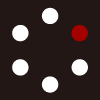
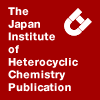
HETEROCYCLES
An International Journal for Reviews and Communications in Heterocyclic ChemistryWeb Edition ISSN: 1881-0942
Published online by The Japan Institute of Heterocyclic Chemistry
e-Journal
Full Text HTML
Received, 2nd October, 2008, Accepted, 1st December, 2008, Published online, 10th December, 2008.
DOI: 10.3987/REV-08-SR(D)6
■ Discovery of Batrachotoxin: The Launch of the Frog Alkaloid Program at NIH
H. Martin Garraffo* and Thomas F. Spande
Laboratory of Bioorganic Chemistry, Bldg. 8, 1A-15, National Institute of Health, 8 Center Drive MSC 0820, Bethesda, MD 20892, U.S.A.
Abstract
The determination of the structures of the batrachotoxins (BTXs), extremely toxic steroidal alkaloids found in the skins of the dart-poison frogs of the genus Phyllobates from Colombia in the 1960s is reviewed. The BTXs function by locking open sodium-ion channels of nerve and muscle, thereby depolarizing them. The structures and pharmacology of the BTXs were determined by a team led by John W. Daly. This research started a 40 year long study of alkaloids from frog skin, whereby John and his team identified and/or characterized more than 800 such alkaloids. The source of the BTXs, not synthesized but sequestered from diet by the frogs, is briefly discussed, in the context of the occurrence of BTXs in birds of Papua New Guinea and in a small melyrid beetle found there. Emphasized is the critical importance of maintaining and safe-guarding the large collection of frog-skin extracts and data accumulated since.INTRODUCTION
In the following review, we, as long-time collaborators of John Daly, will trace the history of the batrachotoxins (BTXs), a frog-skin alkaloid class discovered during the early days of John’s collecting trips in the 1960’s that started and fueled John’s alkaloid program at NIH. We will show the stubborn tenacity of John and a few colleagues in obtaining the structures and demonstrating the mode of action of the dart poisons and in purifying an incredible quantity of these substances considering their toxicity and the personal risks associated with acquiring and handling them. We will consider the importance of having the world’s supply of BTXs and John’s generosity in providing any scientist with research samples. The publication of work on the batrachotoxins triggered an immediate interest in graduate student, Charles W. Myers, on alkaloids in other dendrobatid frogs, in particular Dendrobates pumilio in Panama. A life-long collaboration developed between John Daly and Charles Myers who went on to collect dendrobatids throughout their range in Central and South America and led to the discovery of alkaloids of more than twenty-six classes with more than 800 structures. We will jump from frogs to birds to beetles to establish a possible link through BTXs and to speculate on the origins of these alkaloids. Last, but certainly not least, we will stress the importance of John Daly’s collection of samples and associated records in what could be considered a call to arms to cherish and preserve this treasure trove and to use it in the future with improved technologies and with ultimate care, for the advancement of science.
BATRACHOTOXINS
Of the hundreds of frog skin extracts John Daly acquired over 44 years of collecting, two will occupy our attention; those of the genus Phyllobates and those of another dendrobatid genus, Epipedobates. The unraveling of the structure and action of the blow dart poison from the former, known to be used by the Chocó Indians of Colombia provided the impetus for John’s study of alkaloids in other brightly colored frogs of the huge family Dendrobatidae and three other families (Bufonidae, Myobatrachidae and Mantellidae), where they likely serve in defense. The collections of Epipedobates tricolor and the discovery of the potent analgesic epibatidine will form the basis of another article in this issue (see Samples and Records below).
The collections of Phyllobates species comprised mainly P. aurotaenia (Boulenger) (1) with lesser amounts of P. terribilis Myers, Daly and Malkin (2) and some P. bicolor Bibron in Sagra (3). These were obtained over a 17 year period. The expeditions were as follows:
1) August, 1962: Fritz Märki and Marté Latham, Playa de Oro at headwaters of Río San Juan, Chocó province; 330 frogs (1). M. Latham (explorer-collector) had earlier shipped live frogs air-freight to NIH but all died so skinning was done in the field by Latham and extracts prepared by Märki.1 The frog 1 was misidentified in early work1 as 3.
2) Jan.-April, 1964: J. Daly and M. Latham: 2400 frogs (1), same site.
3) Jan.-April, 1966: J. Daly and M. Latham, ca. 5000 frogs (1), same site,
4) 1968: purchase of 140 skins from M. Latham (1), same site.
5) Feb., 1970: J. Daly and Charles Myers, 972 frogs (1), same site.
6) Feb., 1971: J. Daly and C. Myers, 850 frogs (1), same site.
7) 1973: J. Daly and C. Myers, 1290 frogs (2), Río Saija, Valle Cauca.
8) 1977: J. Daly and C. Myers, 100 frogs from Eva Bartels-Bernal (1); Aug.: same location as collections 1-6, 24 frogs (3); Sept.: Risaralda.
9) in 1979: J. Daly and C. Myers, raised 3 frogs (2) for electrophysiology studies.
DETERMINATION OF STRUCTURES
A 1986 review2 details the isolation, purification, X-ray, mass and 1H-NMR spectral determination of structure and biological activity of the blow dart poison obtained from Phyllobates species 1-3. Other reviews1,4,9 provide history on the use of batrachotoxin (BTX) in poisoning blow darts mainly for killing game. An entertaining review in Spanish by Bernhard Witkop, who coined the name batrachotoxin after organizing the NIH-quest for the toxin, summarizes early work;4 other reviews cover 1970 through 1993.5-13
A 1999 review3 provides additional references to the rapidly expanding literature on the pharmacology and derivatives of BTX as research tools to investigate the Na+ channel in nerve cells.
The early isolation and column chromatography procedures1 used in later work with little change, were based on discovering that the toxin was a basic alkaloid with 90% extractable on exposure of frog skins to 70% methanol. It was detectible on TLC with iodine vapor, had a UV λmax, 265 nm and IR absorptions for νOH, νNH and νC=O. Preliminary HRMS by Klaus Biemann14,15 indicated the likelihood of a steroidal structure for the “kokoi venom”, i.e. C17 ions with 9 double-bond equivalents.
Biological studies were reported using rat diaphragm phrenic nerve preparations where purified venom blocked electrical stimulation. The inference that the “kokoi venom” was interfering with Na+ transport proved correct.1 It was shown, curiously, that the toxin’s activity in crude extracts persisted after 5 minutes of heating at 100 °C, but after purification, was much less stable.1 Dead frogs rapidly lost activity necessitating prompt processing. The purified toxin is the most potent non-protein toxin known at present.
Daly’s notebook records a typical bio-assay following the subcutaneous injection used earlier in mice:1 From 2 µg of pure BTX: “short rapid movement, almost hopping around. Body in spasm, gasping for breath, convulsions, dead in one minute.” Highly purified BTX indicated the LD50 for mice is 2 µg/kg;16 earlier work indicated 2.7 µg/kg.1
The Daly group reported15 that the crude alkaloid extract was not homogeneous but consisted of three major components and several minor ones. The ratios varied from species and populations collected but the following was typical of major components in 2: BTX (1.6 parts), an “iso” BTX, shown (see below) to be homo BTX (hBTX) (1.0 part), batrachotoxinin A (BTX A, a less active hydrolysis product of BTX) (1.2 parts).
The frogs required great care in skinning, particularly 2, which contained approximately 1 mg toxin per frog and was considered very dangerous to handle.9 Daly would have skillfully skinned all of these as well as hundreds more of 1 and 3. Latham had found it cumbersome to wear gloves during skinning and consequently experienced a year-long partial paralysis of her right hand, merely from working with hundreds of frog skins of the 1/20th less toxic species 1.4
The definitive mode of action was shown by Edson Albuquerque, Daly and colleagues to involve Na+-flux disturbance in mammalian muscle nerve cells.13 The action of BTX or hBTX leads to the rapid irreversible depolarization of these cells by a flood of sodium ion.
John’s observation that p-dimethylaminobenzaldehyde (Ehrlich’s reagent) or the cinnamaldehyde analog provided sensitive detection of BTX but were negative for BTX A implied a pyrrole moiety was missing in the latter.15 Initially, a congener of BTX was observed, having a slightly higher Rf on TLC and was also Ehrlich-positive, but since it also gave the same misleading mass spectral thermal elimination product as determined14,15 by Biemann for BTX (m/z 399, C24H33NO4); it was considered an “isobatrachotoxin”. Thermally generated odd mass (neutral) fragments observed14,15 in mass spectra at MIT and later at NIH with John and Henry Fales, indicated formulae C7H9NO2 and C8H11NO2 for those fragments. Takashi Tokuyama recognized these as likely deriving from the two missing pyrrole moieties of BTX and hBTX respectively.16
From the replacement of a pyrrole methyl singlet by an ethyl triplet in the 1H-NMR spectra, Tokuyama deduced16 that “iso BTX” was an ethyl homolog of BTX and the name homo BTX (hBTX) was adopted. BTX A is 1000 times less toxic than BTX or hBTX and requires 20 µg to kill a mouse.
At this point, Tokuyama synthesized and purified the ester BTX-A-O-p-bromobenzoate and provided crystals suitable for X-ray diffraction analysis.17,18 Isabella and Jerome Karle were successful in rapidly solving the structure and showed it to be an unprecedented steroidal alkaloid with the following novel elements: a fused N-methylhomomorpholine ring between rings C and D and a hemiketal formed with a 9α hydroxyl and the 3-ketone (Figure 1).
The correct pyrrole ester moiety, lacking in BTX A, was determined after UV and 1H-NMR work by Tokuyama on various dimethylpyrrole 2- and 3-carboxylic acid esters where esters of 2-carboxylic acids were excluded by UV spectral comparison. By observing upfield and downfield shifts resulting from C6D6 vs. CDCl3 solvents on the methyl or ethyl methylene NMR signals of various pyrrole models and BTX and hBTX, he arrived at the only possible structures for BTX and hBTX, i.e. esters of BTX A with 2,4-dimethylpyrrole-3-carboxylic acid or 2-ethyl-4-methylpyrrole-3-carboxylic acid (Figure 1). A confirmatory partial synthesis of BTX was achieved by Tokuyama from BTX A and a mixed anhydride of 2,4-dimethylpyrrole-3-carboxylic acid and ethylchloroformate.16
Purification of extracts by thin-layer chromatography led to significant losses in activity due to oxidation. Column chromatography on silica, however, was satisfactory; a total of 5000 skins of 1, provided 11 mg BTX, 16 mg hBTX and 46 mg BTX A. HPLC purification, used later, on 426 skins of 2 yielded 175 mg BTX and 113 mg hBTX.20
Table 5 in reference 16, lists mouse LD50 values of six synthetic BTX-A di- and tri-substituted pyrrole esters. These demonstrated that a 2-alkyl substituent was essential in maintaining maximal toxicity, likely by retarding hydrolysis. Surprisingly, BTX-A-O-benzoate when compared with the O-p-bromobenzoate was nearly as active as BTX; the latter is less than 1000 times as active. The O-benzoate also indicated that a hydrogen-bond from a pyrrole NH is not essential for activity. BTX-A supplied by John to New England Nuclear, has provided BTX-A-O-p-[3H]-benzoate as a ligand for neuropharmacology studies.
WHAT REMAINS TO BE DONE?
Unresolved chemical questions remain: A “pseudo batrachotoxin”, presumably a very reactive ester of BTX A, but of unknown structure, is rapidly hydrolyzed to BTX A and seems likely the source of the substantial BTX A detected in all Phyllobates extracts. A collection of 2 had 4β-hydroxy-BTX and 4β-hydroxy-hBTX.20 The former is 200 times less active than BTX. Other congeners deserving structural and pharmacological study might arise from application of the more sensitive LC-UV-MS instrumentation now available.
Challenging biological and ecological questions also remain: Only two additional species of the Phyllobates genus, P. lugubris and P. vittatus of Costa Rica and Panama, are known. These were investigated by John for BTXs and other alkaloids: One Panamanian P. lugubris population is only 200 miles away from the Colombian 1-3, yet both species have only traces of BTXs.2 This suggests a dietary source is deficient at these collection sites or these frogs are inefficient in sequestration of alkaloids. The BTXs however do provide a chemical taxonomic marker for the monophyletic genus Phyllobates. Feeding experiments indicated captive-raised 3 are capable of incorporating BTX A into their skins but no conversion to BTX or hBTX was observed.21
The function of the BTXs in frog skin is likely to protect the frog from predators such as snakes.9 Despite the presence of these potent toxins in skins, Phyllobates frogs in captivity seem no less susceptible to diseases as other dendrobatid species (JWD notebook). It was shown that the Na+- channels of muscle or nerve preparations from Phyllobates frogs either wild-caught or captive-raised are not affected by BTX.20 Incidentally, live frogs of species 2 when put together in a bag with non-dendrobatid frogs, caused their deaths. In some of the earliest indications of what came to be known as the “dietary hypothesis”, captive raised Phyllobates frogs slowly lost toxicity (even though species 2 kept for 6 years by Myers was still as toxic as wild-caught 1 or 3) and the F1 generation of 2 was devoid of toxicity. This was remarked on but not understood until the 1990s when the link between a diet of arthropods and the sequestration of their alkaloids into frog skin glands was formulated after painstaking feeding experiments carried out under John’s direction at NIH and Panama.21
BATRACHOTOXINS IN NEW GUINEAN BIRDS
The dietary source of the BTXs in frogs is not known although presumptive evidence was obtained from another part of the world: the remarkable occurrence of batrachotoxins in the feathers, skins and internal organs of two passerine bird genera, Pitohui and Ifrita of Papua New Guinea (PNG).23,24
These observations of the first truly poison birds were the result of an unusually fortuitous collaboration. In 1991, a biology graduate student, Jack Dumbacher, sent feathers and skins to John of specimens of PNG birds of the genus Pitohui that he had observed caused stinging of his lips when contacting bird-caused pecks he experienced from them in the field. John prepared ethanol extracts and injected mice, noting immediately the signs of BTX-like toxicity: locomotor difficulties, partial paralysis, convulsions then death (15-20 min). GC-MS of an alkaloid fraction of the extract indicated no volatile alkaloids, however, direct probe EIMS (Noel Whittaker)23 demonstrated the presence of hBTX. TLC with a reference sample and Ehrlich-reagent detection confirmed the presence of hBTX. Jack had picked, via C. Myers, the one lab in the world where expertise and reference samples allowed the toxin’s identity to be proved within a few days.
Later, feathers, skins and organs of a totally unrelated PNG bird, genus Ifrita, also provided by Jack, was discovered in our lab to harbor BTX, hBTX, BTX A and cis and trans crotonates of BTX A. In this instance, anecdotal accounts by locals of another “rubbish” bird led us to investigate Ifrita extracts by LC-MS and again, having the reference samples was crucial. The presence of BTXs in birds was variable, suggesting a dietary link, consequently a variety of berries and small insects were investigated but without success. Researching more local lore where a tiny beetle (Nanisani, generic in PNG for “bad”) was known to cause stinging of the eyes of workmen during the gathering of thatch, Jack collected specimens of the culprits with the help of locals and four species of a small beetle were indeed found to have BTX by LC-MS and were quickly identified by Allan Samuelson as belonging to the melyrid family.25 These beetles are known prey items for the Pitohui and presumably for the Ifrita also. They are likely the source of the BTXs in birds (~ 1 µg per bird) and perhaps also in the Phyllobates frogs 9,400 miles away (~ 1 mg per frog 2), since this beetle family is wide spread. However, finding BTXs in some beetle specimens and not in others raises the question as to whether there is not a presently unknown original source, as perhaps a symbiont or mite. The beetles contain mainly BTX while the birds have mainly hBTX, which is also perplexing; consequently this particular dietary link, is tenuous. It is not known at present whether the Na+-channels of the birds are insensitive to BTXs.
OTHER CONSIDERATIONS
A remarkable observation is the occurrence of plant alkaloids, chimonanthine and calycanthine with the BTXs in skins of frog species 2.20 The occurrence of plant alkaloids in skins of frogs is virtually unknown. The occurrence of chimonanthine/calycanthine in 2 and 3 and having an opposite chirality from those reported in plants is baffling, although the synthesis of these cyclized N-methyltryptamines by some Phyllobates frogs seems a possibility. Feeding experiments indicated captive raised 3 are capable of incorporating BTX A into their skins but no conversion to BTX or hBTX was observed.22 These experiments provided early evidence that BTXs are not synthesized by Phyllobates frogs but sequestered from diet.
Figure 2 depicts batrachotoxinin-A-O-p-bromobenzoate. The original stereodiagram17 arbitrarily depicted the wrong enantiomer and led to a mistake in the absolute configuration of C-20. The planar representation was drawn17 as a hybrid structure with the steroid chirality correct since it was chosen to agree with the pregnane system; however, a Fischer projection indicated C-20 as S based on the original stereodiagram. The correct absolute stereochemistry, including C-20-R, was provided by Richard Gilardi in 197019 who used the Karle’s atom coordinates and classical anomalous dispersion of X-radiation by the bromine atom. The C-20-S error persisted in the chemical literature (despite a correct Fischer projection for C-20 by Gilardi and an unambiguous stereodepiction of BTX A by I. Karle in 197226) until the C-20-R configuration was confirmed by the synthesis of Yoshito Kishi in 1998.28 Thus for some 30 years, and in many reviews, the 20α designation was used to refer to the C-20 configuration; it should have been 20β, despite the C-20α alcohol configuration occurring in most natural pregnane-3,20-diols. In the energy minimized (CS Chem3D Pro, version 5.0) structure above, hydrogens are omitted, chiralities are indicated, the structure is numbered with conventional steroid nomenclature and the 3D effect is represented by showing smaller atoms to the back.
SAMPLES AND RECORDS
The discovery of BTX in the 1960’s was possible only after arduous expeditions and the sacrifice of over ten thousand frogs, which fortunately occurred in a high-population density. A crystalline derivative for X-ray determination was the means to obtaining the structure. The accumulation of 180 mg of BTX and of 100 mg of hBTX was the result. A radio-labeled BTX-A-O-benzoate as a widely used research tool in neurophysiology was another consequence. Since the cost of synthetic BTX is prohibitive, John until recently, provided investigators around the world with research samples of BTX. Now NIH has shipped the rest of the remaining BTX (170 mg) to a company (Latoxan Valence, France) to distribute.
Other frog species provided John with other alkaloids and what started as the “kokoi venom” project became an alkaloid program, where the isolation, identification and sometimes synthesis of these alkaloids, proceeded in tandem with pharmacology studies in search of possible biological targets for the alkaloids as well as their functions in the frogs. More recently, due to the discovery that most of the alkaloids are sequestered by frogs from an arthropod diet, efforts were also directed to the study of ants, beetles, millipedes and mites.
Over the last 25 years John explored many places and collected many species of poison frogs, but almost always from 1 to 5 specimens per collection site, preparing many skin extracts and creating a large library of small-amount extracts, usually with the same volume of methanol to wet skin weight.
John was very careful in documenting and preserving his samples. In keeping with the best practices of the empirical sciences, he understood the importance and the sanctity of the sample as well as its history. As far as he could, he kept detailed records of his samples (species, amount, location, date), and of the data obtained with them (chemical, biological, spectroscopic). Taxonomic voucher specimens of the frogs are preserved in the American Museum of Natural History.
The data and the samples that remain with us constitute John’s library for the future. And this library is a treasure chest, the result of a tremendous investment in institute resources and his efforts. The reduction of the rain forest has led to the accelerated disappearance of many species of frogs, consequently John’s samples are often unique and truly irreplaceable. According to John, the original collecting sites were in some cases so transformed that was difficult or impossible to find those frogs again and the profile of alkaloids in the few frogs that remained was completely different. What are we going to do with this resource in John’s absence? How can we continue to draw from these irreplaceable extracts?
Clearly a lot of possibilities for success are available with John’s samples and records together. As can be seen in the accompanying paper on epibatidine,29 a whole new chapter in research of nicotinic receptors and analgesia was launched with the discovery of epibatidine, but it took almost eighteen tortuous, patient years to isolate enough for analysis, mainly waiting for the right technology.
In many cases, the samples available now are extracts from a few frogs only, containing dozens of alkaloids in perhaps µg amounts. Interestingly, most major and minor alkaloids in those extracts are now known, through the efforts of John and his group. There are still many trace components of unknown structure and function, with only limited data available. However, even for the most abundant alkaloids, there is a plethora of activities and targets remaining to be investigated. Perhaps not long from now there will be a technology that can test the skin extracts for many targets simultaneously using only a tiny drop of material, such as currently done with microarray technology. And then, perhaps, there will be also a technology that can identify the trace components responsible for a biological activity. So many secrets lie hidden in these samples!
Our responsibility now is to guard the samples and records, as would a museum, for their historical value, but also for their scientific value. They represent an uninvestigated treasure of immense potential, waiting for the loving hand, the right technology and the carefully designed experiment to reveal its secrets, under the premise that perhaps, these samples are all that there ever will be.
ACKNOWLEDGEMENTS
We thank Takashi Tokuyama, now retired, of the Chemistry Dept., Osaka City University for his many valuable insights and comments on this epic structural work in natural products, in which he was the “most valuable player”. We also thank Charles W. Myers, Curator Emeritus (Dept. of Herpetology) of the American Museum of Natural History, New York City, for his critical reading of this manuscript and his numerous corrections and suggestions.
References
1. F. Märki and B. Witkop, Experientia, 1963, 19, 329. CrossRef
2. J. W. Daly and T. F. Spande, ‘Alkaloids: Chemical and Biological Perspectives: Amphibian Alkaloids: Chemistry, Pharmacology and Biology’, vol. 4, ed. by S. W. Pelletier, Wiley, NY, 1986, , Chapter 1, pp. 1-274.
3. J. W. Daly, H. M. Garraffo, and T. F. Spande, ‘Alkaloids: Chemical and Biological Perspectives: Alkaloids from Amphibian Skin’, vol. 13, ed. by S. W. Pelletier, Pergamon, NY, 1999, , Chapter 1, pp. 1-161.
4. B. Witkop, J. W. Daly, J. W. Karle, T. Tokuyama, E. X. Albuquerque, and H. Shimazu, Revista de la Facultad de Farmacia, Universidad de los Andes, 1970, 7, 51.
5. J. W. Daly and B. Witkop, Aldrichcimia Acta, 1970, 3, 3.
6. J. W. Daly and B. Witkop, ‘Chemistry and Pharmacology of Frog Venoms, Venomous Animals’, vol. II, ed. by W. Büchert and E. E. Buckley, 1971, , chapter 39, pp. 497-519.
7. E. X. Albuquerque, J. W. Daly, and B. Witkop, Science, 1971, 172, 995. CrossRef
8. J. Daly and B. Witkop, Clinical Toxicology, 1971, 4, 331.
9. C. W. Myers, J. W. Daly, and B. Malkin, ‘A dangerously toxic new frog (Phyllobates) used by Embera Indians of western Colombia with discussion of blowgun fabrication and dart poisoning’, Bull. Am. Mus. Nat. Hist., 1978, 161, pp. 307-366.
10. J. W. Daly, ‘Progress in the Chemistry of Organic Natural Products: Alkaloids of Neotropical Poison Frogs (Dendrobatidae)’, vol. 41, ed. by W. Herz, H. Grisebach, G. W. Kirby, Springer-Verlag, NY, 1982, pp. 205-340.
11. B. Witkop and E. Gössinger, The Alkaloids, vol. 21, ed. by A. Brossi, Acad. Press, NY, 1983, , pp. 139-253.
12. J. W. Daly, H. M. Garraffo, and T. F. Spande, ‘The Alkaloids: Amphibian Alkaloids’, vol. 43, ed. by G. A. Cordell, Acad. Press, San Diego, 1993, , chap. 3, pp. 185-288.
13. E. X. Albuquerque and J. W. Daly, ‘The Specificity and Action of Animal, Bacterial and Plant Toxins, Receptors and Recognition, Series B, Batrachotoxin, a selective probe for channels modulating sodium conductances in electrogenic membranes’, vol. 1, Chapman and Hall, London, 1976, , pp. 296-338.
14. K. Biemann, ‘Ann. Rev. Pure Appl. Chem.: High Resolution Mass Spectrometry of Natural Products’, vol. 9, 1964, , pp. 95-118.
15. J. Daly, B. Witkop, P. Bommer, and K. Biemann, J. Am. Chem. Soc., 1965, 87, 124. CrossRef
16. T. Tokuyama, J. Daly, and B. Witkop, J. Am. Chem. Soc., 1969, 91, 3931. CrossRef
17. T. Tokuyama, J. Daly, B. Witkop, I. L. Karle, and J. Karle, J. Am. Chem. Soc., 1968, 90, 1917. CrossRef
18. I. L. Karle and J. Karle, Acta Cryst., 1969, B25, 428. CrossRef
19. R. D. Gilardi, Acta Cryst., 1970, B26, 440. CrossRef
20. T. Tokuyama and J. Daly, Tetrahedron, 1983, 39, 41. CrossRef
21. J. W. Daly, C. W. Myers, J. E. Warnick, and E. X. Albuquerque, Science, 1980, 208, 1383. CrossRef
22. J. W. Daly, S. I. Secunda, H. M. Garraffo, T. F. Spande, A. Wisnieski, and J. F. Cover, Jr., Toxicon, 1994, 32, 657. CrossRef
23. J. P. Dumbacher, B. M. Beehler, T. F. Spande, H. M. Garraffo, and J. W. Daly, Science, 1992, 258, 779. CrossRef
24. J. P. Dumbacher, T. F. Spande, H. M. Garraffo, and J. W. Daly, Proc. Nat. Acad. USA, 2000, 97, 12970. CrossRef
25. J. P Dumbacher, A. Wako, S. C. Derrickson, A. Samuelson, T. F. Spande, and J. W. Daly, Proc. Nat. Acad. USA, 2004, 101, 15857. CrossRef
26. I. Karle, Proc. Nat. Acad. USA, 1972, 69, 2932. CrossRef
27. R. A. Saporito, M. A. Donnelly, H. M. Garraffo, T. F. Spande, and J. W. Daly, J. Chem. Ecol., 2006, 32, 795. CrossRef
28. M. Kurosu, L. R. Marcin, T. J. Grinsteiner, and Y. Kishi, J. Am. Chem. Soc., 1998, 120, 6627. CrossRef
29. H. M. Garraffo, T. F. Spande, and M. Williams, Heterocycles, 2009, 79, 207 CrossRef