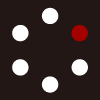
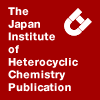
HETEROCYCLES
An International Journal for Reviews and Communications in Heterocyclic ChemistryWeb Edition ISSN: 1881-0942
Published online by The Japan Institute of Heterocyclic Chemistry
e-Journal
Full Text HTML
Received, 1st October, 2008, Accepted, 1st December, 2008, Published online, 10th December, 2008.
DOI: 10.3987/REV-08-SR(D)5
■ Epibatidine: From Frog Alkaloid to Analgesic Clinical Candidates. A Testimonial to “True Grit”!
H. Martin Garraffo, Thomas F. Spande, and Michael Williams*
Discovery Research, Cephalon Inc., 145 Brandywine Parkway, West Chester, PA 19380, U.S.A.
Abstract
A routine toxicity test of the alkaloid extract from the Ecuadoran poison frog Epipedobates anthonyi gave a Straub-tail (S-T) response on sub-cutaneous (sc) injection in mice, a phenomenon never seen before from any poison frog alkaloid. It is characteristic of opioids; however, in this instance it was not blocked by a morphine-antagonist, naloxone. Its site of action was soon shown to be a nicotinic receptor. The determination of the structure of this novel analgesic named epibatidine has led to a renaissance of research into controlling pain via nicotinic pathways (thereby minimizing the risk of tolerance/addiction) and the synthesis of many analogs, some of which are discussed.1. DISCOVERY AND STRUCTURE DETERMINATION
In 1974, while routinely screening frog skin extracts for toxicity from specimens of a frog then known as Phyllobates anthonyi, collected with Charles Myers in February, 1974 at Pasaje (44 frogs) and Sta. Isabel (10 frogs), Ecuador, John Daly recorded in his notebooks a phenomenon he had never seen before, an S-T response. He recorded a typical sc injection of the Sta. Isabel extract equivalent to 100 mg of wet skin: “agitation, labored breathing, pronounced S-T, running convulsions, 3 min on side twitching, still pronounced S-T, rolling convulsions, arching back, extends hind feet…cannot locomote, still rights itself readily.” This arching and rigidity of the mouse tail after sc injection John knew was characteristic of an opioid. Running an analgesia hot plate assay, John realized the extract had indeed powerful analgesic properties; later this analgesic was found to be 200 times more potent than morphine on a weight basis. He showed in 1978 that the S-T response was not blocked by naloxone and consequently the analgesia was not dependent on an opioid receptor. The response was seen from frogs of both sites (which were quite different in color and size) but only in some populations; no correlation with color or habitat was evident. The Sta. Isabel frogs were later called P. tricolor, then Dendrobates tricolor; the Pasaje frogs were called P. anthonyi, then later also D. tricolor. The S-T effect persisted undiminished after alkaloid partitioning. By weight of the alkaloid extract administered, the original Pasaje frogs had only 5% of the S-T activity of the Sta. Isabel collections. Extracts of the initial 54 frogs were quickly exhausted by the demands of the assay and the less sensitive analytical methods at that time (preparative and analytical TLC with iodine-vapor detection; packed column GC with FID; direct-probe MS). One preparative TLC zone associated with a S-T response clearly showed a chlorine-containing [M+H]+ ion (211/209) on CIMS for a P. anthonyi extract from Pasaje; most MS analyses though, of other S-T-zones gave ambiguous results. John returned with Myers to Ecuador several times to collect more frog samples. Their further collections were:
Oct. 1974, 1730 skins of P. anthonyi, Pasaje and 70 from Sta. Isabel.
Nov. 1976, 765 skins of P. anthonyi (D. tricolor), Sta. Isabel + 43 skins from 3 other sites (the most useful collection). No frogs found at all from the original Pasaje site!
Nov. 1979, 365 skins, D. tricolor, Sta. Isabel + 23 skins from 4 other sites.
By 1979, using the tools available to him, John had determined the analgesic substance was present as a trace material of m/z 208/210 on the tail side of a major GC peak (emergent temp. = 218 °C) for pumiliotoxin 251D, 1 and reproducibly confirmed one chlorine in the molecular ion by low resolution EIMS. One D-exchange was detected in CIMS with ND3. The 1976 collection provided a conservative estimate that 2.5 µg of the analgesic compound would cause a pronounced S-T effect. We estimate from integration of the total ion chromatogram of that extract that each frog in that collection had 2 µg of the 208/210 alkaloidal S-T substance. The S-T bioassay was gradually supplanted by a GC assay based on the 218 °C peak. All of the extracts were very toxic in the bioassay with mice, even lethal within minutes, at the amounts being injected.
John was understandably still skeptical however that a naturally occurring chlorine-containing alkaloid was responsible for the S-T effect since this would have been the first example of a chlorine-containing alkaloid detected among the 200 or so amphibian alkaloids known at that time, thus an impurity or an artifact of isolation was considered more likely. Repeating the alkaloid partitioning with chlorine-free acid and solvent eliminated the latter but the impurity possibility was entertained for years, mainly because of the capricious appearance of the S-T effect. Arguing against the early HRMS data (Peter Roller), which indicated a formula C11H13N2Cl for the 208/210 compound, was John’s instinct that its polarity relative to nicotine on TLC (Rf 0.25 vs. 0.70) required the presence of at least one hydroxyl group. Other HRMS data was incorrectly interpreted indicating one or more oxygens in the molecular formula. Using a GC-microwave plasma detector (MPD 850), a formula for m/z 210 of C10H11N2O35Cl resulted confirming chlorine and an anticipated oxygen but ultimately this proved misleading as did the results from a double-beam high-resolution MS-30 instrument that indicated even more oxygens in a C8H15NO335Cl formula for the m/z 208 ion. These side-tracked John for some months until Michael Edwards in 1978, using HPLC with material partially purified by silica column chromatography, provided nearly pure samples of the S-T substance and conclusive mass spectra using N2-NO for EIMS (with an enhanced molecular ion) and CIMS with methane or ammonia and these showed m/z 208/210 and 209/211, respectively. During the silica chromatography, only ~ 40% of the S-T “mouse units” were recovered in the five silica column 208/210 fractions. The HPLC fractions (amounting to 1.5 mg of nearly pure material) were then partly consumed in fruitless chemical reactions such as trimethylsilylation, hydrogenation and acetylation (acetylation yielded ambiguous results initially). Because of the observation that the 208/210 alkaloid was absent in many collections or populations of the same frog, John continued to suspect it was a pyrolysis product and the true analgesic was a parent 308/310 substance. On GC, the 208/210 alkaloid emerged between the MW 251 and 267 pumiliotoxins, suggesting a higher true molecular weight.
Not until 1984, was it clear to John and his several coworkers that the m/z 208/210 substance was not an impurity but was originally present (or a precursor of it) in frog skin, having an oxygen-free formula, and despite being much more polar than nicotine, had an exchangeable and acetylatable secondary amine NH and reproducibly provided the S-T effect. The concept that the m/z 208/210 compound was a pyrolysis product was still considered possible since some purified HPLC fractions gave an S-T response that did not reach a maximum for 30 min while others had an immediate effect. That behavior now appears to have been concentration-dependent. Some preparative thin layer and silica column chromatography did, however, indicate a second, lower Rf S-T component to be present, although it was less active. Some MS also indicated that formulae for m/z 208/210 were fragment ions as even numbers of H were mass-measured with one N only. At this point, Edwards undertook the synthesis of two structural possibilities for the m/z 208/210 chlorine-free nucleus of the putative “pyrolysis product”: 1) an analog of nicotine and 2) an amidine derivative. Neither product had any analgetic effect and further synthetic work was abandoned.
John, like Captain Ahab obsessed with Moby Dick, continued to invest time, manpower and instrument dollars into solving the structure of the elusive m/z 208/210 substance whose presence in P. anthonyi (D. tricolor) skins was erratic and whose amounts were frustratingly variable.
We bought our own MPD but found it troublesome. Then in the late 1980’s, John bought a bench top Finnigan Model 800 Ion Trap interfaced with a Varian GC having a programmable injector (which tended to rule out pyrolysis producing the m/z 208/210 compound) and an HP5965A vapor phase IR spectrophotometer. IR spectra conclusively ruled out any hydroxyl group and ruled in a likely chloropyridine moiety. At this time John adopted a moratorium on further bioassays, purification or chemical characterization work, allowing only some GC studies. Raising the stakes higher was an international CITES agreement that pretty much shut down any further collections of dendrobatid frogs, even though many were not endangered. John had used so much of the m/z 208/210 substance from the Ecuadorian frogs, either from the repeated bioassays, or in mass spectrometry, that less than 1 mg of material remained. Every cutting edge or routine MS instrument available had been tried but often ambiguous data resulted. The originally used JEOL instrument had in retrospect, provided some of the most reliable data. The m/z 208/210 was always a trace and in GC followed closely the often overloaded pumiliotoxin 251D, 1, that invariably gave perfect HRMS data with any instrument. This was rarely true of the m/z 208/210 alkaloid. One huge collection from a banana plantation near Pasaje, frustratingly gave no S-T effect at all and had in fact, scarcely any alkaloids. We now surmise that the 1730 frogs collected there had been feasting on alkaloid-free fruit flies and the optional arthropod prey was scarce or ignored. The dietary hypothesis was not formulated until near the end of the structural work on the S-T compound. Then the fruitless attempts to isolate it or any alkaloids from captive-raised D. tricolor or F1 offspring as well as the seemingly random occurrence of the 208/210 alkaloid and other alkaloids in wild caught frogs could be explained. The arthropod source of the 208/210 alkaloid is still elusive.
By 1991 our own 500 MHz NMR spectrometer became available. Fortunately, the partially purified extract containing minor amounts of the m/z 208/210 substance that remained, had been shown to be stable in long-term storage at -5 °C. Our lab then purified the remaining sample (see Conclusion) and a simple 1D 1H-NMR revealed the structure.1 Not until the structure 2 was known, was the name “epibatidine” applied; by that time the two frog species had been folded into one, Epipedobates tricolor, and now it has been renamed Epipedobates anthonyi. The name epibatidine came from Epipedobates and the fact that it was a diamine, tactfully removing the middle part of the name of the frog for different connotations in Spanish. Nothing is known about epibatidine biosynthesis, not even if the frog, Epipedobates anthonyi, makes epibatidine or sequesters it from a yet unknown dietary source.
2. FROM EPIBATIDINE TO DRUG-LIKE ANALGESICS
The isolation and characterization of epibatidine, like much of John Daly’s research, was responsible for a renewed interest in natural products as potential drug leads.2,3 The unique structure of epibatidine led to considerable interest in its synthesis and groups led by Broka,4 Shen,5 Baker6 and Corey7 synthesized epibatidine confirming its structure, with the latter three groups producing the enantiomers of the alkaloid. Corey and Baker provided the Daly group with the (+)- and (-)-enantiomers of epibatidine to determine that the latter was the natural alkaloid. Both enantiomers had analgesic activity that was blocked by mecamylamine, a nicotinic receptor (nAChR) antagonist.8 Unfortunately these promising analgesic actions, observed at doses as low as 10 ng/kg, were confounded by toxic effects e.g., emesis at doses slightly above this dose thus limiting the therapeutic index and the potential for therapeutic use. However, since epibatidine was non-selective in its interactions with the various subtypes of nAChR9 and its analgesic effects did not show tolerance,10 there remained considerable interest in the possibility that a nAChR subtype selective compound could represent a novel and effective analgesic agent. Certainly, based on its analgesic potency compared to morphine, its non-opioid mechanism of action and the observed lack of tolerance, epibatidine had many of the characteristics being actively sought for in new classes of analgesic agents.11
The lack of stereoselectivity of the enantiomers in their binding and functional interactions with nAChRs has been explained by both occupying similar space in the nAChR binding site12 with the chloropyridyl ring not being essential for activity.8
In 1993, a report in Science on the chemistry of epibatidine13 came to the attention of an Abbott scientist Yat Sun Or, who noted that the structure of epibatidine, the mechanism of action of which was not published until the following year, was structurally similar to compounds in a series of α4β2 nAChR selective ligands14 that Abbott scientists were investigating for potential use in the treatment of Alzheimer’s Disease (AD). The first of these, ABT-418 3 eventually failed in a Phase II trial for AD as did the reference standard at that time, nicotine 4, indicating a failed trial. This conclusion was reinforced when ABT-418 was demonstrated to have benefit in an acute trial in AD patients.15 In searching for additional indications16 from the broad library of nAChR agonists17 that existed at Abbott that also included ABT-089 5, Stephen Arneric, Mark Holladay and co-workers had initiated studies on the potential use of nAChR agonists as analgesics.18 Knowledge of the mechanism of action of epibatidine shared by Daly2 prior to publication of the seminal paper8 led to the identification of the pyridyl ether, A-87048 6 and the azetidine-2-pyridyl ether analog, A-85380 7 both of which had analgesic efficacy but retained some of the side effects observed with epibatidine.18
From a medicinal chemistry campaign that involved over 500 optimized compounds, ABT-594 8 was eventually identified.19,20 Like epibatidine, ABT-594 was 200-times more potent than morphine, lacking the tolerance and respiratory depression associated with the opioid.20 While ABT-594 reached Phase II trials as an analgesic, it was discontinued due to side-effect liabilities including emesis and nausea that were attributable to insufficient selectivity for the α4β2 nAChR versus the α3β4 subtype that is thought to be responsible for these side effects.21 Backup compounds to ABT-594 with reduced α3β4 nAChR activity included ABT-202 and ABT-894, the latter of which is currently in phase II trials for the treatment of pain associated with diabetic neuropathy.22
Additional efforts to improve the therapeutic index for nAChR as analgesics resulted in the diazabicycloheptane analog, A-366833 9, a potent analgesic agent in a variety of animal models with a reduced incidence of emesis.23 TC-6499 is another α4β2 nAChR agonist described as having “a superior…..profile compared to previously characterized analgesic compounds acting via nicotinic mechanisms” and entered Phase I trials as an analgesic in late 2007. Other epibatidine analogs of potential interest are UB-165 10, a hybrid of anatoxin-a and epibatidine24 and 4-nitro-PFEB (11; 2-fluoro-3-(4-nitro-phenyl) deschloroepibatidine)25 described as the most potent epibatidine analog (Ki = 9 pM) synthesized to date. From the extensive publicity around ABT-594,2 the songwriter, Paul Simon memorialized John’s activities in analgesia in the song, Senorita With A Necklace of Tears.26
Nothing but good news
There is a frog in South America
Whose venom is a cure
For all the suffering that mankind must endure
More powerful than morphine
And soothing as the rain
A frog in South America
Has the antidote for pain
Copyright Paul Simon 2000
3. THE JOHN DALY ROADMAP
Much of the research conducted by John Daly in the area of natural product chemistry during his 50 year tenure at the NIH has been viewed as primarily academic with little immediate application in the applied biomedical research realm of drug discovery. Consistent with this is the high likelihood (e.g. certainty) that this type of research would not have been funded outside the unique intramural research structure of the NIH and would have been given short shrift in a more applied environment, e.g., the pharmaceutical and biotech industries. While John’s field trips to Central America, Ecuador, Madagascar and other exotic climes have led to him being described as the “Indiana Jones of the NIH”, his many seminal contributions to biomedical research require more than a superficial comparison with a movie character.
Scientific research is a far from predictable process with serendipity playing a key role in major discoveries27 despite the current day reductionistic focus on molecular mechanisms. In this regard, the continued role of the basic research funded by the intramural programs at the NIH remains an invaluable and irreplaceable facet of biomedical research. Thus while Daly’s research was not considered “applied”, his seminal role in driving the potential for nAChR agonists as novel analgesics as documented above and his equally important work in the field of adenosine research28 had a major impact in drug discovery. In the adenosine area, Daly helped to define the relationship between adenosine action and adenylyl cyclase as a second messenger, and the inhibitory actions of alkylxanthines that led to the discovery of the adenosine A2A receptor and the recognition of its potential as a novel target for improved Parkinson’s Disease (PD) therapeutics.29
Prior to the NIH’s formalized Roadmap for Drug Discovery,30 John had, by default, established his own Roadmap making major contributions to both drug discovery and research, a reflection of his inquisitiveness, perseverance, and selfless interest in biomedical science and his collegial interactions and mentorship. These characteristics are reminiscent of the key attributes that James Black noted in a recent tribute to the famed drug hunter, Paul Janssen31 and which Black considered to be lacking in the current biomedical research environment, being replaced with superficiality. Daly’s reduction to practice of the concept of nicotinic analgesia, proposed in 193232 and some 62 years later in 1994,8 is a prime example of what Black termed commitment - never giving up on a new concept. Thus Daly’s perseverance sustained the 18 years from the original isolation of the frog skin analgesic in 19742 to the identification of its structure in 1992.1
John’s research legacy has thus transcended individual molecular targets and their associated chemistry to provide a viable, renewed interest in natural products as the source for new tools and as leads for therapeutic entities that enhance knowledge of disease etiology and provide the chemistry-driven means to identify new drugs.33 More recently Daly’s work even touched on pumilotoxins resident in frog skin as a natural defense mechanism against mosquitoes.34 This practical, concrete lead from nature contrasts with the abstract intellectual brainstorming on the same topic from Myhrvold and colleagues.35
4. CONCLUSION
A comparison between the discoveries of BTX36 and epibatidine is pertinent here, because it shows the different approaches taken by John according to circumstances. The expeditions that led to the BTXs originated from reports of poison dart frogs used by the native Indians in Colombia. The BTXs were major alkaloids in toxic secretions, in the case of the golden frog, Phyllobates terribilis, up to 1 mg/frog. With epibatidine, the expeditions to Ecuador were part of a screening of colorful dendrobatid frogs searching for the always interesting unknown. Following the bioassay experience gained with the BTXs, after extracting alkaloids from the Epipedobates tricolor frogs, John would use sc injection into a mouse to assess toxicity and consequently he serendipitously stumbled onto the Straub tail effect (as well as other very toxic effects). The S-T effect drove John’s renewed efforts in discovering the structure and mechanism of action of epibatidine, which was a trace component in the alkaloid extract, perhaps at 1-5 µg/frog. While the BTX work was a tour de force in the 1960s mainly for the isolation of these alkaloids, clever NMR experiments at the time and crystallization of a derivative for X-ray analysis, the epibatidine structural work followed less a linear accumulation of reliable data but was, over the period 1974 to the early 1990s, more of an up and down difficult journey with frequently confusing results, false premises, many detours and dead ends. At some point there was a small amount of epibatidine purified by HPLC, but NMR sensitivity was then inadequate to the task. That sample was then partly used in other experiments. The final sample, used only for microliter injections on GC-MS and GC-FTIR, contained major pumiliotoxin contaminants. No purifications were attempted for fear of losing the world’s last supply. The moratorium on this sample was lifted by John when we could demonstrate on a 5 microliter scale that an acetylation of the secondary NH would make epibatidine almost neutral (the other N of 208/210, we were convinced, was present in a weakly basic pyridine). The pumiliotoxins, being tertiary amines, would not react and could be removed with an acid extraction. Applied to the whole 200µL left, this yielded 0.75 mg of N-acetylepibatidine, and the structure was then analyzed by 1H-NMR. As a side story and bonus, the extracts of Epipedobates tricolor also led to the isolation (21 mg) and crystallization of pumiliotoxin 251D HCl, which provided the structure of this alkaloid through X-ray analysis.37 Structures of dozens of pumiliotoxins, found before and after, many with potent cardiotonic activity, were postulated based on 251D, 1. In some cases the proposed structures were confirmed later by NMR and synthesis. The E. tricolor extracts, what little remains, contain at least 80 alkaloids, some of them still unknown and barely seen in the background of a GC run. There are two other chlorine-containing alkaloids, an N-methyl epibatidine and phantasmidine (the frog is also called the phantasmal frog) whose structure is being elucidated.
The classical paradigm of targeting for investigation an interesting property described by cultural lore (such as the BTX quest), then identifying the active substance, is not antagonistic to another approach: the general screening of natural sources and then finding possibly interesting properties and compounds (such as epibatidine). The latter method is what we envision, with the utmost care, for John Daly’s NIH sample collection,38 a potential source for the “next epibatidines.”
ACKNOWLEDGEMENT
We thank Charles W. Myers, Curator Emeritus of the Dept. of Herpetology of the American Museum of Natural History, New York, for valuable comments on this discussion of one aspect of the large body of work shared with his longtime friend and collaborator, John Daly.
References
1. T. F. Spande, H. M. Garraffo, M. W. Edwards, H. J. C. Yeh, L. Pannell, and J. W. Daly, J. Am. Chem. Soc., 1992, 114, 3475. CrossRef
2. J. W. Daly, H. M. Garraffo, T. F. Spande, M. W. Decker, J. P. Sullivan, and M. Williams, Natural Prod. Rep., 2000, 17, 131. CrossRef
3. J. W. Daly, J. Nat. Prod., 1998, 61, 162. CrossRef
4. C. A. Broka, Tetrahedron Lett., 1993, 34, 3251. CrossRef
5. D. F. Huang and T. Y. Shen, Tetrahedron Lett., 1993, 34, 4477. CrossRef
6. S. R. Fletcher, R. Baker, M. S. Chambers, S. C. Hobbs, and P. J. Mitchell, J. Chem. Soc., Chem. Commun., 1993, 15, 1216. CrossRef
7. E. J. Corey, T. E. Loh, S. Achyutha-Rao, D. C. Daley, and S. Sarshar, J. Org. Chem., 1993, 58, 5600. CrossRef
8. B. Badio and J. W. Daly, Mol. Pharmacol., 1994, 45, 563.
9. J. P. Sullivan, M. W. Decker, J. Brioni, D. Donnelly-Roberts, D. A. Anderson, A. W. Bannon, C.-H. Kang, P. Adams, M. Piattoni-Kaplan, M. J. Buckley, M. Gopalakrishnan, M. Williams, and S. P. Arneric, J. Pharmacol. Exp. Ther., 1994, 271, 624.
10. M. I. Damaj and B. R. Martin, Eur. J. Pharmacol., 1996, 300, 51. CrossRef
11. A. D. Corbett, G. Henderson, A. T. McKnight, and S. J. Paterson, Br. J. Pharmacol., 2006, 147, (Supp 1), S153. CrossRef
12. M. Dukat, M. I. Damaj, W. Glassco, D. Dumas, E. L. May, B. R. Martin, and R. A. Glennon, Med. Chem. Res., 1994, 4, 131.
13. D. Bradley, Science, 1993, 261, 1117. CrossRef
14. M. W. Decker, J. Brioni, J. P. Sullivan, M. Buckley, J. L. Raszkiewicz, C.-H. Kang, W. Giardina, R. Radek, M. Williams, and S. P. Arneric, J. Pharmacol. Exp. Ther., 1994, 270, 319.
15. A. Potter, J. Corwin, J. Lang, M. Piasecki, R. Lenox, and P. A. Newhouse, Psychopharmacology (Berl.), 1999, 142, 334. CrossRef
16. G. K. Lloyd and M. Williams, J. Pharmacol. Exp. Ther., 2000, 292, 461.
17. M. W. Holladay, M. J. Dart, and J. K. Lynch, J. Med. Chem., 1997, 40, 4169. CrossRef
18. J. P. Sullivan, D. L. Donnelly-Roberts, C. A. Briggs, D. J. Anderson, M. Gopalakrishnan, M. Piattoni-Kaplan, J. E. Campbell, D. G. McKenna, E. Molinari, L. Monteggia, A.-M. Hettinger, D. S. Garvey, J. T. Wasicak, M. Williams, and S. P. Arneric, Neuropharmacol., 1996, 35, 725. CrossRef
19. M. W. Holladay, J. T. Wasicak, N.-H. Lin, Y. He, K. B. Ryther, A. W. Bannon, M. J. Buckley, D. J. B. Kim, M. W. Decker, D. J. Anderson, J. E. Campbell, T. A. Kuntzweiler, D. L. Donnelly-Roberts, M. Piattoni-Kaplan, C. A. Briggs, M. Williams, and S. P. Arneric, J. Med. Chem., 1998, 41, 407. CrossRef
20. A. W. Bannon, M. W. Decker, M. W. Holladay, P. Curzon, D. L. Donnelly-Roberts, P. S. Puttfarcken, R. S. Bitner, A. Diaz, A. H. Dickenson, R. D. Porsolt, M. Williams, and S. P. Arneric, Science, 1998, 289, 77. CrossRef
21. M. W. Holladay and M. W. Decker, Adv. Med. Chem., 2000, 5, 85. CrossRef
22. http://clinicaltrials.gov/ct2/show/NCT00507936?term=ABT894&rank=1.
23. J. Ji, W. H. Bunnelle, D. J. Anderson, C. Faltynek, T. Dyhring, P. K. Ahring, L. E. Rueter, P. Curzon, M. J. Buckley, K. C. Marsh, A. Kempf-Grote, and M. D. Meyer, Biochem. Pharmacol., 2007, 74, 1253. CrossRef
24. E. Wright, T. Gallagher, C. G. V. Sharples, and S. Wonnacott, Bioorg. Med. Chem. Lett., 1997, 7, 2867. CrossRef
25. F. I. Carroll, R. Ware, L. E. Brieaddy, H. A. Navarro, M. I. Damaj, and B. R. Martin, J. Med. Chem., 2004, 47, 4588. CrossRef
26. P. Simon, ‘Senorita with a necklace of tears. You’re The One.’, Warner Bros Records, 2000, CD 9:47844–5-2 .
27. D. Shaywitz and N. Taleb, ‘Drug Research Needs Serendipity’, Financial Times, 2008 FT.com July 29, 2008 .
28. B. B. Fredholm and K. A. Jacobson, This issue.
29. N. Simola, M. Morelli, and A. Pinna, Curr. Pharma Des., 2008, 14, 1475. CrossRef
30. E. Zerhouni, ‘The NIH Roadmap’, Science, 2003, 302, 63 . CrossRef
31. J. W. Black, ‘A personal perspective on Dr. Paul Janssen’, J. Med. Chem., 2005, 38, 1687. CrossRef
32. L. Davis, L. J. Pollock, and T. T. Stone, Surg. Gynecol. Obstet., 1932, 55, 418.
33. D. J. Newman, J. Med. Chem., 2008, 51, 2589. CrossRef
34. P. J. Weldon, M. Kramer, S. Gordon, T. F. Spande, and J. W. Daly, Proc. Natl. Acad. Sci. USA, 2006, 103, 17818. CrossRef
35. M. Gladwell, ‘In The Air. Annals of Innovation’, New Yorker, May 12, 2008.
36. H. M. Garraffo and T. F. Spande, Heterocycles, 2009, 79, 195. CrossRef
37. J. W. Daly, T. Tokuyama, T. Fujiwara, R. J. Highet, and I. L. Karle, J. Am. Chem. Soc., 1980, 102, 830. CrossRef
38. J. W. Daly, T. F. Spande, and H. M. Garraffo, J. Nat. Prod., 2005, 68, 1556. CrossRef