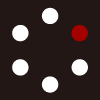
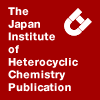
HETEROCYCLES
An International Journal for Reviews and Communications in Heterocyclic ChemistryWeb Edition ISSN: 1881-0942
Published online by The Japan Institute of Heterocyclic Chemistry
e-Journal
Full Text HTML
Received, 8th February, 2008, Accepted, 12th March, 2008, Published online, 14th March, 2008.
DOI: 10.3987/COM-08-S(F)4
■ Diels-Alder Reaction of 2-Pyridones Having an Acyl or a Sulfonyl Group on Nitrogen
Masato Hoshino, Kazuhiro Watanabe, Yosuke Ohtake, Takeshi Sato, Hisao Matsuzaki, and Reiko Fujita*
Tohoku Pharmaceutical University, 4-4-1 Komatsushima, Aoba-ku, Sendai 981-8558, Japan
Abstract
Diels-Alder (DA) reaction of 5-methoxycarbonyl-2-pyridone, which has an electron-withdrawing acyl group at nitrogen, with 1,3-diene afforded 2-quinolone derivatives in modest yields. Further, DA reaction of 5,4-dimethoxycarbonyl-1-sulfonyl-2-pyridone gave 2-quinolone and 1-isoquinolone (1:1). DA reaction of 2-sulfonyl-1-isoquinolone afforded a functionalized phenanthridone. The site-selectivity was well correlated with the corresponding activation energies calculated using an ab initio molecular orbital method.INTRODUCTION
There have been many reports describing the Diels-Alder (DA) reactions of 2(1H)-pyridones, which are aromatic, as dienes.1 In contrast, we have previously reported on the DA reaction of N-methyl-2(1H )-pyridones (A), which have an electron-withdrawing group at the 4- or 6-position, in which A acts as a dienophile, giving tetrahydro-1(2H)-isoquinolones (Scheme 1).2 To the best of our knowledge, there are few examples of the synthesis of tetrahydro-2(1H)-quinolones from the DA reaction of 2(1H)-pyridones. We have also reported on the DA reaction of 1-methyl-5-nitro-2(1H)-pyridones (B) to yield the aromatic 2(1H)-quinolone in modest yields (Scheme 1).3 From these results, we expect that 2(1H)-pyridones having two electron-withdrawing groups, at the 1- and 5-positions, would have higher reactivities as dienophiles than 2(1H)-pyridones having a withdrawing group at the 5-position only. It is considered that the additional electron-withdrawing group at the 1-position may decrease delocalization of the unshared electrons of the nitrogen atom, thus enhancing the dienophilic character of the pyridone ring. Recently, we report a novel method of preparing
tetrahydro-2(1H)-quinolones by DA reaction between 1-arylsulfonyl-2(1H)-pyridones (C), which have an electron-withdrawing group at the 5-position, and a diene.4 Moreover, we described a novel DA reaction in which 2-methyl-4-nitro-1(2H)-isoquinolone (D) reacts with a diene to give aromatic 5(6H)-phenanthridone derivatives in modest yields (Scheme 1).5 Herein, we wish to report DA reactions of the 1-acyl or 1-sulfonyl-2(1H)-pyridones, bearing a methoxy-carbonyl group at the 5-position, and 2-sulfonyl-4-methoxycarbonyl-1(2H)-isoquinolone. Further, site-selectivity analyses based on MO calculations of the 5-substituted 2(1H)-pyridones are investigated.
RESULTS AND DISCUSSION
1. DA reactions of 1-acyl- or 1-sulfonyl-2(1H)-pyridone derivatives with 1,3-butadiene
Firstly, the acylation of 2(1H)-pyridone 1,6 and sulfonylations of 4 and isoquinolone 9 were investigated (Scheme 2). Acylation of 5-methoxycarbonyl-2(1H)-pyridone 16 with p-nitrobenzoyl chloride 2 (1.5 equiv.) using NaH (1.5 equiv.) as a base in THF was carried out at room temperature for 2.5 h, providing the desired 1-p-nitrobenzoyl-2(1H)-pyridone 3 in 67% yield. Acylation of 1 using Et3N (1.5 equiv.) as a base afforded 3 in 63% yield. Under similar condition (NaH, room temperature for 6 h), the reaction of 4,5-dimethoxycarbonyl-2(1H)-pyridone 47 with p-tosyl chloride 5 gave 1-p-tosyl-2(1H)-pyridone 6 in 84% yield. Also, 1(2H)-Isoquinolone 9 was easily prepared from isoquinoline 7 via isoquinoline-1-oxide 8 in two steps (Scheme 2). Sulfonylation of 1(2H)-isoquinolone 9 with 5 afforded 2-p-tosyl-1(2H)-isoquinolone 10 in 63% yield under similar conditions.
Next, as listed in Table 1 and Scheme 2, the subsequent DA reactions of 1-acyl- or 1-sulfonyl-2(1H)-pyridone derivatives were examined. The DA reaction of benzoylpyridone 3 with 2,3-dimethyl-1,3-butadiene 11 at 180 or 160 °C for 3 d under atmospheric pressure (Entries 1, 2) proceeded stereoselectively and the site-selectively, afford the cis-adduct quinolone 12 (45% and 33%), which has a benzoyl group at the 1-position. The DA reaction of 6 with 11 at 120, 140, or 180 °C (Entries 3-5) gave stereoselectively the cis-adduct quinolone 13 (39%, 34% and 26%) and the cis-adduct isoquinolone 14 (37%, 33% and 27%); the total yields were 76%, 67% and 53%, respectively. Subsequent DA reaction of 10 with 11 under atmospheric or high pressure conditions yielded the cis-adduct phenanthridone 15 (18%, 11% and 17%; Entries 6-8) which has electron-withdrawing groups at the 2- and 10a-positions. The DA reaction of 2(1H)-pyridone 3, which has an acyl group at the 1-position afforded the cis-adduct quinolone the site-selectively in reasonable yield, whereas the DA reaction of 6 afforded the quinolone- and the isoquinolone-adducts in good total yield. However, the DA reaction of 10 gave the phenanthridone in low yield.
Assignment of the stereochemistry of the ring juncture in 12-15 were confirmed as follow. The cis-stereochemistry of the ring juncture in 12 was determined by NOE measurement. When H-8a (δ 5.05) was irradiated, an NOE was observed between H-8a and CH3OOC-4a (ca. 8%) in 12. This is consistent with a previous report, where we showed that the proton signals of the ring juncture in the cis-quinolone adducts appeared at δ 4.77-5.24.8 The cis-stereochemistry of the ring juncture in 13 was deduced by the similarity of the 1H-NMR spectra of 12 with that of 13 (H-8a, δ 5.16). The ring juncture in 15 was confirmed as cis from the signal at δ 5.28, which is assigned to the proton (H-6a) of the ring juncture in 15, which possess two cyclohexane rings (B, C: quinolone type). We have previously reported that signals arising from the proton at the ring juncture of cis-isoquinolone adducts (δ 2.84-3.20; Figure 1) are found at lower shifts than those of the corresponding trans-isoquinolone adducts (δ 2.00-2.80; Figure 1).8 The signal assigned to the proton (H-8a) of the ring juncture in 14 appeared at δ 3.12, supporting the existence cis stereochemistry.
2.Site-selectivity
The DA reactions of 3 and 6 as dienophiles with 11 were theoretically studied using Gaussian 03 at B3LYP/6-31G(d) level.9 Calculated activation energies (Ea) are summarized in Table 2 together with the experimental yields of the adducts. For the reaction of 3 with 11, the calculated Ea value is much smaller for (5, 6) than for (3, 4) addition, supporting the formation of adduct 12 as shown experimentally. For the reaction of 6 with 11, the difference in these Ea values is small, which is consistent with the fact that the experimental yields of 13 and 14 were almost the same.
CONCLUSION
In conclusion, we have developed a synthetic methodology of preparing tetrahydro-2(1H)-quinolones, isoquinolone and phenanthridone through DA reaction of 2(1H)-pyridone derivatives, having an electron-withdrawing group at the nitrogen, in which the 2(1H)-pyridone acts as dienophiles. Furthermore, when studying the DA reactions of 3 and 6 working as a dienophile, the calculated Ea values were useful to understand the site-selectivity in these reactions.
EXPERIMENTAL
The following instruments were used to obtain physical data: Melting points, Yanaco micromelting point apparatus (values are uncorrected); IR spectra, Perkin Elmer FT-IR1725X spectrophotometer; MS spectra, JEOL JMN-DX 303/JMA-DA 5000 spectrometer; NMR spectra, JEOL JNM-GSX 400 (1H-NMR, 400 MHz; 13C-NMR, 100 MHz), JEOL JNM-EX270 spectrometers (1H-NMR, 270 MHz; 13C-NMR, 67.8 MHz), with tetramethylsilane (TMS) as an internal standard. For column chromatography, Merck Kieselgel silica gel 60 (230-400 mesh) was used.
Synthesis of 5-methoxycarbonyl-1-(4-nitrobenzoyl)-2(1H)-pyridone (3). A solution of benzoyl chloride 2 (0.557 g, 3 mmol) in THF (10 mL) at -78 °C was added to a stirred suspension of 110 (0.459 g, 3 mmol) and NaH (0.072 g, 3 mmol) in THF (20 mL) at -25 °C. After 1 h, the reaction mixture was allowed to warm to rt, and then was stirred for 2.5 h. The mixture was poured into ice water (10 mL), neutralized with 10% NaOH aqueous solution, then extracted with CHCl3 (200 mL). The CHCl3 extract was dried over MgSO4 and concentrated in vacuo. The residue was purified by flash column chromatography (acetone-hexane = 1:2) to give benzoylpyridone 3 (0.610 g, 67%). Colerless plates (benzene), mp 138-140 °C. IR (KBr) cm-1: 1752, 1746, 1725, 1608, 1526, 859. 1H-NMR (CDCl3) δ: 3.99 (3H, s, OCH3), 6.45 (1H, d, J = 0.6, 9.6 Hz, 3-H), 8.37 (2H, dd, J = 2.7, 6.6 Hz, 3',5'-H), 8.42 (2H, dd, J = 2.7, 8.9 Hz, 2',6'-H), 8.49 (1H, dd, J = 2.3, 8.4 Hz, 4-H) , 9.09 (1H, dd, J = 0.6, 2.3 Hz, 6-H). 13C-NMR (CDCl3) δ: 52.5, 116.04, 123.9 (C2), 125.3, 131.6 (C2), 134.1, 141.2, 150.7, 151.2, 160.4, 162.5, 164.8. LMS m/z: 302 (M+), 274, 150, 104. HRMS m/z: Calcd for C14H10N2O6, 302.0538. Found: 302.05461.
General procedures for sulfonylations of 2(1H)-pyridone derivatives 4, 9 with TsCl (5). A solution of sulfonyl chloride 5 (1.14 g, 6 mmol) in THF (10 mL) at -78 °C was added to a stirred suspension of 4 (0.844 g, 4 mmol) and NaH (0.144 g, 6 mmol) in THF (20 mL) at -78 °C. After 1 h, the reaction mixture was allowed to warm to rt, and then was stirred for 5 h. The mixture was poured into ice water (10 mL), neutralized with 10% NaOH aqueous solution, then extracted with CHCl3 (200 mL). The CHCl3 extract was dried over MgSO4 and concentrated in vacuo. The residue was purified by flash column chromatography (acetone-hexane = 1:3) to give sulfonylpyridone 6 (1.23 g, 84%). Reaction of 9 (0.305 g, 1.5 mmol) with 5 (0.475 g, 2.5 mmol) were carried out under similar condition to give 10 (0.22g, 63%).
6: Colerless plates (acetone), mp 205-206 °C. IR (KBr) cm-1: 1740, 1724, 1687, 1616, 1532, 1311, 772, 731. 1H-NMR (CDCl3) δ: 2.46 (3H, s, Ph-Me), 3.88 (3H, s, OMe), 3.89 (3H, s, OMe), 6.44 (1H, d, J = 0.5 Hz, 3-H), 7.37 (2H, d, J = 8.6 Hz, 3',5'-H), 8.00 (2H, d, J = 8.6 Hz, 2',6'-H), 8.87 (1H, d, J = 0.5 Hz, 6-H). 13C-NMR (CDCl3) δ: 21.9, 52.7, 53.1, 108.3, 121.4, 129.6, 130.1, 131.9, 137.7, 144.7, 147.0, 158.4, 162.8, 165.4. LMS m/z: 365 (M+), 301 (M+-SO2), 242, 91. HRMS m /z: Calcd for C16H15NO7S, 365.0569. Found: 365.0601.
10: Colerless needles (acetone), mp 179-181 °C. IR (KBr) cm-1: 1718, 1697, 1592, 845, 782. 1H-NMR (CDCl3) δ: 2.44 3.97 (3H, s, PhMe), 7.38 ( 2H, d, J = 8.6 Hz, H-benzene), 7.49 (1H, ddd, J = 1.0, 7.3, 8.3 Hz, H-6), 7.75 (1H, ddd, J = 1.5, 7.3, 8.4 Hz, H-7), 8.05 (2H, d, J = 8.6 Hz, H-benzene), 8.30 (1H, dd, J = 1.5, 8.3 Hz, H-5), 8.75 ( 1H, dd, J = 1.5, 8.3 Hz, H-8), 8.89 (1H, s, H-3). 13C-NMR (CDCl3) δ:21.8, 52.2, 108.6, 125.8, 126.0, 128.1, 128.5, 129.7 (C2), 129.8 (C2), 133.1, 133.5, 133.8, 134.5, 146.5, 159.9, 165.0. MS m/z: 357 (M+), 293, 91. HR-MS m/z: Calcd for C18H15NO5S, 357.0671. Found: 357.0698.
Synthesis of 4-methoxycarbonylisoquinoline-1-oxide (8). A suspension of 7 (3.74 g, 20 mmol ) and m-chloroperoxybenzoic acid (4.14 g, 24 mmol) in CH2Cl2 (30 mL) was stirred at room temperature for 1 d, and m-chloroperoxybenzoic acid (4.14 g, 24 mmol) was added to the reaction mixture. After 2 d, the reaction mixture was diluted with CHCl3 (200 mL), and treated with K2CO3 (46 g, 0.33 mol) and H2O (15 mL). The organic layer was separated, dried over Na2SO4, and evaporated in vacuo to give 8 (2.52 g, 73%) as colorless plates (acetone), mp 102-105 °C. 1H-NMR (CDCl3) δ: 4.05 (3H, s, OMe), 7.58-7.78 (3H, m, aromatic –H), 7.83 (1H, dd, J = 1.1, 7.0 Hz, aromatic–H), 8.03 (1H, s, 3-H), 8.79 (1H, s, 1-H). 13C-NMR (CDCl3) δ: 53.3, 124.6, 125.6, 127.4, 127.7, 129.3, 130.1, 130.8, 137.3, 139.0, 162.2. HRMS m/z: Calcd for C11H9NO3, 203.0582. Found: 203.0586.
Reaction of 8 with acetic anhydride. A solution of 8 (2.03 g, 10 mmol) and acetic anhydride (10 mL) was refluxed for 8 h. After concentrating the reaction mixture in vacuo, the residue was purified using column chromatography (EtOAc). The first fraction was evaporated to give 4-methoxy-carbonyl-1(2H)-isoquinolone (9, 3.45 g, 85%) as colorless needles (acetone), mp 154-157 °C. 1H-NMR (CDCl3) δ: 4.00 (3H, s, OMe), 7.38 (1H, s, 3-H), 7.60-7.77 (3H, m, aromatic–H), 8.44 (1H, d, J = 8 Hz, 8-H), 9.22 (1H, s, OH). 13C-NMR (CDCl3) δ: 53.16, 111.30, 127.68, 127.92, 128.14, 128.31, 129.39, 133.03, 135.94, 161.69, 162.20. HRMS m/z: Calcd for C11H9NO3, 203.0582. Found: 203.0546.
General procedure for Diels-Alder reaction of 3, 6 and 10 with 11. A solution of 6 (0.365 g, 1 mmol) and 11 (0.410 g, 5 mmol) in o-xylene (3 mL) was heated at 120 °C for 3 d in a sealed tube. The reaction mixture was concentrated in vacuo, and then purified by column chromatography (acetone-hexane = 1:2). The first fraction was evaporated to give 13 (0.175 g, 39%). The second fraction was evaporated, then further purified by preparative TLC over silica gel (Et2O-hexane = 3:1) to afford 14 (0.166 g, 37%). Reactions of 3, 6, l0 (1 mmol) with 11 (5 mmol) were carried out under similar the conditions, as listed in Table 1, to afford 12-l5, respectively. Yields of 12-l5 are summarized in Table 1.
12:Pale yellow plates (Et2O), mp 153-155 °C. IR (KBr) cm-1: 1733, 1686, 1606, 1525, 822. 1H-NMR (CDCl3) δ:1.63 (3H, s, 6-CH3), 1.69 (3H, s, 7-CH3), 2.08 (1H, m, 8-H), 2.43 (1H, d, J = 17.3 Hz, 5-H), 2.66 (1H, d, J = 17.3 Hz, 5-H), 2.77 (1H, dd, J = 7.1, 17.3 Hz, 8-H), 3.78 (3H, s, OCH3), 5.10 (1H, dd, J = 2.3, 7.1 Hz, 8a-H), 5.97 (1H, d, J = 9.6 Hz, 3-H), 6.68 (1H, dd, J = 2.3, 9.6 Hz, 4-H), 7.57 (2H, d, J = 8.9 Hz, 2',6'-H), 8.23 (2H, d, J = 8.9 Hz, 3',5'-H). 13C-NMR (CDCl3) δ: 18.6, 18.7, 35.0, 39.9, 47.1, 53.0, 54.5, 122.3, 124.5, 125.7, 126.0, 128.5, 142.0, 148.1, 149.0, 163.5, 171.2, 172.8. LMS m/z: 384 (M+), 186, 159, 150. HRMS m/z: Calcd for C20H20N2O6, 384.1321. Found: 384.1299.
13: Coloreless oil. IR (film) cm-1: 1732, 1693, 1597, 1359, 815. 1H-NMR (CDCl3) δ: 1.56 (3H, s, C-CH3), 1.60 (3H, s, C-CH3), 2.02-2.12 (1H, brm, 8-H), 2.42 (3H, s, C6H5-CH3), 2.67-2.76 (2H, brm, 5,8-H), 3.18 (1H, d, J = 17.2 Hz, 5-H), 3.56 (3H, s, OCH3), 3.77 (3H, s, OCH3), 5.16 (1H, dd, J = 7.3, 9.8 Hz, 8a-H), 6.62 (1H, s, 3-H), 7.29-7.37 (2H, m, 3', 5'-H), 7.90-7.94 (2H, m, 2', 6'-H). 13C-NMR (CDCl3) δ: 18.4, 18.6, 21.7, 36.0, 38.0, 50.2, 52.6, 53.2, 57.9, 123.8, 125.6, 129.0, 129.1, 132.0, 135.7, 142.6, 145.0, 161.0, 164.5, 172.1. LMS m/z: 447 (M+), 292 (M+-Ts), 242. HRMS m/z: Calcd for C22H25NO7S, 447.1352. Found: 447.1320.
14: Coloreless oil. IR (film) cm-1: 1716, 1640, 1597, 1379, 815. 1H-NMR (CDCl3) δ: 1.52 (3H, s, C-CH3), 1.56 (3H, s, C-CH3), 1.80 (1H, d, J =18.4 Hz, 5-H), 1.86 (1H, d, J =16.8 Hz, 8-H), 2.45 (3H, s, C6H5-CH3), 2.47 (1H, d, J = 16.8 Hz, 8-H), 2.63 (1H, d, J = 18.4 Hz, 5-H), 3.12 (1H, m, J = 1.0, 2.1, 4.2 Hz, 8a-H), 3.67 (3H, s, OCH3), 3.78 (3H, s, OCH3), 7.30-7.36 (2H, m, 3', 5'-H), 7.86-7.94 (2H, m, 2', 6'-H), 8.13 (1H, s, 3-H). 13C-NMR (CDCl3) δ: 18.6, 18.7, 21.7, 27.4, 35.1, 44.3, 46.4, 52.0, 52.7, 116.4, 121.5, 122.3, 128.6, 129.5, 132.8, 134.0, 145.7, 164.6, 167.7, 172.4. LMS m/z: 447 (M+), 388, 242. HRMS m/z: Calcd for C22H25NO7S, 447.1352. Found: 447.1388.
15: Coloreless crystalline powder (acetone), mp 240-243 °C. IR (KBr) cm-1: 1720, 1697, 1660, 1624, 786. 1H-NMR (CDCl3) δ: 1.38 (3H, s, C-Me), 1.63 (3H, s, C-Me), 1.80-1.90 (1H, brm, CH), 2.34 (3H, s, Ph-Me), 7.75 (1H, ddd, J = 1.5, 7.3, 8.4 Hz, H-7), 8.05 (2H, d, J = 8.6 Hz, H-benzene), 8.30 (1H, dd, J = 1.5, 8.3 Hz, H-5), 2.53 (1H, dd, J = 6.6, 10.9 Hz, CH), 2.82 (2H, s, CH2), 3.40 (3H, s, OMe), 5.28 (1H, dd, J = 6.6, 10.9 Hz, 6a-H), 7.19-7.33 (4H, m, aromatic-H), 7.47 (1H, ddd, J = 1.5, 7.8, 7.8 Hz, aromatic -H), 7.91-7.99 (3H, brm, aromatic-H). 13C-NMR (CDCl3) δ: 18.47, 18.7 (C2), 21.8, 37.2, 50.1 (C2), 53.0, 56.6, 121.7, 125.0, 125.6, 128.0, 128.9, 129.0 (C2), 129.0, 129.7, 133.6, 136.3, 137.0, 144.6, 162.2, 173.3. LMS m/z: 439 (M+), 293, 91. HRMS m/z: Calcd for C24H25NO5S, 439.1454. Found: 439.1476.
Diels-Alder reaction of 10 with 11 under High pressure. A dichloromethane solution (1 mL) of 10 (0.089 g, 0.25 mmol) and 11 (103 g, 1.25 mmol) was placed in Teflon tube. The tube was placed in a high pressure reactor and pressurized to 10 kbar, followed by heating at 90 °C. After 2 d, the pressure was released and the reaction mixture was chlomatographed over silica gel using hexane-EtOAc (2:1) as eluent to afford 15 (0.015 g, 17%).
Calculation of activation energies. The structures of each state were optimized using the ab initio molecular orbital method Gaussian 03 at B3LYP/6-31G(d) level.9 The reactants were assumed to be far apart at the initial state. Two types of the transition state (TS) were searched; the one making bonds at the 3 and 4 positions of 3 or 6 and the other at the 5 and 6 positions. Solvent effects were not considered. After optimizing TS structures, vibrational calculations were carried out to confirm that the TS had only one imaginary vibrational frequency. Intrinsic reaction coordinate calculations were also performed to ensure that the TS connected the initial and the desired final states. The Ea values were defined as the difference in the energies between the TS and initial states.
This paper is dedicated to Professor Emeritus Keiichiro Fukumoto on the occasion of his 75th birthday
References
1. Review for Diels-Alder reaction of 2(1H)-pyridones: K. Afarinkia, V. Vinader, T. D. Nelson, and G. H. Posner, Terahedron, 1992, 48, 9111; CrossRef H. Tomisawa, R. F ujita, K. Noguchi, and H. Hongo, Chem. Pharm. Bull., 1970, 18, 941; H. Nakano, Y. Saito, and H. Hongo, Chem. Pharm. Bull., 1992, 40, 2876.
2. R. Fujita, M. Hoshino, H. Tomisawa, and H. Hongo, Chem. Pharm. Bull., 2000, 48, 1814; R. Fujita, M. Hoshino, H. Tomisawa, and H. Hongo, Chem. Pharm. Bull., 2001, 49, 497; CrossRef H. Kato, R. Fujita, H. Hongo, and H. Tomisawa, Heterocycles, 1979, 12, 1. CrossRef
3. R. Fujita, K. Watanabe, Y. Nishiuchi, R. Honda, H. Matuzaki, and H. Hongo,Chem. Pharm. Bull., 2001, 49, 601. CrossRef
4. R. Fujita, K. Watanabe, W. Ikeura, Y. Ohtake, H. Hongo, Y. Haragaya, and H. Matuzaki, Tetrahedron, 2001, 57, 8841; CrossRef R. Fujita, K. Watanabe, W. Ikeura, Y. Ohtake, H. Hongo, and H. Tomisawa, Heterocycles, 2000, 53, 2607. CrossRef
5. R. Fujita, S. Wakayanagi, H. Wakamatsu, and H. Matsuzaki, Chem. Pharm. Bull., 2006, 54, 209. CrossRef
6. H. Nakano, Y. Saito, and H. Hongo, Chem. Pharm. Bull., 1992, 40, 2876.
7. A. Nuvole and G. Paglietti, J. Chem. Soc., Perkin Trans. 1, 1989, 1007. CrossRef
8. R. Fujita, K. Watanabe, T. Yoshisuji, H. Matsuzaki, Y. Haragaya, and H. Hongo, Chem. Pharm. Bull., 2001, 49, 407. CrossRef
9. Gaussian 03, Revision C.02, M. J. Frisch, G. W. Trucks, H. B. Schlegel, G. E. Scuseria, M. A. Robb, J. R. Cheeseman, J. A. Montgomery, Jr., T. Vreven, K. N. Kudin, J. C. Burant, J. M. Millam, S. S. Iyengar, J. Tomasi, V. Barone, B. Mennucci, M. Cossi, G. Scalmani, N. Rega, G. A. Petersson, H. Nakatsuji, M. Hada, M. Ehara, K. Toyota, R. Fukuda, J. Hasegawa, M. Ishida, T. Nakajima, Y. Honda, O. Kitao, H. Nakai, M. Klene, X. Li, J. E. Knox, H. P. Hratchian, J. B. Cross, V. Bakken, C. Adamo, J. Jaramillo, R. Gomperts, R. E. Stratmann, O. Yazyev, A. J. Austin, R. Cammi, C. Pomelli, J. W. Ochterski, P. Y. Ayala, K. Morokuma, G. A. Voth, P. Salvador, J. J. Dannenberg, V. G. Zakrzewski, S. Dapprich, A. D. Daniels, M. C. Strain, O. Farkas, D. K. Malick, A. D. Rabuck, K. Raghavachari, J. B. Foresman, J. V. Ortiz, Q. Cui, A. G. Baboul, S. Clifford, J. Cioslowski, B. B. Stefanov, G. Liu, A. Liashenko, P. Piskorz, I. Komaromi, R. L. Martin, D. J. Fox, T. Keith, M. A. Al-Laham, C. Y. Peng, A. Nanayakkara, M. Challacombe, P. M. W. Gill, B. Johnson, W. Chen, M. W. Wong, C. Gonzalez, and J. A. Pople, Gaussian, Inc., Wallingford CT, 2004