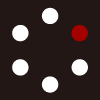
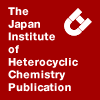
HETEROCYCLES
An International Journal for Reviews and Communications in Heterocyclic ChemistryWeb Edition ISSN: 1881-0942
Published online by The Japan Institute of Heterocyclic Chemistry
e-Journal
Full Text HTML
Received, 30th September, 2008, Accepted, 21st November, 2008, Published online, 25th November, 2008.
DOI: 10.3987/COM-08-S(D)51
■ Syntheses and Absolute Configuration Assignments of Mono- and Di-substituted Chiral Quinoline Alkaloids Obtained by Asymmetric Oxidation
Derek R. Boyd,* Narain D. Sharma, Stephen A. Barr, and Pui L. Loke
School of Chemistry and Chemical Engineering, The Queen's University of Belfast, David Keir Kuilding, Belfast, BT9 5AG, U.K.
Abstract
Mono- and di-substituted quinoline, 2-quinolone, dihydrofuroquinoline and dihydropyranoquinoline alkaloids have been synthesised with enantiomeric excess values of > 90%, via asymmetric epoxidation, or asymmetric dihydroxylation of the corresponding alkene precursors. The absolute configurations of isobalfourodine, ψ-balfourodine, ψ-isobalfourodine, isobalfourodinium methiodide, balfourolone, hydroxylunidine, orixine, nororixine, isopteleflorine, O-acetylisopteleflorine, O-methylhydroxylunium methiodide and hydroxylunine have been rigorously determined by a combination of circular dichroism spectroscopy, ozonolysis, and stereochemical correlation. Of these, the absolute configurations of six alkaloids were previously unknown and six were assigned incorrect configurations in the literature.Dedicated to the memory of Dr. John Daly, a good friend and mentor
INTRODUCTION
Quinoline alkaloids are widely distributed among the Rutaceae family of plants and several hundred members have been isolated to date. Recent comprehensive annual reviews of quinoline alkaloids1 provide clear evidence of the diverse, and continually expanding, range of this large group of natural products, many of which have been used in folk medicines, including some with potentially beneficial cytotoxic activity. The majority of the known quinoline alkaloids contain substituted quinoline, 2-quinolone, furoquinoline, dihydrofuroquinoline and dihydropyranoquinoline structures. Each of these quinoline alkaloid types can be further grouped, according to the regioselectivity (and degree) of enzyme-catalysed aromatic hydroxylation occurring at the unsubstituted carbocyclic ring, to give mainly mono-, di-, or tri-substituted alkaloids. Hydroxylation, often followed by alkylation, can produce the corresponding alkaloids bearing hydroxy, methoxy, or methylenedioxy groups.
In 1968, the detection of the first arene oxide metabolite (naphthalene 1,2-oxide), as an intermediate in the aromatic hydroxylation of naphthalene by Jerina and Daly at the National Institutes of Health (NIH), Bethesda,2 proved to be a seminal discovery in the context of elucidating the mechanism of enzyme-catalysed aromatic hydroxylation and its relevance to the NIH Shift.3 Later results, obtained from a biosynthetic study by Grundon,4 led to the suggestion that hydroxylation of aromatic heterocycles, including the parent unsubstituted furoquinoline alkaloid dictamnine, might similarly occur via monooxygenase-catalysed epoxidation of the benzo ring to give an arene oxide intermediate, dictamnine 7,8-oxide (A). Although this arene oxide has not yet been isolated as a plant metabolite, it was assumed that its formation as a biosynthetic intermediate, followed by isomerisation, could yield the corresponding phenols and other substituted quinoline alkaloids. These biosynthetic proposals4 have recently been supported by the synthesis of the relatively stable dictamnine 7,8-oxide (A), from the corresponding cis-7,8-dihydrodiol bacterial metabolite.5 Arene oxide A was in turn readily converted into the corresponding monosubstituted phenol, 8-hydroxydictamnine (robustine), and other furoquinoline alkaloids including 8-methoxydictamine (γ-fagarine).5
Dioxygenase-catalysed aromatic hydroxylation of dictamnine was also proposed as a biosynthetic route to other quinoline alkaloids.4 This possibility was realised by a bacterial dioxygenase-catalysed cis-dihydroxylation of dictamnine at the 7,8-bond to yield a cis-dihydrodiol metabolite, followed by a bacterial cis-diol dehydrogenase-catalysed dehydrogenation to yield 7,8-dihydroxydictamnine. Dimethylation of this catechol using diazomethane provided a chemoenzymatic route (and possible biosynthetic route) to the disubstituted quinoline alkaloid 7,8-dimethoxydictamnine (skimmianine).5
Epoxidation of a 3,3-dimethylallyl (prenyl) group, to give C-prenyl and O-prenyl epoxides, is a further type of biocatalytic oxidation found in quinoline alkaloid biosynthesis. Monooxygenase-catalysed formation of prenyl epoxides has been postulated, as a common biosynthetic route to transient intermediates, during the formation of 2-quinolone, furoquinoline, dihydrofuroquinoline and dihydropyranoquinoline alkaloids.1 The latter types of quinoline alkaloids are assumed to result, mainly, from enzyme-catalysed epoxide hydrolysis to yield vic-diols, and intramolecular cyclisation to form dihydrofurans and dihydropyrans. The C-prenyl epoxide (R)-preorixine (B)6,7 and O-prenyl epoxide (R)-anhydroevoxine (C)8,9 are thus among the relatively few stable quinoline alkaloid epoxides to have been isolated from plants. This introduction has emphasised the important roles that enzyme-catalysed epoxidation, vic-diol formation and arene hydroxylation can play in the biosynthesis of chiral quinoline alkaloids.
It has become evident that while most quinoline alkaloids isolated from plants are chiral, as a result of enzyme-catalysed oxidations, not all are enantiopure. Thus, for example, recent studies of the fourteen chiral quinoline alkaloids, isolated from Choisya ternata, showed that ee values covered the entire range (0-100%).9 Furthermore, in the majority of cases, neither the absolute configurations nor the ee values of chiral quinoline alkaloids, isolated from this and other plants, have been determined. In view of the increasing evidence of the potentially useful biological activity of many quinoline alkaloids, in both animal and microbial systems, it is desirable to have suitable methods for the synthesis of enantiopure or highly enantioenriched members, and for assignment of their absolute configurations.
The chemical synthesis of a series of quinoline alkaloids, using asymmetric epoxidation as a key step, was first reported more than 40 years ago.10-13 Unfortunately, the enantioselectivity of asymmetric epoxidation achieved during these early studies, using (+)-peroxycamphoric acid, was very poor. The resulting quinoline alkaloids were consistently isolated with low enantiomeric excess (2-10% ee) and optical rotation values ([α]D < 5). At that time, the reliability of methods available for purification, and unequivocal determination of absolute configurations of small quantities of chiral quinoline alkaloids having such low ee and [α]D values, was rather limited. It was therefore not surprising to find that our current, and earlier synthetic studies on single enantiomer quinoline alkaloids,14-16 have shown that many of these earlier assignments,10-13 based on low ee and [α]D values, were incorrect.
In the absence of reported synthetic routes to single enantiomer quinoline alkaloids, we earlier synthesised the nine unsubstituted chiral quinoline alkaloids platydesmine E, platydesminium methiodide F, edulinine G, geibalansine H, O-acetylgeibalansine I, geibalansinium methiodide J, ribalinine K, ψ-ribalinine L and araliopsine M14,15 and one monosubstituted alkaloid lunacridine N,16 in enantiopure form. The synthetic methods employed involved, mainly, resolution of the MTPA esters of trans-bromohydrin precursors by chromatography including preparative HPLC. A major objective of the present study was to synthesise a wider range of enantiopure (or highly enantioenriched) monosubstituted and 7,8-disubstituted alkaloids by modern catalytic asymmetric oxidation approaches, that would not involve chromatographic separation of diastereoisomers, and to use unequivocal methods for the determination of their absolute configurations.
RESULTS AND DISCUSSION
The acyclic alkene (glycolone) 1 bearing an OMe substituent at C-8 was synthesised by the literature route.17 Treatment of glycolone 1 with NBS in diethyl ether yielded the bromodihydropyran 2 as the major product (65%) which was readily separated from the minor isomeric bromodihydrofuran 3 by flash chromatography (Scheme 1). Dehydrobromination of compound 2 using sodium methoxide gave pyran 4 in quantitative yield. Asymmetric epoxidation of alkene 4 first involved the formation of the Mn(III)-(R,R)- and -(S,S)-salen catalysts with NaOCl as oxidant using the reported method.18,19 This chiral epoxidation reagent had been used earlier on 2,2-dimethylchromene to give the corresponding epoxide derivative in enantiopure form (>99% ee).19 Alkene 4, also having a 2,2-dimethyl (2H)-pyran ring, was similarly oxidized using NaOCl and Mn(III)-(R,R)-salen catalyst. A small proportion of the crude product, containing the required (3R,4R)-epoxide 5 ([α]D +121, CHCl3) was obtained after PLC purification (ca: 19% yield). The opposite (3S,4S) enantiomer of epoxide 5 ([α]D -125, CHCl3; 20% yield) was obtained after, using the corresponding (S,S)-salen catalyst, but again in low yield after chromatography.
Suspecting that a significant amount of relatively unstable (3R,4R)-epoxide 5 had decomposed during the PLC purification, a major fraction of the crude asymmetric epoxidation product, was catalytically hydrogenated (Pd-C in MeOH) to yield (R)-9-methoxygeibalansine 6 ([α]D -23, EtOH) in good yield (83% from alkene 4). Similarly, (S)-9-methoxygeibalansine 6 ([α]D +24, EtOH) was isolated in 89% yield from the crude (3S,4S)-epoxide 5. Both (R)- and (S)-9-methoxygeibalansine 6 were found to be enantiopure (>98% ee) by NMR analysis of their (+)-(α)-methoxy-(α)-trifluoromethylphenylacetates (MTPA esters). Thus, in common with the earlier asymmetric epoxidation of 2,2-dimethylchromene, 19 an enantiopure product was obtained from the epoxidation of the (2H)-pyran 4.
Although 9-methoxygeibalansine 6 has not yet been isolated as a plant alkaloid, the (R) enantiomer was used as a precursor of the alkaloids (R)-isobalfourodinium methiodide 7 (6→7), (R)-balfourolone 8 (7→8), (R)-isobalfourodine 9 (6→9), (S)-ψ-balfourodine 10 (9→10) and (R)-ψ-isobalfourodine 11 (9→11) (Scheme 2). A similar series of stereochemical correlation reactions was carried out using (S)-9-methoxygeibalansine 6 and had been used earlier for the corresponding unsubstituted (without a methoxy group at C-8) quinoline alkaloids i.e. geibalansine H → geibalansinium methiodide J (cf: 6→7), geibalansinium methiodide J → edulinine G (cf: 7→8), geibalansine H → ribalinine K (cf: 6→9), ribalinine K → araliopsine M (cf: 9→10), and ribalinine K → ψ-ribalinine L (cf: 9→11).15
N-Methylation of (-)-(R)-9-methoxygeibalansine 6 was achieved by stirring a solution of MeI in benzene for three days at ambient temperature. A precipitate of (R)-isobalfourodinium methiodide salt 7 ([α]D -14, MeOH) was filtered off and dried (Scheme 2). The (S)-isobalfourodinium methiodide ([α]D +15, MeOH) was similarly obtained from (+)-(S)-9-methoxygeibalansine 6 but the isolated yield for either enantiomer was low (<30 %). Treatment of (-)-(R)-isobalfourodinium methiodide 7 with aqueous ammonia resulted in nucleophilic attack of hydroxide at the immonium group and ring opening occurred to give (R)-balfourolone 8 ([α]D +31, MeOH) in good yield (83%). Under identical conditions, (+)-(S)-isobalfourodinium methiodide 7 gave (S)-balfourolone 8 ([α]D -29, MeOH).
While reaction of (-)-(R)- or (+)-(S)-9-methoxygeibalansine 6 with MeI in benzene solution gave relatively poor yields of (R)- or (S)-isobalfourodinium methiodide 7, refluxing compound (-)-6 or (+)-6 with an excess of neat MeI gave (R)-isobalfourodine 9 ([α]D -13, MeOH) or (S)-isobalfourodine 9 ([α]D +12, MeOH) in excellent yields (88-96%) (Scheme 2).
The dihydropyran ring of (-)-(R)-isobalfourodine 9 was found to open in the presence of NaOMe in DMF solvent and was followed by spontaneous cyclization to yield the angular dihydrofuran alkaloid (S)-ψ-balfourodine 10 ([α]D +29, EtOH; 74% yield). However, (R)-ψ-isobalfourodine 11 ([α]D -25, EtOH; 95% yield) was obtained when ring opening and cyclization of compound (-)-9 occurred in the presence of NaOH in MeOH solvent. The dihydrofuran 10 was assumed to have been formed with inversion of configuration, and dihydropyran 11 with retention of configuration, both originating from a common transient (R)-prenyl epoxide intermediate 12, as shown in Scheme 2. This mechanistic sequence had been proposed earlier20 using isobalfourodine 9 having a low ee value (<5%), and is now confirmed by our rigorous stereochemical assignments of the enantiopure alkaloids 7-11.
The absolute configurations of the homochiral alkaloids 7-11 were determined in an unequivocal manner by several methods including comparison of their CD spectra with those of the corresponding unsubstituted alkaloids of known configuration.15 In this context, the CD spectra of compounds (-)-(R)-9-methoxygeibalansine 6, (-)-(R)-isobalfourodinium methiodide 7, (+)-(R)-balfourolone 8, (-)-(R)-isobalfourodine 9, (+)-(S)-ψ-balfourodine 10 and (-)-(R)-ψ-isobalfourodine 11 were found to be very similar to the corresponding enantiomers of alkaloids of established configuration but devoid of a MeO substituent in the carbocyclic ring (geibalansine H, geibalansinium methiodide J, edulinine G, ribalinine K, araliopsine M, ψ-ribalinine L).15 The (R) absolute configuration (and >98% ee value) of (+)-balfourolone 8 was also confirmed by ozonolysis to yield the lactone 13 of established (R) absolute configuration followed by chiral stationary phase-GC-MS (CSP-GC-MS) analysis, a method successfully applied to other chiral quinoline alkaloids.9,21
We have employed an asymmetric oxidation approach to the synthesis of two chiral quinoline alkaloids (>90% ee).15 Thus AD-mix-β was employed as oxidant in the dihydroxylation of an O-prenyl group to give the chiral furoquinoline alkaloid (R)-evoxine (D, 93% ee), a precursor of the derived (R)-anhydroevoxine (C),9 and (R)-edulinine (G, 94% ee), a precursor of (S)-platydesmine (E) and (S)-platydesminium methiodide (F).15 As a logical extension, asymmetric dihydroxylations of alkenes 14, 16 and 18 were also carried out using commercially available AD-mix-α and AD-mix-β (Scheme 3). AD-mix contained a catalytic quantity of osmium tetroxide in the presence of K3Fe(CN)6 and the chiral ligands hydroquinine (1,4-phthalazinediyl diether, [DHQ]2PHAL; mix-α) or hydroquinidine (1,4-phthalazinediyl diether, [DHQD]2PHAL; mix-β); all dihydroxylations were conducted in the presence of methanesulfonamide in tert-butanol:water (1:1) at 0 oC.
The AD-mix-β reagent yielded the corresponding vicinal diol products (R)-nororixine 15 ([α]D +23, EtOH; 85% ee), (R)-hydroxylunidine 17 ([α]D +26, EtOH; 76% ee), and (R)-orixine 19 ([α]D +52, EtOH; 70% ee). The alternative quinoline alkaloid enantiomers, (S)-15 ([α]D -22, EtOH), (S)-17 ([α]D -26, EtOH), and (S)-19 ([α]D -51, EtOH) having similar ee values were obtained when using AD-mix-α (Scheme 3). The enantiopurity values of alkaloids 15, 17 and 19 (70-85% ee before recrystallization), and their absolute configurations, were determined by ozonolysis followed by CSP-GC-MS analysis of the resulting lactone 13, using the method reported earlier for other chiral quinoline alkaloids.9,21 The ee value of orixine 19, obtained using AD mix, was found to increase (70→>95% ee) upon recrystallisation. A similar increase in ee value (85→>90% ee) was observed for (R)-nororixine 15 which was then used as precursor of the alkaloids (S)-isopteleflorine 22, (S)-O-acetylisopteleflorine 23, (S)-O-methylhydroxylunium methiodide 24 and (S)-hydroxylunine 25 (Scheme 4).
Reaction of (R)-nororixine 15 with acetoxyisobutryl bromide yielded the (R) enantiomer of the corresponding bromoacetate derivative 20. Treatment with sodium methoxide in THF then yielded the dihydrofuran, (S)-isopteleflorine 22 ([α]D +19, EtOH; 90% yield). Treatment of the (S)-isopteleflorine 22 with Ac2O in pyridine containing a trace of DMAP gave the corresponding acetate, (S)-O-acetylisopteleflorine 23 ([α]D -6, CHCl3; 93% yield). It is assumed that the (S)-dihydrofuran 22 was formed via the (R)-prenyl epoxide intermediate 21 and that, in common with the prenyl epoxide 12 which formed (S)-ψ-balfouridine 10, it would also spontaneously cyclise to a dihydrofuroquinoline alkaloid, but in this case to the (S)-linear isomer 22.
Methylation of (S)-isopteleflorine 22, using MeI in EtOH, yielded (S)-O-methylhydroxylunium methiodide 24 ([α]D +16, EtOH) in remarkably good yield (91%) compared with the earlier methylation of 9-methoxygelbalansine 6 under similar conditions to yield the (R)-pyridinium salt 7 (<30% yield, Scheme 2). O-Demethylation of the (S)-quaternary ammonium iodide 24 to yield (S)-hydroxylunine 25 ([α]D +25, EtOH; 85% yield) was observed when heated in pyridine solvent.
Each of the alkaloids, derived from (R)-nororixine 15 (91% ee), i.e. (S)-isopteleflorine 22, (S)-O-acetylisopteleflorine 23, (S)-O-methylhydroxylunium methiodide 24, and (S)-hydroxylunine 25, were purified by recrystallisation, and were assumed to be virtually enantiopure (>95% ee). This conclusion was supported by their optical rotation values being comparable or higher than those reported for the naturally occurring alkaloids extracted from plant sources. The absolute configurations and % ee values of (+)-(R)-nororixine 15, (+)-(R)-hydroxylunidine 17, (+)-(R)-orixine 19, (+)-(S)-isopteleflorine 22 and (+)-hydroxylunine 25 were confirmed by ozonolysis and CSP-GC-MS analysis of the resultant lactone 13. The absolute configurations of (-)-(S)-O-acetylisopteleflorine 23 and (+)-(S)-O-methylhydroxylunium methiodide 24 were, in turn, derived by stereochemical correlation with (+)-(S)-isopteleflorine 22.
The final phase of this programme involved a comparison of the absolute configurations of the twelve enantiopure alkaloids, synthesised and configurationally assigned during the current study, with those reported earlier, and determination of the unknown configurations of the chiral alkaloids isolated from plant sources (Table 1). Absolute configurations had not been reported earlier for (+)-9-methoxygeibalansine 6, (+)-isobalfourodinium methiodide 7, (+)-nororixine 15, (+)-hydroxylunidine 17, (+)-isopteleflorine 22, (+)-O-acetylisopteleflorine 23, and (+)-hydroxylunine 25. The earlier assignments for (+)-balfourolone 8,12 (-)-isobalfourodine 9,12 (-)-ψ-balfouriodine 10,20 (+)-ψ-isobalfourodine 11,20 (-)-orixine 1911 and (+)-O-methylhydroxylunium methiodide 2422 were wrong but the absolute configuration of (+)-orixine 19 was later reassigned correctly (Table 1).6,12
CONCLUSION
The chiral quinoline alkaloids 7-11, 15, 17, 19, 22-25 have been synthesised with >90% ee values, using asymmetric oxidation methods involving both stable (5) and unstable (12 and 21) epoxide intermediates. The previously unknown absolute configurations of six alkaloids, 7, 15, 17, 22, 23 and 25, have been determined using unequivocal methods and the incorrectly reported configurations of the other six alkaloids, 8-11, 19, 24, have been reassigned. The availability of enantiopure quinoline alkaloids, using the described synthetic methods, will be of value in (i) elucidating the biosynthetic pathways of these and other alkaloids and (ii) evaluating the pharmacological potential of individual alkaloid enantiomers.
EXPERIMENTAL
NMR (1H and 13C) spectra were recorded on Bruker Avance DPX-300 and DPX-500 instruments and mass spectra were run at 70 eV, on a VG Autospec Mass Spectrometer, using a heated inlet system. Accurate molecular weights were determined by the peak matching method, with perfluorokerosene as the standard. Elemental microanalyses were carried out on a Perkin Elmer 2400 CHN microanalyser. For optical rotation ([α]D) measurements (ca. 20 °C, 10-1 deg cm2 g-1), a Perkin Elmer 341 polarimeter was used. Electronic circular dichroism (ECD) spectra were recorded on a Jasco J-720 instrument in acetonitrile solvent.
Flash column chromatography and Preparative Layer Chromatography (PLC) were performed on Merck Kieselgel type 60 (250-400 mesh) and PF254/366 respectively. Merck Kieselgel type 60F254 analytical plates were used for TLC. The (R,R)- and (S,S)-Mn salen ligands were prepared in accordance with the literature method23 using commercially available reagents (Aldrich Chemical Co.). Chemically synthesised chiral alkaloids 7-11, 15, 17, 19, 22-25 were found to have identical 1H-NMR spectra to those reported in the literature. Additional matching spectra (NMR, IR, ECD) have been included. Absolute configurations and ee values of chiral quinoline alkaloids 8, 15, 17, 19 and 22 were determined by ozonolysis and CSP-GC-MS analysis of the resulting lactone 13 by the method described earlier.9,21
4,8-Dimethoxy-3-(3'-methylbut-2'-enyl)-2-quinolinone (Glycolone) 1
Glycolone 1 (0.890 g, 84%) was synthesised using the literature method.17 Rf 0.48 (2% MeOH in CHCl3), mp 110-112 °C (from iPr2O) (Lit.,17 mp 119-120 °C); νmax 3407 cm-1 (OH), 1643 cm-1 (C=O); δH (300 MHz, CDCl3) 1.69 (3H, s, CMe), 1.80 (3H, s, CMe), 3.39 (2H, d, J1',2' 6.7, H-1'), 3.92 (3H, s, OMe), 3.96 (3H, s, OMe), 5.27 (1H, t, J2',1' 6.6, H-2'), 6.94 (1H, d, J5,6 7.9, H-5), 7.14 (1H, dd, J6,7 8.2, J6,5 7.9, H-6), 7.35 (1H, d, J7,6 8.2, H-7), 9.10 (1H, br s, NH).
3-Bromo-3,4-dihydro-5,9-dimethoxy-2,2-dimethyl-[2H]-pyrano[2,3-b] quinoline 2
N-Bromosuccinimide (0.326 g, 1.83 mmol) was added in portions to a solution of glycolone 1 (0.50 g, 1.83 mmol) in dry Et2O (80 cm3) and the mixture stirred at ambient temperature for 20 min. The precipitated succinimide by-product was removed, by filtration, and the solvent distilled off under reduced pressure to give the crude products as a yellow gum. Purification by flash chromatography (CHCl3) gave the desired product 2 as a colourless oil which solidified to a white solid (0.419 g, 65%), Rf 0.25 (CHCl3), mp 154-156 °C (from iPr2O/MeOH); (Found: M+, 351.0460 C16H18NO379Br requires M+, 351.0470); δH 300 MHz, CDCl3) 1.54 (3H, s, CMe), 1.63 (3H, s, CMe), 3.36 (1H, dd, J4,4' 17.4, J4,3 8.8, H-4), 3.60 (1H, dd, J4',4 17.5, J4',3 5.5, H'-4), 4.01 (6H, s, 2 x OMe), 4.31 (1H, dd, J3,4 8.8, J3,4' 5.5, H-3), 6.97 (1H, d, J6,7 7.6, H-6), 7.30 (1 H, dd, J7,6 7.6, J7,8 8.7, H-7), 7.50 (1H, d, J8,7 8.7, H-8); m/z 353 (M+, 81Br, 66%), 351 (M+, 79Br, 64%), 272 (82%) and 216 (100%).
Further elution gave the isomeric 2-(1-bromo-1-methylethyl)-2,3-dihydro-4,8- dimethoxyfuro [2,3-b] quinoline 3 as white needles (0.225 g, 35%); Rf 0.19 (CHCl3); mp 140-141°C (from iPr2O/MeOH); (Found: M+, 351.0465 C16H18NO379Br requires M+, 351.0470); δH (300 MHz, CDCl3) 1.76 (3H, s, CMe), 1.93 (3H, s, CMe), 3.73-3.80 (2H, m, H-3, H-3'), 4.00 (3H, s, OMe), 4.26 (3H, s, OMe), 4.79 (1H, dd, J2,3 , J2,3' 7.7, H-2), 6.84 (1H, d, J5,6 7.7, H-5), 7.24 (1H, dd, J6,5 7.7, J6,7 8.3, H-6), 7.62 (1H, d, J7,6 8.3, H-7); m/z 353 (M+, 81Br, 20%), 351 (M+, 79Br, 20%), 232 (97%) and 200 (100%).
5,9-Dimethoxy-2,2-dimethyl-[2H]-pyrano[2,3-b]quinoline 4
Sodium methoxide (0.40 g, 7.4 mmol) was added in one portion to a solution of the bromopyrano derivative 2 (1.0 g, 2.84 mmol) in dry MeOH (200 cm3). The mixture was refluxed under nitrogen for 1.5 h and the methanol removed under reduced pressure. The residue was dispersed in water (50 cm3) and extracted with CHCl3 (3 x 30 cm3). The combined extract was dried (MgSO4) and the solvent evaporated to yield the pure title compound 4 as a viscous colourless oil which solidified to a white solid on standing (0.77 g, 98%); Rf 0.85 (1% MeOH in CHCl3; mp 71-72 °C (from pentane); (Found: M+, 271.1209 C16H17NO3 requires M+, 271.1208); δH (300 MHz, CDCl3) 1.52 (6H, s, CMe2), 4.00 (3H, s, OMe), 4.00 (3H, s, OMe), 5.86 (1H, d, J3,4 10.0, H-3), 6.73 (1H, d, J4,3 10.0, H-4), 6.96 (1H, d, J6,7 7.9, H-6), 7.26 (1H, dd, J7,6 7.9, J7,8 8.6, H-7), 7.49 (1H, d, J8,7 8.6, H-8); m/z 271 (M+, 100%) and 256 (M+-Me, 98%).
(+)-(3R,4R)- and (-)-(3S,4S)-3,4-Epoxy-3,4-dihydro-5,9-dimethoxy-2,2-dimethyl-[2H]-pyrano [2,3-b] quinoline 5
An aqueous solution of Na2HPO4 (0.05 M, 1.2 cm3) was added to an aqeous solution of NaOCl (0.77 M, 3 cm3). The pH of the resulting buffered solution (~ 0.55 M in NaOCl) was adjusted, as required, to pH 11.3 by addition of aqueous sodium hydroxide (1 M) or aqueous hydrochloric acid (1 M). The solution, cooled to 0 °C, was added in one portion to a solution of Mn-salen R,R-catalyst (0.05 mmol) and alkene 4 (0.32 g, 1.18 mmol) in CH2Cl2 (2 cm3) kept at 0 °C. The two phase mixture was stirred, at rt, and the progress of the reaction was followed by TLC analysis. On completion of the reaction (3.5 h), hexane (10 cm3) was added to the mixture, the organic phase separated, washed successively with water (2 x 5 cm3) and saturated sodium chloride solution (5 cm3). The organic solution was dried (Na2SO4), the solvent evaporated under reduced pressure, and the crude product purified by PLC (CHCl3: EtOAc; 4:1) to give epoxide 5 (0.064 g, 19%) as a pale yellow viscous oil (Found: M+, 287.1159 C16H17NO4 requires M+, 287.1158); [α]D +121 (c 1.6, CHCl3); δH (300 MHz, CDCl3) 1.41 (3H, s, CMe), 1.66 (3H, s, CMe), 3.58 (1H, d, J3,4 4.3, H-3), 4.00 (3H, s, OMe), 4.13 (3H, s, OMe), 4.47 (1H, d, J4,3 4.1, H-4), 7.01 (1H, d, J6,7 7.8, H-6), 7.33 (1H, dd, J7,6 7.8, J7,8 8.3, H-7), 7.54 (1H, d, J8,7 8.3, H-8); m/z 287 (M+, 100%), 258 (47%) and 83 (84%); >95% ee.
(-)-(3S,4S)-3,4-epoxy-3,4-dihydro-5,9-dimethoxy-2,2-dimethyl-[2H]-pyrano[2,3-b]quinoline 5 : With Mn-salen S,S-catalyst (20% yield); [α]D -125; >95% ee.
(+)-(S) and (-)-(R)-9-Methoxygeibalansine 6
A solution of crude (-)-(3S,4S)-epoxide (-)-5 (0.176 g, 0.61 mmol) in MeOH (15 cm3) containing 10% Pd/C (0.015g) was stirred overnight under a hydrogen atmosphere. The reaction mixture was filtered through a pad of celite and the solvent evaporated under reduced pressure to yield a viscous oil. Purification by PLC (5% MeOH in CHCl3) gave (+)-(S)-9-methoxygeibalansine 6 as a colourless oil (0.121 g, 89% for 2 steps), with 1H-NMR data similar to that of a racemic sample;24 [α]D +24 (c 2.27, EtOH); δH (300 MHz, CDCl3) 1.42 (6H, s, CMe2), 2.94 (1H, dd, J4,4'17.2, J4,3 6.5, H-4), 3.16 (1 H, dd, J4',4 17.2, J4',3 5.0, H-4'), 3.92 (1H, dd, J3,4 6.5, J3,4' 5.0, H-3), 3.97 (3H, s, OMe), 3.99 (3H, s, OMe), 6.95 (1H, d, J6,7 7.7, H-6), 7.26 (1H, dd, J7,6 7.7, J7,8 8.3, H-7), 7.47 (1H, d, J8,7 8.3, H-8); m/z 289 (M+, 11%) and 83 (100%); ECD Δε -2.18 (324 nm), Δε +2.62 (257 nm), Δε -11.8 (242 nm), Δε -16.5 (214 nm).
(-)-(R)-9-Methoxygeibalansine 6: 83% yield; [α]D -23 (c 1.28, EtOH).
(+)-(S)-and (-)-(R)-Isobalfourodine 9
A solution of (+)-(S)-9-methoxygeibalansine 6 (0.080 g, 0.28 mmol) in MeI (20 cm3, 0.32 mol) was refluxed overnight. Removal of the solvent under reduced pressure yielded the crude product. Purification by PLC gave (+)-(S)-isobalfourodine 9 as a white solid (0.077 g, 96%); mp 174-175 °C (from iPr2O/MeOH, needles) (Lit.,24 mp (rac.) 184-186 °C); [α]D +13 (c 0.83, EtOH); νmax 3318 cm-1 (OH), 1605 cm-1 (C=O); δH (300 MHz, CDCl3) 1.38 (3H, s, CMe), 1.47 (3H, s, CMe), 2.84 (1H, dd, J4,4' 17.0, J4,3 5.2, H-4), 2.96 (1H, dd, J4',4 17.0, J4',3 4.8, H-4'), 3.78-3.87 (7H, m, NMe, OMe, H-3), 7.03 (1H, d, J8,7 7.3, H-8), 7.18 (1H, dd, J7,6 8.1, J7,8 7.3, H-7), 7.95 (1H, d, J6,7 8.1, H-6); m/z 289 (M+, 58%) and 218 (100%); ECD Δε +14.5 (323 nm), Δε +1.90 (264 nm), Δε -7.42 (245 nm), Δε +10.26 (208 nm).
(-)-(R)-Isobalfourodine 9: 88% yield; [α]D -13 (c 0.6, EtOH).
(+)-(S)-ψ-Isobalfourodine 11
(+)-(S)-Isobalfourodine 9 (0.051 g, 0.176 mmol) was taken up in MeOH (4 cm3), aqueous sodium hydroxide (30%, 4 cm3) was added and the solution stirred under reflux for 7 h. The reaction mixture was cooled, diluted with water and the product extracted into CHCl3 (3 x 15 cm3). The extract was dried (MgSO4) and the solvent removed to yield the crude product as a colourless viscous oil. Purification by PLC (5% MeOH in CHCl3) gave (+)-(S)-ψ-isobalfourodine 11 as a white solid (0.051 g, 97%); mp 189-191°C (from iPr2O/ MeOH) (Lit.,20 mp [rac.] 190-191°C); [α]D +25 (c 1.04, EtOH); νmax 3301 cm-1 (OH), 1628 cm-1 (C=O); δH (300 MHz, CDCl3) 1.38 (3H, s, CMe), 1.44 (3H, s, CMe), 2.64 (1H, br s, OH), 2.71 (1H, dd, J4,4' 18.0, J4,3 5.6, H-4), 2.91 (1H, dd, J4',4 17.9, J4',3 5.0, H-4'), 3.85-3.88 (7H, m, NMe, OMe, H-3), 7.01 (1H, d, J10,9 7.7, H-10), 7.13 (1H, dd, J9,10 7.7, J9,8 7.9, H-9), 7.61 (1H, d, J8,9 7.9, H-8); m/z 289 (M+, 47%), 230 (40%), 218 (41%) and 69 (100%); ECD Δε -0.33 (323 nm), Δε -4.94 (291 nm), Δε -12.01 (254 nm), Δε +1.42 (226 nm).
(-)-(R)-ψ-Isobalfourodine 11: 95% yield; [α]D -25 (c 1.35, EtOH).
(+)-(S)- and (-)-(R)-ψ-Balfourodine 10
A solution of (-)-(R)-isobalfourodine 9 (0.027 g, 0.09 mmol.) in DMF (3 cm3) was stirred, at rt overnight, with sodium methoxide (0.075 g, 1.39 mmol). Water (20 cm3) was added and the product extracted into CHCl3 (3 x 15 cm3). The extract was dried (MgSO4), concentrated under reduced pressure, and the crude product purified by PLC (5% MeOH in CHCl3) to give (S)-ψ-balfourodine as colourless viscous oil (0.020 g, 74%); [α]D +40 (c 1.36, MeOH) (Lit.,12; [α]D +3); νmax. 3371 cm-1 (OH), 1655 cm-1 (C=O); δH (300 MHz, CDCl3), 1.26 (3H, s, CMe), 1.37 (3H, s, CMe), 2.11 (1H, br s, OH), 3.15-3.94 (2H, m, CH2), 3.88-3.94 (7H, m, NMe, OMe, H-2), 7.06 (1H, d, J9,8 7.3, H-9), 7.15 (1H, dd, J8,9 7.3, J8,7 7.8, H-8), 7.39 (1H, d, J7,8 7.8, H-7); m/z 289 (M+, 83%) and 230 (100%); ECD Δε -11.26 (299 nm), Δε -8.18 (259 nm), Δε -17.35 (237 nm), Δε +33.86 (213 nm).
(-)-(R)-ψ-Balfourodine (-)-10: 69% yield; [α]D -39 (c 1.4, EtOH).
(+)-(S)-and (-)-(R)-Isobalfourodinium methiodide 7
A solution of (+)-(S)-9-methoxygeibalansine 6 (0.072 g, 0.25 mmol) in dry benzene (2 cm3) and iodomethane (2 cm3, 0.032 mol) was kept at rt for 3 days. The precipitate formed was separated by filtration and washed, on the filter paper, with dry benzene. (+)-(S)-Isobalfourodinium methiodide 7 was obtained as a cream coloured solid (0.028 g, 26%); Rf 0.21 (10% MeOH in CHCl3); mp 113-115 °C; (Found: M+, 289.1315. C17H22NO4I -MeI requires M+, 289.1314); [α]D +15 (c 1.08, MeOH); δH (300 MHz, CDCl3), 1.57 (3H, s, CMe), 1.75 (3H, s, CMe), 3.26 (1H, dd, J4,4' 17.2, J4,3 3.8, H-4), 3.67 (1H, dd, J4',4 17.3, J4',3 4.1, H-4'), 4.05 (3H, s, NMe), 4.31 (3H, s, OMe), 4.36 (3H, s, OMe), 4.25-4.40 (1H, m, H-3), 7.37 (1H, d, J6,7 7.9, H-6), 7.58 (1H, dd, J7,6 7.9, J7,8 8.2, H-7), 7.80 (1H, d, J8,7 8.2, H-8); m/z 289 (M+-MeI, 60%) and 218 (100%); ECD Δε +2.30 (324 nm), Δε -0.63 (293 nm), Δε +13.33 (253 nm), Δε -40.26 (211 nm).
(-)-(R)-Isobalfourodinium methiodide 7: 14% yield; [α]D -14 (c 0.64, MeOH).
(-)-(S)- and (+)-(R)-Balfourolone 8
(+)-(S)-Isobalfourodinium methiodide 7 (0.022 g, 0.05 mmol) was taken up in aqueous ammonia (33% w/w, 5 cm3) and the mixture stirred at rt for 3 h. The reaction mixture was diluted with water (10 cm3), saturated with sodium chloride, and the solution extracted with CHCl3 (3 x 10 cm3). The organic extract was washed with water (10 cm3), dried (MgSO4), and the solvent removed under reduced pressure. The crude product obtained was purified by PLC (5% MeOH in CHCl3) to give (-)-(S)-balfourolone 8 as a colourless viscous oil (0.014 g, 85%); [α]D -29 (c 1.27, MeOH) (Lit.,25; [α]D +32); νmax 3399 cm-1 (OH), 1630 cm-1 (C=O); δH (500 MHz, CDCl3), 1.31 (3H, s, CMe), 1.31 (3H, s, CMe), 2.72 (1H, dd, J1',1 13.7, J1,2' 10.3, H-1), 3.10 (1H, dd, J1',1 13.7, J1',2' 2.1, H-1'), 3.59 (1H, dd, J2',1' 10.3, J2',1 2.1, H-2'), 3.92 (3H, s, NMe), 3.93 (3H, s, OMe), 3.98 (3H, s, OMe), 5.27 (1H, br s, OH), 7.09 (1 H, dd, J5,6 7.9, J5,7 1.1, H-5), 7.23 (1H, dd, J6,5 = J6,7 7.9, H-6), 7.46 (1H, dd, J7,6 7.9, J7,5 1.5, H-7); m/z 321 (M+, 0.6%), 303 (M+-H2O, 11%) and 262 (100%); ECD Δε -10.56 (286 nm), Δε +3.96 (260 nm), Δε -14.34 (242 nm), Δε 1.50 (227 nm).
(+)-(R)-Balfourolone (+) 11: 83% yield; [α]D +31 (c 0.84, MeOH).
(+)-(R)-Hydroxylunidine 17
The crude product obtained by the dihydroxylation of alkene 16 using AD mix-β on purification, by PLC (5% MeOH in CHCl3), afforded (+)-(R)-hydroxylunidine 17: (0.090 g, 81 %) colourless crystals; Rf 0.25; mp 120.5-121.5 °C (from EtOH) (Lit.,26 mp 124-125 °C); [α]D +29 (c 0.16, EtOH) (Lit.,26 [α]D +28, EtOH); δH (500 MHz, CDCl3) 1.30 (3H, s, CMe), 1.61 (3H, s, CMe), 2.60 (1H, s, OH), 2.69 (1H, dd, J1'a,1'b 13.9, J1'a,2' 10.2, H-1a'), 3.06 (1H, d, J1a',1b' 13.9, J1b',2' 2.0, H-1b'), 3.56 (1H, ddd, J2',1a' 10.2, J2',OH 3.5, J2',1'b 2.0, H-2'), 3.91 (3H, s, NMe), 3.92 (3H, s, OMe), 5.02 (1H, d, JOH,2' 3.5, CH2CHOH), 6.07 (2H, s, OCH2O), 6.87 (1H, d, J4,5 8.6, H-4), 7.40 (1H, d, J5,4 8.6, H-5); m/z 336 (M++1, 1 %), 276 (100%); ECD data: Δε +7.40 (301 nm), Δε -1.56 (261 nm), Δε +1.91 (215 nm), Δε -2.92 (204 nm).
(-)-(S)-Hydroxylunidine 17: With AD mix-α; [α]D –29 (c 0.92, EtOH).
(+)-(R)-Nororixine 15
The crude product formed by the dihydroxylation of alkene 14 using AD mix-β, on purification by PLC (6% MeOH-CHCl3), gave (+)-(R)-nororixine 15 (0.15 g, 70%); Rf 0.2; mp 198-199 °C (from EtOH) (Lit.,26 mp 199-200 °C); [α]D +24 (c 0.76, EtOH) (Lit.,26 [α]D +27, EtOH); δH (500 MHz, CDCl3) 1.24 (3H, s, CMe), 1.59 (3H, s, CMe), 2.64 (1H, s, OH) 2.67 (1H, dd, J1'a,1'b 14.3, J1'a,2' 10.0, H-1'a), 3.13 (1H, d, J1'b,1'a 14.2, H-1'b), 3.58 (1H, d, J2',1'a 9.8, H-2'), 3.97 (3H, s, OMe), 5.20 (1H, s, OH), 6.16 and 6.17 (2H, s, OCH2O), 6.85 (1H, d, J4,5 8.6, H-4), 7.33 (1H, d, J5,4 8.6, H-5); δC (125 MHz, CDCl3) 167.5, 164.0, 149.3, 133.5, 122.3, 119.3, 117.6, 113.5, 105.4, 103.0, 79.5, 73.2, 62.7, 27.6, 25.8, 24.3; ECD : Δε + 0.46 (296 nm), Δε -1.80 (262 nm), Δε +1.81 (212 nm).
(-)-(S)-Nororixine 15: With AD mix-α; [α]D -22 (c 0.8, EtOH).
(+)-(R)-Orixine 19
The dihydroxylation complex was generated by adding methane sulfonamide (0.035 g, 0.36 mmol) into a stirring solution of AD mix-β (0.5 g) in tert-butanol (2 cm3) and water (2 cm3) at 0 °C. Alkene 18 (0.10 g, 0.33 mmol) was added to the mixture and stirring was continued at 0 °C for 24 h. After completion of the reaction (TLC analysis), a saturated Na2S2O5 solution (1 cm3) was added and the mixture stirred for 1h at rt. The reaction mixture was saturated with NaCl and then extracted with EtOAc (3 x 20 cm3). The organic extract was dried (Na2SO4) and concentrated under reduced pressure. Purification by PLC (5% MeOH-CHCl3) afforded (+)-(R)-orixine 19 as a white solid (0.080 g, 73%); (Rf 0.2); mp 146-147 °C (from EtOAc-hexane) (Lit.,26 mp 153 °C); [α]D +64 (c 0.5, CHCl3) (Lit.,27 [α]D +83); δH (500 MHz, CDCl3) 1.33 and 1.36 (3H, s, CMe), 2.24 (1H, s, OH), 2.56 (1H, d, JOH,2' 4.4, OH), 2.83 (1H, dd, J1'a,1'b 13.7, J1'a,2' 10.2, H-1'a), 2.98 (1H, dd, J1'b,1'a 13.7, J1'b,2' 2.5, H-1'b), 3.67 (1H, ddd, J2',1'a 10.2, J2',OH 4.4, J2',OH 2.5, H-2'), 4.11 (1H, s, OMe), 4.12 (1H, s, OMe), 6.20 (2H, s, OCH2O), 7.06 (1H, d, J4,5 8.7, H-4), 7.48 (1H, d, J5,4 8.7, H-4); m/z 335 (40%), 246 (100); ECD: Δε +6.21 (254 nm), Δε + 3.23 (211 nm), Δε -2.34 (193 nm).
(-)-(S)-Orixine 19: With AD mix-α; [α]D -61 (c 0.52, CHCl3).
(-)-(R)-1'-Bromo-3'-(6-methoxy-8-oxo-8,9-dihydro[1,3]dioxolo[4,5-h]quinolin-7-yl)-1',1'-
dimethylpropyl acetate 20
To a solution of (+)-(R)-nororixine 15 (0.12 g, 0.374 mmol) in anhydrous MeCN (2 cm3) at 0 °C, 1-bromocarbonyl-1-methylethyl acetate (0.066 cm3, 0.449 mmol) was added. The mixture was stirred at 0 °C for 2 h and at rt for 2 h. Most of the MeCN was removed under reduced pressure, the residue taken up in EtOAc (15 cm3) and thoroughly washed with a solution of aqueous NaHCO3 (2.5%, 20 cm3). The aqueous basic layer was back-extracted with EtOAc (20 cm3). The organic layers were combined, dried (Na2SO4) and concentrated under reduced pressure to give the crude bromo acetate 20 as a yellow oil. Purification by PLC (50% EtOAc in hexane) gave (-)-(R)-bromoacetate 20 as colourless oil which solidified on standing (0.13 g, 76%); Rf 0.5; mp 137-138 °C (from CHCl3); [α]D - 4 (c 0.14, CHCl3); (Found: M+ 345.1219. C18H19NO6 requires 345.1212); δ H (500 MHz, CDCl3) 1.54 (3H, s, CMe), 1.63 (3H, s, CMe), 1.97 (3H, s, OCOMe), 3.49 (1H, dd, J3'a,3'b 15.8, J3'a,2' 6.7, H-3'a), 3.61 (1H, dd, J3'b,3'a 15.8, J3'b,2' 9.3, H-3'b), 4.24 (3H, s, OMe), 4.98 (1H, dd, J2', 3'b 9.3, J2',3'a 6.7, H-2'), 6.15 (2H, s, OCH2O), 6.94 (1H, d, J4,5 8.7, H-4), 7.59 (1H, d, J5,4 8.7, H-5); δ C (125 MHz, CDCl3) 170.2, 169.4, 147.8, 140.1, 133.9, 116.6, 115.6, 106.6, 102.1, 99.7, 84.0, 82.1, 58.3, 28.9, 22.3, 22.2, 20.8; m/z 345 (M+-HBr, 15%).
(+)-(S)-1'-Bromo-3'-(6-methoxy-8-oxo-8,9-dihydro[1,3]dioxolo[4,5-h]quinolin-7-yl)-1',1'-
dimethylpropyl acetate 20: [α]D +5 (c 0.31, CHCl3).
(+)-(S)-Isopteleflorine 22
To a solution of (-)-(R)-bromoacetate 20 (0.1 g, 0.235 mmol) in dry Et2O (30 cm3) anhydrous NaOMe (0.5 g) was added. After stirring the reaction mixture (24 h) at rt, it was filtered through a pad of celite to remove the inorganic salts. The filtrate was concentrated under reduced pressure and the residue purified by multiple elution PLC (75% EtOAc in hexane). (+)-(S)-Isopteleflorine 22 was obtained as white solid (0.064 g, 90%); Rf 0.3; mp 164-165 °C (from CHCl3) (Lit.,28 mp 163-166 °C); [α]D +19 (c 0.35, CHCl3) (Lit.,29 [α]D -18, CHCl3); Anal.Calcd for C16H17NO5: C, 63.4; H, 5.6; N, 4.6%. Found: C, 63.5; H, 5.5; N, 4.3 ; δH (500 MHz, CDCl3) 1.26 (3H, s, CMe), 1.41 (3H, s, CMe), 3.54 (1H, dd, J7a,7b 15.4, J7a,8 8.8, H-7a), 3.56 (1H, dd, J7b,7a 15.4, J7b,8 7.7, H-7b), 4.21 (3H, s, OMe), 4.62 (1H, dd, J8,7a 8.8, J8,7b 7.8, H-8), 6.13 (1H, d, J 2.4, OCH2O), 6.15 (1H, J 2.4, OCH2O), 6.93 (1H, d, J4,5 8.7, H-4), 7.58 (1H, d, J5,4 8.7, H-5); δC (125 MHz, CDCl3) 24.7, 26.5, 29.3, 58.7, 71.9, 86.8, 100.6, 102.5, 107.0, 116.4, 117.1, 134.2, 140.4, 148.1, 159.7, 169.8; m/z 303 (60%); ECD: Δε -0.94 (250 nm), Δε +2.98 (224 nm), Δε +2.02 (199 nm).
(-)-(R)-Isopteleflorine 22: [α]D -18 (c 0.72, CHCl3).
(-)-(S)-O-Acetylisopteleflorine 23
A mixture of (+)-(S)-isopteleflorine 22 (0.01 g, 0.033 mmol), acetic anhydride (1cm3), pyridine (1cm3) and DMAP (0.002 g) was heated (1.5 h) under reflux and then strirred (12 h) at rt. The reaction mixture was concentrated under reduced pressure and the crude product obtained purified by PLC (50% EtOAc in hexane). (-)-Acetylisopteleflorine 23 was obtained as a white solid (0.010 g, 91%); mp 129-130 °C (from EtOAc-hexane); Rf 0.3; [α]D -6.4 (c 0.45, CHCl3) (Lit.,30 [α]D +6.4, CHCl3); δH (500 MHz, CDCl3) 1.55 (3H, s, CMe), 1.62 (3H, s, CMe), 1.97 (3H, s, OCOMe) 3.49 (1H, dd, J7a,7b 15.9, J7a,8 6.8, H-7a), 3.62 (1H, dd, J7b,7a 15.9, J7b,8 9.2, H-7b), 4.22 (3H, s, OMe), 4.97 (1H, dd, J8,7a 9.2, J8,7b 6.8, H-8), 6.14 (1H, d, J 1.5, OCH2O), 6.16 (1H, J 1.5, OCH2O), 6.95 (1H, d, J4,5 9.7, H-4), 7.58 (1H, d, J5,4 8.7, H-5); m/z 345 (M+, 45%); ECD : Δε -1.55 (251 nm), Δε +1.53 (222 nm), Δε +0.57 (197 nm).
(+)-(R)-O-Acetylisopteleflorine 23: [α]D +6.4 (c 0.40, CHCl3).
(+)-(S)-O-Methylhydroxylunium methiodide 24
A solution of (+)-(S)-isopteleflorine 22 (0.05 g, 0.164 mmol) in MeI (1 cm3) and EtOH (5 cm3) was heated (2 h) under reflux. The reaction mixture was concentrated under reduced pressure to give (+)-(S)-methiodide 24 (0.066 g, 90%) as a yellow solid; mp 155-156 °C (from EtOH) (Lit.,28 mp 159-161 °C); [α]D +16 (c 0.25, EtOH) (Lit.,22 [α]D -18, EtOH); δH (500 MHz, CD3OD) 1.30 (3H, s, CMe), 1.44 (3H, s, CMe), 3.95 (1H, dd, J7a,7b 15.5, J7a,8 6.8, H-7a), 3.97 (1H, dd, J7b,7a 15.5, J7b,8 9.2, H-7b), 4.22 (3H, s, OMe), 4.48 (3H, s, NMe), 5.23 (1H, dd, J8,7b 9.3, J8,7a 6.9, H-8), 7.28 (1H, d, J4,5 8.8, H-4), 7.91 (1H, d, J5,4 8.8, H-5); δC (125 MHz, CD3OD) 24.9, 26.0, 30.0, 60.6, 71.9, 95.7, 102.7, 104.7, 110.6, 116.9, 121.0, 124.6, 125.6, 153.7, 164.7, 168.6; m/z 303 (M+-MeI, 12 %), 141; Electronic CD: Δε -2.30 (261 nm), Δε +6.15 (221 nm).
(-)-(R)-O-Methylhydroxylunium iodide 24: [α]D -16 (c 0.19, EtOH).
(+)-(S)-Hydroxylunine 25
A solution of (+)-(S)-methiodide 24 (0.05 g, 0.11 mmol) in pyridine (1 cm3) was heated (3.5 h) under reflux in an oil bath. Pyridine was removed under reduced pressure and the residue purified by PLC (7% MeOH in CHCl3). (+)-(S)-Hydroxylunine 25 was obtained as a white solid (0.028 g, 85%); mp 224-225 °C (from EtOH) (Lit.,28 mp 222-225 °C); Rf 0.4 [α]D +25 (c 0.45, EtOH) (Lit.,26 [α]D -6); δH (500 MHz, CDCl3) 1.26 (3H, s, CMe), 1.38 (3H, s, CMe), 3.17 (1H, dd, J7a,7b 14.5, J7a,8 9.8, H-7a), 3.25 (1H, dd, J7b,7a 14.6, J7b,8 8.0, H-7b), 3.83 (3H, s, NMe), 4.80 (1H, dd, J8,7a 9.8, J8,7b 8.0, H-8), 6.03 (2H, s, OCH2O), 6.85 (1H, d, J4,5 8.5, H-4), 7.98 (1H, d, J5,4 8.5, H-5);δC (125 MHz, CDCl3) 24.6, 25.9, 27.6, 34.2, 71.4, 92.0, 101.4, 105.6, 120.9, 122.9, 125.2, 134.9, 149.6, 161.8, 173.0; m/z 303 (M+, 40 %), 232 (100); ECD data: Δε -1.67 (239 nm), Δε +2.63 (215 nm).
(-)-(R)-Hydroxylunine 25:[α]D -24 (c 0.40, EtOH).
ACKNOWLEDGEMENTS
We thank the BBSRC (N.D.S) and Oxford Glycosciences (P.L.L) for funding and also DENI (S.A.B) and Queen's University Belfast (P.L.L) for providing Postgraduate Studentships.
References
1. J. P. Michael, Nat. Prod. Rep., 2008, 25, 166, and earlier annual reviews in this journal by the same author. CrossRef
2. D. M. Jerina, J. W. Daly, and B. Witkop, J. Am. Chem. Soc., 1968, 90, 6525. CrossRef
3. D. R. Boyd, J. W. Daly, and D. M. Jerina, Biochemistry, 1972, 11, 1961. CrossRef
4. M. F. Grundon, D. M. Harrison, and C. G. Sypropoulos, J. Chem. Soc., Perkin Trans. 1, 1974, 2181. CrossRef
5. D. R. Boyd, N. D. Sharma, C. O’Dowd, J. G. Carroll, P. L. Loke, and C. C. R. Allen, Chem. Commun, 2005, 3989. CrossRef
6. S. Funayama, K. Murata, and S. Nozoe, Chem. Pharm. Bull., 1996, 44, 1885.
7. C. Ito, M. Itoigawa, A. Furukawa, T. Hirano, T. Murata, N. Kaneda, Y. Hisada, K. Okuda, and H. Furukawa, J. Nat. Prod., 2004, 67, 1800. CrossRef
8. C. L. Cantrell, K. K. Schrader, L. K. Mamonov, G. T. Sitpaeva, T. S. Kustova, C. Dunbar, and D. E. Wedge, J. Agric. Food Chem., 2005, 53, 7741. CrossRef
9. D. R. Boyd, N. D. Sharma, P. L. Loke, J. F. Malone, W. C. McRoberts, and J. T. G. Hamilton, Org. Biomol. Chem., 2007, 5, 2983. CrossRef
10. R. M. Bowman, J. F. Collins, and M. F. Grundon, J. Chem. Soc., Chem. Commun., 1967, 1131.
11. R. M. Bowman and M. F. Grundon, J. Chem. Soc.(C), 1967, 2368. CrossRef
12. R. M. Bowman, J. F. Collins, and M. F. Grundon, J. Chem. Soc., Perkin Trans.1, 1973, 626. CrossRef
13. M. F. Grundon, in ‘The Alkaloids: Quinoline Alkaloids Related to Anthranilic Acid’, Academic Press, London, 1988, 32, 341.
14. S. A. Barr and D. R. Boyd, J. Chem. Soc., Chem. Commun., 1994, 153. CrossRef
15. D. R. Boyd, N. D. Sharma, S. A. Barr, J. G. Carroll, D. Mackerracher, and J. F. Malone, J. Chem. Soc., Perkin Trans. 1, 2000, 3397. CrossRef
16. S. A. Barr, D. R. Boyd, N. D. Sharma, T. A. Evans, J. F. Malone, and V. D. Mehta, Tetrahedron, 1994, 50, 11219. CrossRef
17. E. A. Clarke and M. F. Grundon, J. Chem Soc., 1964, 4196. CrossRef
18. E. N. Jacobsen, W. Zang, A. R. Murci, J. R. Ecker, and L. Deng, J. Am. Chem. Soc., 1991, 113, 7063. CrossRef
19. N. H. Lee, A. R. Murci, and E. N. Jacobsen, Terahedron. Lett., 1991, 32, 5055. CrossRef
20. K. J. James and M. F. Grundon, J. Chem. Soc., Perkin Trans. 1, 1979, 1467. CrossRef
21. D. R. Boyd, N. D. Sharma, P. L. Loke, J. F. Malone, W. C. McRoberts, and J. T. G. Hamilton, Chem. Commun., 2002, 3070. CrossRef
22. M. Rideau, C. Verchere, P. Hibon, J. Chenieux, P. Maupas, and C. Viel, Phytochemistry, 1979, 18, 155. CrossRef
23. W. Zang, J. L. Leobach, S. R. Wilson, and E. N. Jacobsen, J. Am. Chem. Soc., 1991, 113, 7063. CrossRef
24. R. M. Bowman and M. F. Grundon, J. Chem. Soc. (C), 1966, 1504. CrossRef
25. S. Goodwin, A. F. Smith, A. A. Smith, A. A. Velasquez, and A. C. Horning, J. Am. Chem. Soc., 1959, 81, 6209. CrossRef
26. M. Terasaka, Chem. Pharm. Bull., 1960, 8, 523.
27. M. Terasaka, Chem. Pharm. Bull., 1959, 7, 946.
28. C. F. Neville, M. F. Grundon, V. N. Ramachandran, and J. Reisch, J. Chem. Soc., Perkin Trans. 1, 1991, 259. CrossRef
29. T. Noshita, M. Tando, K. Suzuki, K. Murata, and S. Funayama, Biosci. Biotechnol. Biochem., 2001, 65, 710. CrossRef
30. S. Funayama, K. Murata, and S. Nozoe, Phytochemistry, 1996, 41, 1231. CrossRef