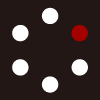
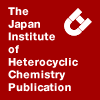
HETEROCYCLES
An International Journal for Reviews and Communications in Heterocyclic ChemistryWeb Edition ISSN: 1881-0942
Published online by The Japan Institute of Heterocyclic Chemistry
e-Journal
Full Text HTML
Received, 1st October, 2008, Accepted, 27th November, 2008, Published online, 2nd December, 2008.
DOI: 10.3987/COM-08-S(D)55
■ Stereoselective Synthesis of 2,3,5-Trisubstituted Pyrrolidines Using Metathesis-Derived β-Aminoallylsilanes
Alison D. McElhinney and Stephen P. Marsden*
School of Chemistry, The University of Leeds, Leeds, LS2 9JT, U.K.
Abstract
Amine-functionalised allylsilanes, readily prepared by olefin cross metathesis of protected homoallylamines with allyltrimethylsilane, undergo stereoselective condensation with aldehydes to yield trisubstituted pyrrolidines.Polysubstituted pyrrolidines are prevalent in biologically active compounds, encompassing both natural products, such as the neuorexcitatory kainic acid, and synthetic pharmaceutical candidates such as the anti-influenza compound A-315675. Additionally, they occur widely as substructures of fused or bridged polycyclic motifs, as in the indolizidine alkaloid tashiromine or the anti-HIV drug MaravirocTM (Figure 1). Methods for their efficient and stereoselective preparation are therefore in constant demand.2
The intramolecular trapping of iminium ions by pendant allylsilanes as a route to pyrrolidines, piperidines and their polycyclic variants has been an area of interest since the first reports of Hiemstra and Speckamp,3 and still finds widespread application in target synthesis.4 In all of these studies, the assembly of an amine-functionalised allylsilane is a prerequisite. We5 and others6 have previously demonstrated the efficient synthesis of oxygen-functionalised allylsilanes by ring-closing olefin metathesis, and their subsequent exploitation for the stereoselective formation of oxygen heterocycles and carbocycles. We therefore conceived a similar plan for the convergent synthesis of polysubstituted pyrrolidines based on the retrosynthesis below (Figure 2), wherein the key amine-bearing allylsilane would be prepared by olefin cross-metathesis with commercial allyltrimethylsilane. This latter strategy has not previously been reported as a route to simple pyrrolidines, though it has found some application in total syntheses of polycyclic products,7 including our own synthesis of tashiromine.8 We report herein the successful results of our studies.
Initial attempts at cross-metathesis between allyltrimethylsilane and N-benzyl allylglycinate 1a met with failure, presumably due to the basic nature of the nitrogen. However, the use of electron-withdrawing protecting groups such as Fmoc or Bz allowed for efficient cross-metathesis of homoallylamines 1b-e, providing the corresponding allylsilanes 2b-e with moderate E-selectivity. Since early experiments revealed that a strongly nucleophilic nitrogen was required for productive iminium formation, conversion to the corresponding secondary benzylamines 3b-e was achieved by deprotection/reductive amination (from 2b) or simple hydride reduction (from benzoyl amides 2c-e).
Pleasingly, after some optimisation, we found that condensation of allylsilanes 3b-e with a range of aldehydes could be effected in good yield under the action of trimethylsilyl triflate in the presence of diisopropylethylamine (to minimise unwanted protodesilylation by adventitious triflic acid). The results of our screen are shown in Table 1. The reactions work well for a range of aromatic and heteroaromatic-substituted aldehydes, with yields generally in the range 65-85%. The reactions produced mixtures of the four possible stereoisomeric products, with diastereomer a generally forming 60-90% of the mixture.
The internal alkene signals in the 1H NMR spectra appeared at consistent and distinct chemical shifts for each diastereoisomer across the various pyrrolidines,10 allowing assignment of diastereomeric ratios as shown. The relative stereochemistry of the major stereoisomer a was determined by X-ray crystallography for 7a,11 and by nOe studies for 4a. The stereochemistries of the three minor components could not be unambiguously assigned at this stage. A model explaining the preferential formation of the 2,3-trans,2,5-trans isomers a is given in Figure 3. This major product arises from a chair-like transition state in which the amine α-substituent is in an equatorial position and the iminium ion has Z-configuration, establishing the 2,5-trans relationship. The pseudo-equatorial disposition of the allylsilane then leads to the 2,3-trans configuration upon cyclisation. The minor isomers may arise from various combinations of (a) E-configured iminium ions; (b) boat-like transition states; or (c) axial disposition of the amine α-substituent.
At the heart of the selectivity issue lies the problem of control of iminium geometry. We reasoned that this ought to be influenced by the bulk of the nitrogen substituent, an effect elegantly exploited by Overman in constructing an octahydroisoquinoline by allylsilane/iminium condensation in his synthesis of morphine.12 We therefore investigated the effect of the protecting group bulk by preparing the N-methyl and N-isopropyl-protected aminoallylsilanes 3f and 3g13 and investigated their condensation with 4-nitrobenzaldehyde (Scheme 2). As expected, with the smaller N-methyl substituent the diastereoselectivity fell, presumably reflecting a higher contribution from the E-iminium ion compared to the N-benzyl substituent. Conversely, moving to the bulkier isopropyl substituent heavily disfavoured the E-iminium, leading to almost exclusive formation of 18a by way of the Z-iminium ion.
In summary, we have shown that olefin cross-metathesis allows ready access to a range of substituted amine-functionalised allylsilanes, and that these undergo stereoselective cyclisation to generate pyrrolidines with the 2,3-trans,2,5-trans stereoisomer being favoured. Control of the levels of stereoselectivity by manipulation of the N-substituent is possible. The further development of this chemistry to encompass the synthesis of piperidines is under study and will be reported in due course.
ACKNOWLEDGEMENTS
We thank the EPSRC for a DTA studentship (to ADM) and Pfizer for unrestricted research funding.
References
1. This paper is in memory of the late Professor John W. Daly.
2. D. O’Hagan, Nat. Prod. Rep., 2000, 17, 435. CrossRef
3. H. Hiemstra, W. J. Klaver, and W. N. Speckamp, J. Org. Chem., 1984, 49, 1149; CrossRef H. Hiemstra, M. H. A. M. Sno, R. J. Vijn, and W. N. Speckamp, J. Org. Chem., 1985, 50, 4014; CrossRef H. Hiemstra, H. P. Fortgens, and W. N. Speckamp, Tetrahedron Lett., 1985, 26, 3155. CrossRef
4. For some recent examples, see: W. R. Judd, S. Ban, and J. Aube, J. Am. Chem. Soc., 2006, 128, 13736; CrossRef S. Kwon and A. G. Myers, J. Am. Chem. Soc., 2005, 127, 16796, and references 7 and 8. CrossRef
5. J. H. Cassidy, S. P. Marsden, and G. Stemp, Synlett, 1997, 1411; CrossRef S. M. Miles, S. P. Marsden, R. J. Leatherbarrow, and W. J. Coates, J. Org. Chem., 2004, 69, 6874; CrossRef S. M. Miles, S .P. Marsden, R. J. Leatherbarrow, and W. J. Coates, Chem. Commun., 2004, 2292; CrossRef T. Akindele, S. P. Marsden, and J. G. Cumming, Org. Lett., 2005, 7, 3685; CrossRef T. Akindele, S. P. Marsden, and J. G. Cumming, Tetrahedron Lett., 2005, 46, 7235; CrossRef J. H. Cassidy, C. N. Farthing, S. P. Marsden, A. Pedersen, M. Slater, and G. Stemp, Org. Biomol. Chem., 2006, 4, 4118. CrossRef
6. C. Meyer and J. Cossy, Tetrahedron Lett., 1997, 38, 7861; CrossRef S. Garcia Munoz, L. Jimenez Gonzalez, M. Alvarez Corral, M. Munoz Dorado, and I. Rodriguez Garcia, Synlett, 2005, 3011; CrossRef L. Jimenez Gonzalez, M. Alvarez Corral, M. Munoz Dorado, and I. Rodriguez Garcia, Chem. Commun., 2005, 2689; CrossRef L. Jimenez Gonzalez, S. Garcia Munoz, M. Alvarez Corral, M. Munoz Dorado, and I. Rodriguez Garcia, Chem. Eur. J., 2006, 12, 8762; CrossRef M. Alvarez Corral, C. Lopez Sanchez, L. Jimenez Gonzalez, A. Rosales, M. Munoz Dorado, and I. Rodriguez Garcia, Beilstein J. Org. Chem., 2007, 3; L. Jimenez Gonzalez, S. Garcia Munoz, M. Alvarez Corral, M. Munoz Dorado, and I. Rodriguez Garcia, Chem. Eur. J., 2007, 13, 557. CrossRef
7. Synthesis of lemonomycin: K. Rikimaru, K. Mori, T. Kan, and T. Fukuyama, Chem. Commun., 2005, 394; CrossRef synthesis of 2,6-bridged piperazine-3-ones: J. J. N. Veerman, R. S. Bon, B. T. B. Hue, D. Girones, F. P. J. T. Rutjes, J. H. van Maarseveen, and H. Hiemstra, J. Org. Chem., 2003, 68, 4486. CrossRef
8. A. D. McElhinney, and S. P. Marsden, Synlett, 2005, 2528. CrossRef
9. Sample experimental procedure for the condensation of allylsilanes 3 with aldehydes: Allylsilane 3b (296 mg, 0.97 mmol) and benzaldehyde (98 μL, 0.97 mmol) were dissolved in anhydrous toluene (4 mL) and heated to reflux for 2 h. Diisopropylethylamine (0.25 mL, 1.45 mmol) and trimethylsilyl trifluoromethanesulfonate (0.26 mL, 1.45 mmol) were added and the reaction heated under reflux for 19 h. The solution was allowed to cool to rt and the solvent removed in vacuo. The residue was redissolved in CH2Cl2 (20 mL), washed with water (15 mL), dried over MgSO4 and the solvent was removed in vacuo. Purification by column chromatography (2% EtOAc/petrol) yielded as a mixture of diastereomers isolated in two fractions: fraction 1 (157 mg, 50%) was a 85:0:0:15 mixture of 4a:b:c:d; fraction 2 (55 mg, 18%) was a 13:66:21:0 mixture of 4a:b:c:d. Combined yield was 212 mg (68%) of a 66:17:6:11 mixture of 4a:b:c:d. NMR data for diastereomer 4a: δH (300 MHz, CDCl3) 7.45-7.18 (10H, m), 5.84 (1H, ddd, J 16.9, 10.2, 8.2), 4.91 (1H, dd, J 10.2, 1.0), 4.78 (1H, dd, J 16.9, 1.0), 4.06 (1H, d, J 8.2), 3.83 (1H, dd, J 8.7, 3.4), 3.70 (1H, d, J 13.5), 3.66 (3H, s), 3.50 (1H, d, J 13.5), 2.67 (1H, app. quintet, J 8.2), 2.51 (1H, dt, 13.3, 8.7), 1.78 (1H, ddd, 13.3, 6.7, 3.4); δC (75 MHz, CDCl3) 175.5, 142.1, 139.7, 139.5, 128.9, 128.8, 128.6, 128.3, 127.8, 127.3, 115.8, 72.6, 61.1, 52.8, 52.0, 51.5, 34.7. NMR data for diastereomer 4b: δH (300 MHz, CDCl3) 7.46-7.02 (10H, m), 5.62 (1H, ddd, J 17.5, 10.1, 7.7), 4.90 (1H, d, J 10.2), 4.80 (1H, d, J 17.5), 3.85 (1H, d, J 14.0), 3.53 (1H, d, J 3.3), 3.49 (1H, m), 3.46 (3H, s), 3.42 (1H, m), 2.78 (1H, app. quintet, J 8.7), 2.04 (1H, ddd, 12.6, 7.7, 3.4), 1.78 (1H, dt, J 12.6, 8.2); δC (75 MHz, CDCl3) 176.7, 142.5, 138.9, 138.8, 130.8, 129.6, 129.5, 129.2, 128.7, 128.2, 117.4, 75.5, 64.6, 56.0, 52.9, 52.8, 36.3. Diagnostic (internal alkene) 1H NMR signals for diastereomers 4c and 4d appeared at 5.47 and 5.14 ppm respectively. HRMS Found [M+H]+ 322.1819. C21H24NO2 requires M+ 322.1807 .
10. For all pyrrolidines 4-18 (except the C2-alkyl 13) the characteristic chemical shifts always appeared in the order a>b>c>d, and characteristic values are as follows: a average δ 5.85 ppm, range 5.94–5.75 ppm; b average δ 5.64 ppm, range 5.81–5.54 ppm; c average δ 5.46 ppm, range 5.69–5.18 ppm; d average δ 5.14 ppm, range 5.36–4.92 ppm .
11. CCDC-709222 contains the supplementary crystallographic data for compound 7a. This data can be obtained free of charge from The Cambridge Crystallographic Data Centre via www.ccdc.cam. ac.uk/data_request/cif.
12. C. Y. Hong, N. Kado, and L. E. Overman, J. Am. Chem. Soc., 1993, 115, 11028. CrossRef
13. Prepared from 1-phenylbut-3-en-1-ylamine as follows: 3f by formylation, cross-metathesis with allyltrimethylsilane, and LiAlH4 reduction; 3g by Fmoc protection, cross-metathesis with allyltrimethylsilane, deprotection with piperidine and reductive amination with acetone/sodium cyanoborohydride.