 g·cm-3, μ = 0.297 cm-1, F(000) = 616, 14368 measured, 3196 unique, 2959 observed [I>2σ(I)]; R¬1 = 0.0375 [I>2σ(I)], wR2 = 0.0976 (all); GOF = 1.053.
ACKNOWLEDGEMENTS
Financial support in the form of a Grant-in-Aid for Scientific Research (C) (Kawase, No. 20590114) from Japan Society for the Promotion of Science (JSPS) is gratefully acknowledged.
References
1. G. L. Buchanan, Chem. Soc. Rev., 1988, 17, 91. CrossRef
2. Y. M. Loksha, A. A. El-Barbary, M. A. El-Badawi, C. Nielsen, and E. B. Pedersen, Bioorg. Med. Chem., 2005, 13, 4209. CrossRef
3. A. G. Godfrey, D. A. Brooks, L. A. Hay, M. Peters, J. R. McCarthy, and D. Mitchell, J. Org. Chem., 2003, 68, 2623. CrossRef
4. Y. M. Loksha, M. A. El-Badawi, A. A. El-Barbary, E. B. Pedesen, and C. Nielsen, Arch. Pharm., 2003, 336, 175. CrossRef
5. Y. M. Loksha, P. T. Jorgensen, E. B. Pedersen, M. A. El-Badawi, A. A. El-Barbary, and C. Nielsen, J. Heterocycl. Chem., 2002, 39, 375. CrossRef
6. R. W. Fischer and M. Misun, Org. Process Res. Develop., 2001, 5, 581. CrossRef
7. R. Huisgen, H. Gotthardt, H. O. Bayer, and F. C. Schaefer, Chem. Ber., 1970, 103, 2611. CrossRef
8. F. M. Hershenson, J. Org. Chem., 1975, 40, 1260. CrossRef
9. I. Lalezeri and E. L. Schwartz, J. Med. Chem., 1988, 31, 1427. CrossRef
10. M. Kawase, H. Miyamae, and T. Kurihara, Chem. Pharm. Bull., 1998, 46, 749.
11. M. Kawase, S. Saito, and T. Kurihara, Chem. Pharm. Bull., 2000, 48, 1338.
12. M. Kawase, M. Hirabayashi, H. Koiwai, K. Yamamoto, and H. Miyamae, Chem. Commun., 1998, 641. CrossRef
13. K.-V. Tran and D. Bickar, J. Org. Chem., 2006, 71, 6640. CrossRef
14. Full details of the D-W reaction of N-acylprolines or N-acyl-N-alkyl-α-amino acids with chlorodifluoroacetic anhydride in the presence of pyridine will be reported elsewhere.
15. M. A. McClinton and D. A. McClinton, Tetrahedron, 1992, 48, 6555. CrossRef
16. P. Quitt. J. Helberbach, and K. Vogler, Helv. Chim. Acta, 1963, 46, 327. CrossRef
17. SIR-97: A package for crystal structure solution and refinement, Istituto di Gristallografia, Italy; A. Altomare, M. C. Burla, M. Camalli, G. Cascarano, C. Giacovazzo, A. Guagliardi, A. G. G. Moliterni, G. lidori, and R. Rpagna, J. Appl. Cryst., 1999, 32, 115. CrossRef
18. DIRDIF-2008: A Computer Program for Crystal Structure Determination by Patterson Methods and Direct Methods applied to Difference Structure Factors. P. T. Beurskens, G. Beurskens, R. de Gelder, J. M. M. Smits, S. Garcia-Granda, and R. O. Gould, Crystallography Laboratory, Radboud University Nijmegen, Toernooiveld 1, 6526 ED Nijmegen, The Netherlands, 2008.
19. CrystalStructure Ver. 3.8.2, Crystal structure analysis package, Rigaku (3-9-12 Akishima, Tokyo, Japan) and Rigaku/MSC (9009 New Trails Dr., The Woodlands, TX 77381 USA).
20. Shelxs-97 and Shelxl-97 (wshelxs and wshelxl for WinGX): Program for the refinement of crystal structures from diffraction data, University of Göttingen, Göttingen, Germany; G. M. Sheldrick and T. Schneider, Methods Enzymol., 1997, 277, 319; CrossRef G. M. Sheldrick, Acta Cryst., 2008, A64, 112.
21. PLATON, A Multipurpose Crystallographic Tool, Utrecht University, Utrecht, The Netherland; A. L. Spek, J. Appl. Cryst., 2003, 36, 7. CrossRef
22. WinGX 1.80.00 suite for small-molecule single-crystal crystallography, University of Glasgow, L. J. Farrugia, J. Appl. Cryst., 1999, 32, 837. CrossRef