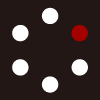
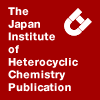
HETEROCYCLES
An International Journal for Reviews and Communications in Heterocyclic ChemistryWeb Edition ISSN: 1881-0942
Published online by The Japan Institute of Heterocyclic Chemistry
e-Journal
Full Text HTML
Received, 1st October, 2008, Accepted, 1st December, 2008, Published online, 4th December, 2008.
DOI: 10.3987/COM-08-S(D)58
■ Synthesis of a Novel trans-3’,4’-BNA Monomer Bearing a 4,8-Dioxa-5-azabicyclo[5.3.0]decane Skeleton
Tetsuya Kodama, Kensaku Sugaya, Takeshi Baba, Takeshi Imanishi, and Satoshi Obika*
Graduate School of Pharmaceutical Science, Osaka University, 1-6 Yamadaoka, Suita, Osaka 560-0871, Japan
Abstract
A novel trans-3’,4’-BNA monomer, in which sugar conformation was restricted to S-type by a trans-fused 3-oxa-4-azapentylene bridge between C3’ and C4’ position, was designed and synthesized. The trans-fused 7-membered cyclic structure within a 4,8-dioxa-5-azabicyclo[5.3.0]decane skeleton was prepared by means of intramolecular substitution reaction using potassium carbonate as a base. We found that all of protecting groups (benzyl, benzyloxymethyl, and benzyloxycarbonyl group) were able to be removed smoothly by DDQ oxidation followed by boron trichloride treatment without cleavage of N-O linkage to afford the desired trans-3’,4’-BNA monomer.INTRODUCTION
Chemically modified nucleic acid analogues that interact strongly with a complementary strand are of interest in medicinal chemistry and genome technology.1,2 We have synthesized a series of 2’-O,4’-C-bridged nucleic acids (2’,4’-BNAs3–6/LNA7), of which sugar puckering is exactly restricted to N-type, and have shown that they confer high binding affinity towards complementary RNA strands and superior resistance to enzymatic digestion.
Oligonucleotides containing a nucleoside analogue with S-type sugar puckering are expected to form stable B-type duplexes with complementary DNA and act as DNA mimics, and are also fascinating materials due to their potential applications in such technologies as DNA microarray8 and decoy strategy.9 Therefore, nucleoside analogues bearing a restricted S-type sugar conformation have been designed and synthesized to date, and some of them were introduced into oligonucleotides and the hybridizing properties were evaluated.10-16 However, these oligonucleotide analogues showed only small increase or
considerable decrease in the duplex stability, probably due to unfavorable restriction of the sugar conformation and/or steric repulsion caused by the additional structural components.
Recently, we synthesized several nucleic acid analogues with S-type sugar puckering, a series of trans-3’,4’-BNA,17-19 which have an additional trans-fused ring between C3’ and C4’ (Figure 1). In previous work, we showed that 2’-deoxy-trans-3’,4’-BNA monomer (2) was successfully incorporated into oligonucleotide, and the oligonucleotide formed duplexes with DNA and RNA complements as stable as natural DNA did.19 Because of the possibility of creating superior artificial S-type nucleic acids by fixing the sugar conformation but employing a more appropriate bridge structure, we reconsidered the sugar restriction strategy. First, because it was expected that the entropic advantage of conformational fixing during duplex formation was canceled out by unfavorable interactions, e.g. disturbance of the hydration network due to the additional hydrophobic bridge structure of 2, we planned to use a relatively hydrophilic bridge structure for sugar restriction. Second, bridge size would be enlarged for fine adjustment of sugar conformation. In B-type DNA duplex, S-type sugar conformation20 was mainly found and the pseudorotation phase angle (P)21 and a dihedral angle (δ) were in range of 86°-189° and 90°-159°, respectively (Figure 2).22 On the other hand, the 2’-deoxy-trans-3’,4’-BNA monomer (2) showed small difference in these numbers; 194° (P) and 174° (δ).19 In previous study, X-ray crystallography revealed that the P (174°) and δ (164°) of 3 with a 7-membered-bridge structure was similar with those of B-form
DNA.17 Based on the results, we here designed a novel trans-3’,4’-BNA monomer 1 bearing a relatively hydrophilic 3-oxa-4-azapentylene bridge between C3’ and C4’ position (Figure 1). An ab initio calculation using 6-31G* basis set for the trans-3’,4’-BNA monomer 1 estimated the P and δ value to be 173° and 164°, which met our expectation (Figure 2).
In this paper, we describe the synthesis of a novel trans-3’,4’-BNA monomer 1, via a cyclization reaction using potassium carbonate to form a trans-fused 7-membered bridge structure, 1,2-oxazepane.
RESULTS AND DISCUSSION
The desired trans-3’,4’-BNA 1 was synthesized from compound 4, which was prepared from thymidine according to previously reported methods.19 Compound 4 was treated under Mitsunobu reaction conditions using N-hydroxyphthalimide in THF to give phthalimide 5 in 90% yield (Scheme 1). The obtained phthalimide 5 was converted to a primary amine 6 using hydrazine hydrate. First, we attempted the bridge-forming reaction of amine 6 under various basic conditions, but the trans-3’,4’-BNA structure did not obtained. Previously, in synthesis of 2’,4’-BNANC, acylation or carbamation of its primary amino group was effective for the similar cyclization reaction to form the corresponding bridged structure whereas the cyclization of a naked amine derivative was failed.6 Based on this result, the amino group of 6 was protected with benzyloxycarbonyl (Cbz) group to give compound 7. Then, a cyclization reaction of
7 was investigated, with the result that the intramolecular substitution reation proceeded by treatment with K2CO3 in DMF at 80 °C to form compound 8 bearing the desired bridged structure in 67% yield. Neither CsCO3, sodium hexamethyldisilazide, nor sodium hydride gave sufficient results.
Deprotection conditions for a fully-protected trans-3’,4’-BNA 8 was next investigated, and the results were shown in Table 1. Reductive de-benzylation was attempted initially. Compound 8 was subjected to palladium hydroxide and ammonium formate (Run 1) or hydrogen gas (Run 2), but thymine base and N-O bond were found to undergo decomposition. Neither sodium in liquid ammonia (Run 3) nor lithium naphthalenide in THF (Run 4) gave the corresponding deprotected compounds. Acidic conditions were next examined. Whereas methanesulfonic acid (MsOH) as a strong Brønsted acid (Run 5) and timethylsilyl iodide (TMSI) as a Lewis acid (Run 6) did not produce the desired compound, use of boron trichloride at 0 °C afforded compound 9 on which a benzyl group at the O-3’ position remained in 19% yield (Run 7). Oxidative deprotection of 8 was attempted with excess 2,3-dichloro-5,6-dicyano-1,4-benzoquinone (DDQ) in wet dichloromethane under reflux.23 In this conditions, Cbz-protected compound 10 was obtained in good yield, although the reaction took a lot of
time to be completed (Run 8). By using wet 1,2-dichloroethane as a solvent in place of dichloromethane, the oxidative deprotection reaction proceeded smoothly to give 10 in a moderate yield (Run 9).
We finally accomplished synthesis of the desired trans-3’,4’-BNA 1 by treatment of compound 10 with boron trichloride in dichloromethane at 0 °C. The 1H NMR spectrum of 1 showed similar features to that of compound 3; e.g. H-1’ (δ 6.32, dd, J = 6 and 9 Hz) for 1 and (δ 6.32, dd, J = 6 and 9 Hz) for 3.19 This suggests the newly designed trans-3’,4’-BNA 1 is restricted to S-type conformation as expected by ab initio calculation.
CONCLUSION
We designed a novel trans-3’,4’-BNA monomer bearing a 4,8-dioxa-5-azabicyclo[5.3.0]decane skeleton which consists of a S-type-restricted sugar moiety and a relatively hydrophilic trans-fused bridge structure. The trans-fused 7-membered cyclic structure was prepared by intramolecular substitution reaction of Cbz-protected amino group. Deprotection of fully protected trans-3’,4’-BNA monomer was performed by subjection to DDQ followed by boron trichloride.
EXPERIMENTAL
Unless otherwise mentioned, all chemicals from commercial sources were used without further purification. Acetonitrile (MeCN), 1,2-dichloroethane, dichloromethane (CH2Cl2), and pyridine used in reaction were distilled from calcium hydride. Tetrahydrofuran (THF) was distilled from lithium aluminum hydride just before use. All reactions were performed under an atmosphere of nitrogen. Melting points were measured on a Yanagimoto micro melting points apparatus and are uncorrected. The 1H and 13C NMR spectra were recorded on JEOL EX-270 (1H, 270 MHz; 13C, 67.8 MHz), GX-500 (1H, 500 MHz), or Varian INOVA-600 (13C, 150.8 MHz) instruments. Values for δ are in ppm relative to tetramethylsilane or deuterated solvent as internal standard. The numbering used for NMR assignments is shown in Scheme 1. The IR spectra were recorded on a JASCO FT/IR-4200 spectrometer. The FAB-mass spectra were measured on a JEOL JMS-600 or JMS-700 mass spectrometer. Column chromatography was carried out using Fuji Silysia BW-127ZH, FL-100D silica gel for normal phase, and Nacalai Tesque Cosmosil 75C18-OPN for reverse phase.
3’,5’-Di-O-benzyl-3-N-benzyloxymethyl-3’-C-[2-(phthalimidyloxy)ethyl]-4’-C-(tosyloxymethyl)thy-midine (5). To a solution of 419 (454 mg, 0.589 mmol) in THF (10 mL) was added N-hydroxyphthalimide (115 mg, 0.707 mmol) and Ph3P (185 mg, 0.707 mmol) at rt, and the resulting mixture was stirred for 15 min. Diethyl azodicarboxylate (2.2 M in toluene, 0.320 mL, 0.71 mmol) was added to the reaction mixture on an ice-cooled bath. After stirred for 20 min, the resulting mixture was concentrated under reduced pressure. The obtained crude mixture was separated between Et2O and H2O, and the organic layer was washed with H2O and brine, dried over Na2SO4, and evaporated. The residue was purified by silica gel column chromatography [hexane:AcOEt (2:3)] to give 5 (485 mg, 90%) as white powder: mp 58–61°C. [α]D23 –3.7 (c 1.00, CHCl3). IR νmax (KBr): 1095, 1177, 1273, 1363, 1464, 1495, 1599, 1666, 1709, 1734, 1789, 2925, 3031 cm−1. 1H-NMR (CDCl3): δ 1.51 (3H, d, 5-Me, J = 1 Hz), 2.21 (1H, dd, H-2’, J = 10, 14 Hz), 2.29 (3H, s, tosyl-Me), 2.33-2.56 (2H, m, H-6’), 2.80 (1H, dd, H-2’, J = 5, 14 Hz), 3.78 (2H, s), 4.10 (1H, d, OCH2, J = 10 Hz), 4.15-4.36 (2H, m, H-7’), 4.32 (1H, d, OCH2, J = 10 Hz), 4.49 (1H, d, OCH2, J = 11 Hz), 4.50 (2H, s), 4.57 (2H, s), 4.61 (1H, d, OCH2, J = 11 Hz), 5.36 (2H, s, NCH2O), 6.12 (1H, dd, H-1’, J = 5, 10 Hz), 7.10-7.31 (17H, m), 7.51 (1H, d, H-5, J = 1 Hz), 7.63-7.80 (6H, m). 13C-NMR (CDCl3):δ 12.9, 21.7, 30.5, 40.0, 64.6, 70.0, 70.4, 71.5, 72.1, 73.8, 74.0, 83.9, 85.8, 88.4, 110.0, 123.5, 127.0, 127.5, 127.5, 127.6, 128.0, 128.1, 128.4, 128.6, 128.7, 129.6, 132.0, 134.3, 134.5, 136.5, 136.9, 137.8, 144.8, 150.8, 163.1, 163.2. FAB-MS: m/z 916 (MH+). High-resolution FAB-MS: Calcd for C50H50N3O12S (MH+): 916.3125. Found: 916.3115.
3’-C-[2-(Aminoxy)ethyl]-3’,5’-di-O-benzyl-3-N-benzyloxymethyl-4’-C-(tosyloxymethyl)thymidine (6). To a solution of 5 (485 mg, 0.505 mmol) in CH2Cl2 (5 mL) was added hydrazine monohydrate (32 mg, 0.64 mmol) at rt, and the resulting mixture was stirred for 15 min. The resulting mixture was concentrated under reduced pressure, and the residue was purified by silica gel column chromatography [hexane:AcOEt (1:1)] to give 6 (367 mg, 93%) as white powder: mp 39 – 41 °C. [α]D24 –13.2 (c 1.00, MeOH). IR νmax (KBr): 1094, 1176, 1274, 1362, 1455, 1496, 1597, 1667, 1709, 2925, 3031, 3064, 3322 cm−1. 1H-NMR (CDCl3): δ 1.57 (3H, d, 5-Me, J = 1 Hz), 2.20 (1H, dd, H-2’, J = 10, 14 Hz), 2.24-2.31 (2H, m, H-6’), 2.41 (3H, s, tosyl-Me), 2.69 (1H, dd, H-2’, J = 5, 14 Hz), 3.72-3.86 (2H, m, H-7’), 3.88 (1H, d, J = 11 Hz), 3.95 (1H, d, J = 11 Hz), 4.16 (1H, d, J = 11 Hz), 4.37 (1H, d, J = 11 Hz), 4.40 (1H, d, J = 11 Hz), 4.48 (1H, d, J = 11 Hz), 4.55 (1H, d, J = 11 Hz), 4.64 (1H, d, J = 11 Hz), 4.64 (2H, s, PhCH2O), 5.42 (2H, s, NCH2O), 6.17 (1H, dd, H-1’, J = 5, 10 Hz), 7.17-7.58 (15H, m), 7.62-7.74 (5H, m). 13C-NMR (CDCl3): δ 13.0, 21.7, 30.9, 40.9, 65.1, 71.8, 72.2, 73.1, 74.6, 85.4, 87.8, 89.8, 110.5, 128.2, 128.4, 128.5, 128.5, 128.7, 128.9, 129.1, 129.1, 129.4, 129.5, 130.8, 133.6, 135.2, 136.5, 138.7, 139.1, 139.4, 146.4, 152.0, 164.9. FAB-MS: m/z 786 (MH+). High-resolution FAB-MS: Calcd for C42H48N3O10S (MH+): 786.3052. Found: 786.3060.
3’,5’-Di-O-benzyl-3’-C-[2-(N-benzyloxycarbonylaminoxy)ethyl]-3-N-benzyloxymethyl-4’-C-(tosyl- oxymethyl)thymidine (7). To a solution of 6 (340 mg, 0.434 mmol) in pyridine (8.7 mL) was added benzyl chloroformate (89.0 mg, 0.521 mmol) on an ice-cooled bath, and the resulting mixture was stirred for 20 min at rt. After addition of saturated aqueous NaHCO3, the mixture was extracted with AcOEt. The organic layer was washed with H2O and brine, dried over Na2SO4, and evaporated. The residue was purified by silica gel column chromatography [hexane:AcOEt (2:1)] to give 7 (390 mg, 98%) as white powder: mp 43–46 °C. [α]D24 +2.4 (c 1.00, CHCl3). IR νmax (KBr): 1096, 1176, 1242, 1362, 1455, 1496, 1599, 1666, 1709, 1748, 2887, 2956, 3032, 3064, 3268 cm−1. 1H-NMR (CDCl3): δ 1.56 (3H, s, 5-Me), 2.19 (1H, dd, H-2’, J = 10, 14 Hz), 2.23-2.45 (2H, m, H-6’), 2.39 (3H, s, tosyl-Me), 2.70 (1H, dd, H-2’, J = 5, 14 Hz), 3.80 (1H, d, J = 11 Hz), 3.90 (1H, d, J = 11 Hz), 4.02-4.13 (2H, m, H-7’), 4.09 (1H, d, J = 10 Hz), 4.35 (1H, d, J = 10 Hz), 4.35 (1H, d, J = 11 Hz), 4.47 (1H, d, J = 11 Hz), 4.49 (1H, d, J = 11 Hz), 4.61 (1H, d, J = 11 Hz), 4.64 (2H, s, PhCH2O), 5.17 (2H, s, PhCH2O), 5.42 (2H, s, NCH2O), 6.17 (1H, dd, H-1’, J = 5, 10 Hz), 7.16-7.35 (22H, m), 7.58 (1H, s, H-6), 7.66-7.70 (3H, m). 13C-NMR (CDCl3): δ 13.2, 21.8, 30.5, 40.4, 65.1, 67.7, 71.4, 71.5, 71.7, 72.5, 72.7, 74.2, 84.8, 87.2, 89.1, 110.3, 128.2, 128.3, 128.4, 128.5, 128.6, 128.8, 128.9, 129.1, 129.4, 129.4, 129.5, 130.8, 133.1, 135.5, 137.3, 138.4, 139.0, 139.4, 146.3, 151.8, 158.0, 164.0. FAB-MS: m/z 920 (MH+). High-resolution FAB-MS: Calcd for C50H54N3O12S (MH+): 920.3428. Found: 920.3417.
3’,5’-Di-O-benzyl-3’-C,4’-C-(N-benzyloxycarbonyl-3-oxa-4-azapentylene)-3-N-benzyloxymethylthy-midine (8). A mixture of 7 (78 mg, 0.085 mmol) and K2CO3 (94 mg, 0.68 mmol) in DMF (3 mL) was stirred for 48 h at 80 °C. After neutralization with diluted aqueous HCl, the mixture was extracted with AcOEt. The organic layer was washed with H2O and brine, dried over Na2SO4, and concentrated under reduced pressure. The residue was purified by silica gel column chromatography [hexane:AcOEt (5:2)] to give 8 (35 mg, 67%) as white powder: mp 49–52 °C. [α]D23 –27.7 (c 1.00, CHCl3). IR νmax (KBr): 1183, 1211, 1262, 1300, 1362, 1412, 1453, 1496, 1659, 1707, 2871, 2950, 3031, 3064 cm−1. 1H-NMR (CDCl3): δ 1.61 (3H, s, 5-Me), 2.00-2.18 (2H, m, H-6’), 2.37 (1H, dd, H-2’, J = 9, 14 Hz), 2.81 (1H, dd, H-2’, J = 5, 14 Hz), 3.39 (1H, d, J = 12 Hz), 3.63 (1H, d, J = 11 Hz), 4.06 (2H, brs, H-7’), 4.20 (1H, d, J = 12 Hz), 4.20 (1H, d, J = 11 Hz), 4.30 (1H, d, J = 11 Hz), 4.52 (2H, s, PhCH2O), 4.54 (1H, d, J = 11 Hz), 4.60 (2H, s, PhCH2O), 4.85 (1H, d, J = 12 Hz), 5.04 (1H, d, J = 12 Hz), 5.38 (2H, s, NCH2O), 6.30 (1H, dd, J = 5, 9 Hz), 7.16-7.30 (20H, m), 7.73 (1H, s, H-6). 13C-NMR (CDCl3): δ 13.8, 22.2, 29.3, 39.6, 52.0, 64.2, 68.3, 71.1, 72.8, 74.5, 84.8, 84.9, 86.6, 87.0, 89.8, 110.2, 127.7, 128.1, 128.2, 128.3, 128.6, 128.7, 128.9, 128.9, 129.1, 129.3, 134.8, 135.5, 136.5, 137.2, 137.4, 137.9, 138.5, 146.1, 151.6, 164.0, 184.2. FAB-MS: m/z 748 (MH+). High-resolution FAB-MS: Calcd for C43H46N3O9 (MH+): 748.3234. Found: 748.3246.
3’-O-Benzyl-3’-C,4’-C-(3-oxa-4-azapentylene)thymidine (9). To a solution of 8 (15.0 mg, 0.020 mmol) in CH2Cl2 (1 mL) was added BCl3 (1 M in hexane, 0.20 mL, 0.20 mmol) at –78 °C, and the resulting mixture was stirred for 1 h. After stirring for 6 h at 0 °C, MeOH was added to the reaction mixture at the same temperature. The resulting mixture was concentrated to give a crude mixture, which was purified by silica gel column chromatography [CHCl3:MeOH (15:1)] to give 9 (1.5 mg, 19%) as a white foam. 1H-NMR (CD3OD) δ: 1.79 (3H, d, 5-Me, J = 1 Hz), 1.89-2.01 (1H, m, H-6’), 2.23-2.29 (1H, m, H-6’), 2.34 (1H, dd, H-2’, J = 9, 14 Hz), 2.76 (1H, dd, H-2’, J = 5, 14 Hz), 3.06 (1H, d, H-8’, J = 13 Hz), 3.20 (1H, d, H-8’, J = 13 Hz), 3.78-3.89 (1H, m, H-7’), 3.85 (1H, d, J = 11 Hz), 3.98-4.08 (1H, m, H-7’), 4.01 (1H, d, J = 11 Hz), 4.46 (1H, d, J = 11 Hz), 4.56 (1H, d, J = 11 Hz), 6.21 (1H, dd, H-1’, J = 5, 9 Hz), 7.07-7.60 (5H, m), 8.13 (1H, d, H-6, J = 1 Hz). FAB-MS: m/z 404 (MH+). High-resolution FAB-MS: Calcd for C20H26N3O6 (MH+): 404.1823. Found: 404.1825.
3’-C,4’-C-(N-Benzyloxycarbonyl-3-oxa-4-azapentylene)thymidine (10). To a solution of 8 (70.0 mg, 0.094 mmol) in wet 1,2-dichloroethane (2.0 mL) was added DDQ (213 mg, 0.94 mmol), and the mixture was refluxed for 6 h. After saturated aqueous NaHCO3 and saturated aqueous Na2S2O3 were added to the reaction mixture at rt, the resulting mixture was extracted with AcOEt. The organic layer was washed with H2O, saturated aqueous NaHCO3, and brine, dried over Na2SO4, and evaporated. The residue was purified by silica gel column chromatography [CHCl3:MeOH (6:1)] to give 10 (23 mg, 57%) as a white solid. Colorless crystals from hexane:AcOEt (1:1): mp 139-141 °C. [α]D24 +100.9 (c 0.80, MeOH). IR νmax (KBr): 1034, 1139, 1267, 1356, 1453, 1694, 3351 cm−1. 1H-NMR (CD3OD): δ 1.80-1.88 (1H, m, H-6’), 1.88 (3H, d, 5-Me, J = 1 Hz), 2.15-2.25 (1H, m, H-6’), 2.32 (1H, dd, H-2’, J = 6, 13 Hz), 2.57 (1H, dd, H-2’, J = 9, 13 Hz), 3.60 (1H, d, J = 12 Hz), 3.86 (1H, d, J = 12 Hz), 3.90 (1H, d, J = 12 Hz), 4.06 (1H, d, J = 12 Hz), 4.10-4.32 (2H, m, H-7’), 5.17 (2H, s), 6.42 (1H, dd, H-1’, J = 6, 9 Hz), 7.28-7.40 (5H, m), 8.19 (1H, d, H-6, J = 1 Hz). 13C-NMR (CD3OD): δ 12.6, 30.9, 34.2, 46.4, 51.1, 67.5, 68.6, 74.0, 82.0, 87.1, 90.3, 110.9, 128.7, 129.0, 129.4, 137.6, 138.7, 152.3, 156.5, 166.3. FAB-MS: m/z 448 (MH+). High-resolution FAB-MS: Calcd for C21H26N3O8 (MH+): 448.1720. Found: 448.1710.
3’-C,4’-C-(3-Oxa-4-azapentylene)thymidine (1). To a solution of 10 (19.0 mg, 0.042 mmol) in CH2Cl2 (1 mL) was added BCl3 (1 M in hexane, 0.13 mL, 0.13 mmol) at 0 °C and the resulting mixture was stirred for 1 h at rt. After AcOEt was added to the reaction mixture at 0 °C, the mixture was extracted with H2O. The obtained aqueous layer was washed with AcOEt and concentrated to give crude mixture, which was purified by C18 reverse phase column chromatography [MeCN:H2O (10:1)] to give 1 (9.3 mg, 70%) as a white solid. White powder from MeOH:H2O (1:9): mp >300 °C. 1H-NMR (D2O): δ 1.83 (3H, s, 5-Me), 1.88-2.05 (2H, m, H-6’), 2.38 (1H, dd, H-2’, J = 6, 14 Hz), 2.46 (1H, dd, H-2’, J = 9, 14 Hz), 3.06 (1H, d, J = 13 Hz), 3.46 (1H, d, J = 13 Hz), 3.76 (1H, d, J = 12 Hz), 3.91 (1H, d, J = 12 Hz), 4.01-4.66 (2H, m, H-7’), 6.32 (1H, dd, H-1’, J = 6, 9 Hz), 7.83 (1H, s, H-6). 13C-NMR (5% CD3CN in D2O): δ 12.9, 33.4, 43.8, 53.5, 66.9, 71.9, 84.1, 87.9, 91.1, 111.9, 120.2, 138.7, 153.4, 168.3. FAB-MS: m/z 314 (MH+). High-resolution FAB-MS: Calcd for C13H19N3O6 (MH+): 314.1354. Found: 314.1349.
ACKNOWLEDGEMENTS
This work was partly supported by a grant from a Grant-in-Aid for Science Research from the Ministry of Education, Culture, Sports, Science and Technology of Japan and the Japan Society for the Promotion of Science. We thank Dr. N. Kotoku (Gradate School of Pharmaceutical Sciences, Osaka University) for 13C NMR spectrometry measurements.
References
1. M. Koizumi, Curr. Top. Med. Chem., 2007, 7, 661. CrossRef
2. N. K. Saru, G. Shilakari, A. Nayak, and D. V. Kohli, Curr. Pharm. Biotechnol., 2007, 8, 291. CrossRef
3. T. Imanishi and S. Obika, Chem. Commun., 2002, 1653. CrossRef
4. S. Obika, D. Nanbu, Y. Hari, K. Morio, Y. In, T. Ishida, and T. Imanishi, Tetrahedron Lett., 1997, 38, 8735; CrossRef S. Obika, D. Nanbu, Y. Hari, J. Andoh, K. Morio, T. Doi, and T. Imanishi, Tetrahedron Lett., 1998, 39, 5401. CrossRef
5. Y. Hari, S. Obika, R. Ohnishi, K. Eguchi, T. Osaki, H. Ohishi, and T. Imanishi, Bioorg. Med. Chem., 2006, 14, 1029; CrossRef Y. Mitsuoka, T. Kodama, R. Ohnishi, Y. Hari, T. Imanishi, and S. Obika, Nucleic Acids Res., in press.
6. K. Miyashita, S. M. A. Rahman, S. Seki, S. Obika, and T. Imanishi, Chem. Commun., 2007, 3765; CrossRef S. M. A. Rahman, S. Seki, K. Utsuki, S. Obika, K. Miyashita, and T. Imanishi, Nucleosides Nucleotides Nucleic Acids, 2007, 26, 1625; CrossRef S. M. A. Rahman, S. Seki, S. Obika, S. Haitani, K. Miyashita, and T. Imanishi, Angew. Chem. Int. Ed., 2007, 46, 4306; CrossRef S. M. A. Rahman, S. Seki, S. Obika, H. Yoshikawa, K. Miyashita, and T. Imanishi, J. Am. Chem. Soc., 2008, 130, 4886. CrossRef
7. J. Wengel, Acc. Chem. Res., 1999, 32, 301; CrossRef A. A. Koshkin, P. Nielsen, M. Meldgaard, V. K. Rajwanshi, S. K. Sing, and J. Wengel, J. Am. Chem. Soc., 1998, 120, 13252; CrossRef V. K. Rajwanshi, A. E. Hakansson, M. D. Sørensen, S. Pitsch, S. K. Sing, R, Kumar, P. Nielsen, and J. Wengel, Angew. Chem. Int. Ed., 2000, 39, 1656. CrossRef
8. For a recent review: S. Audrey, D. L.-B. Bëatrice, and J. B. Loïc, Chem. Rev., 2008, 108, 109. CrossRef
9. For reviews: Y. S. Cho-Chung, Y. G. Park, and Y. N. Lee, Curr. Opin. Mol. Ther., 1999, 1, 386; R. Morishita, M. Aoki, and Y, Kaneda, Curr. Drug Targets, 2000, 1, 15; CrossRef N. Tomita, T. Ogihara, and R. Morishita, Curr. Drug Targets, 2003, 4, 603; CrossRef I. K. Lee, D. J. Ahn, H. S. Kim, J. Y. Park, and K. U. Lee, Curr. Drug Targets, 2003, 4, 619. CrossRef
10. S. Obika, M. Onoda, K. Morita, J. Andoh, M. Koizumi, and T. Imanishi, Chem. Commun., 2001, 1992; S. Obika, O. Nakagawa, A. Hiroto, Y. Hari, and T. Imanishi, Chem. Commun., 2003, 2202; CrossRef S. Obika, M. Sekiguchi, R. Somjing, and T. Imanishi, Angew. Chem. Int. Ed., 2005, 44, 1944. CrossRef
11. T. Kodama, C. Matsuo, H. Ori, S. Obika, K. Miyashita, and T. Imanishi, Nucleic Acids Symp. Ser., 2007, 51, 159. CrossRef
12. M. Tarköy, M. Bolli, B. Schweizer, and C. Leumann, Helv. Chim. Acta, 1993, 76, 481; CrossRef M. Tarköy, M. Bolli, and C. Leumann, Helv. Chim. Acta, 1994, 77, 716; CrossRef M. Bolli and C. Leumann, Angew. Chem., Int. Ed. Engl., 1995, 34, 694; CrossRef M. Bolli, J. C. Litten, R. Schütz, and C. J. Leumann, Chem. Biol., 1996, 3, 197; CrossRef M. Bolli, H. U. Trafelet, and C. Leumann, Nucleic Acids Res., 1996, 24, 4660. CrossRef
13. R. Steffens and C. Leumann, Helv. Chim. Acta, 1997, 80, 2426; CrossRef R. Steffens and C. J. Leumann, J. Am. Chem. Soc., 1997, 119, 11548; CrossRef R. Steffens and C. J. Leumann, J. Am. Chem. Soc., 1999, 121, 3249; CrossRef D. Renneberg and C. J. Leumann, J. Am. Chem. Soc., 2002, 124, 5993. CrossRef
14. K.-H. Altmann, R. Imwinkelried, R. Kesselring, and G. Rihs, Tetrahedron Lett., 1994, 35, 7625; CrossRef V. E. Marquez, P. Wang, M. C. Nicklaus, M. Maier, M. Manoharan, J. K. Christman, N. K. Banavali, and A. D. Mackerell Jr., Nucleosides Nucleotides Nucleic Acids, 2001, 20, 451; CrossRef M. A. Maier, Y. Choi, H. Gaus, J. J. Jr. Barchi, V. E. Marquez, and M. Manoharan, Nucleic Acids Res., 2004, 32, 3642. CrossRef
15. P. Nielsen, H. M. Pfundheller, C. E. Olsen, and J. Wengel, J. Chem. Soc., Perkin Trans. 1, 1997, 3423. CrossRef
16. J. Ravn, N. Thorup, and P. Nielsen, J. Chem. Soc., Perkin Trans. 1, 2001, 1855.
17. S. Obika, M. Sekiguchi, T. Osaki, N. Shibata, M. Masaki, Y. Hari, and T. Imanishi, Tetrahedron Lett., 2002, 43, 4365; CrossRef S. Obika, T. Osaki, M. Sekiguch, R. Somjing, Y. Harada, and T. Imanishi, Tetrahedron Lett., 2004, 45, 4801. CrossRef
18. M. Sekiguch, S. Obika, Y. Harada, T. Osaki, R. Somjing, Y. Mitsuoka, N. Shibata, M. Masaki, and T. Imanishi, J. Org. Chem., 2006, 71, 1306. CrossRef
19. K. Sugaya, Y. Harada, Y. Mitsuoka, T. Osaki, S. Obika, T. Kodama, and T. Imanishi, Nucleic Acids Symp. Ser., 2007, 51, 155; CrossRef T. Osaki, S. Obika, Y. Harada, Y. Mitsuoka, K. Sugaya, M. Sekiguchi, S. Roongjang, and T. Imanishi, Tetrahedron, 2007, 63, 8977; CrossRef T. Kodama, K. Sugaya, Y. Harada, Y. Mitsuoka, T. Imanishi, and S. Obika, Heterocycles, 2009, 77, 1209. CrossRef
20. W. Saenger, Principles of Nucleic Acid Structure, Springer, New York, 1984, .
21. C. Altona and M. Sundaralingam, J. Am. Chem. Soc., 1972, 94, 8250. CrossRef
22. G. G. Privé, K. Yanagi, and R. E. Dickerson, J. Mol. Biol., 1991, 217, 177. CrossRef
23. N. Ikemoto and S. L. Schreiber, J. Am. Chem. Soc., 1992, 114, 2524. CrossRef