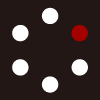
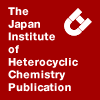
HETEROCYCLES
An International Journal for Reviews and Communications in Heterocyclic ChemistryWeb Edition ISSN: 1881-0942
Published online by The Japan Institute of Heterocyclic Chemistry
e-Journal
Full Text HTML
Received, 27th September, 2008, Accepted, 19th November, 2008, Published online, 27th November, 2008.
DOI: 10.3987/COM-08-S(D)60
■ Hydrophilic Diazirine Polymer for One-Step Photo-Fabrication of Proteins on Polypropylene Surface
Takenori Tomohiro, Norie Tachi, Yusuke Azuma, and Yasumaru Hatanaka*
Faculty of Pharmaceutical Sciences, Toyama University, 2630 Sugitani, Toyama, Toyama 930-0194, Japan
Abstract
A facile photochemical fabrication of chemical and biological functionalities on polypropylene surface has been performed by using photoactivatable polymers containing diazirine photophore. The water-soluble polymers have been synthesized from polyamine or polycarboxylic acid by coupling with aryldiazirine derivatives. Upon irradiation, the photoactivatable polymer successfully immobilized proteins such as horseradish peroxidase, lysozyme, and pepsin on the inert surface of polypropylene film in one step. This soft polymer cushion supplied a hydrophilic environment around the enzyme and enhanced the enzyme activity. Specifically, the polymer (HDA-PEI) introduced a hydrophilic spacer between diazirine and polymer main chain significantly increased the activity.INTRODUCTION
The creation of chemical and biological functionalities on polymer surface is a subject of great interest because most polymers have intrinsically low surface tension. It requires to altering chemical natures of the surface such as wettability, biocompatibility, and chemical reactivity corresponding to the purpose of interest. A number of techniques have been developed for patterning of organic substances on polymer surface such as plasma treatment, graft polymerization,1 photolithography,2 and microcontact printing.3 These methods can directly deposit biomolecules such as proteins onto the surface covalently or noncovalently, but usually need prior chemical activation of the inert polymer surface. In addition, most patterning techniques require the masking and the desorption processes of non-substance-addressed area before/after fabrication of substances. Once the polymer surface is activated, however, it nonspecifically adsorbs substantive amount of biomolecules that resulting in decreasing detection sensitivity due to the background noise. Because the surface cannot be cleaned in strong washing condition, the nonspecific adsorption is especially a serious disadvantage for the high-sensitive detection methods using noncovalent attachments of proteins on the surface. It should be better to retain the low surface tension outside of area for fabrication, which should be resistant to nonspecific protein adsorption. Covalent photoimmobilization, another fabrication technique, of target molecules on the polymer surface is generally achieved by following two different methods, 1) the target molecules are photocross-linked after chemical immobilization by photoactivatable compounds on the activated surface, and 2) direct irradiation on the inert surface after deposition of the target bearing a photoactivatable moiety. Photochemical method using photoactivatable compounds has an advantage since these functional groups can be intrinsically activated by UV irradiation. Therefore, fabrication on the surface is limited to the irradiated area. Photophores such as benzophenone, arylazide,4 and aryldiazirine5,6 that produce high reactive species, radical, nitrene, or carbene, respectively, and which were often used for cross-linking of substances to the polymer surface.
For the analysis of biomolecular interactions, it is also required for controlling the distribution of substances and the spatial environment (hydrophobicity, hydrophilicity, ionic strength, and pH) around the biomolecules to represent precisely these interactions in vivo. Hydrogels such as polyacrylamide,7 polysaccharide, gelatin8 covalently bounded to the surface gave 3D-biocompatible interface on the polymer substrates. By arraying of polyacrylamide gel on the glass surface of microchip, sequencing by hybridization to synthesized oligonucleotides under a liquid phase environment has become possible.9 However, a prior surface treatment process such attachment of functional groups on the glass surface is unavoidable and the gel on the surface is sometimes easily damaged by mechanical shock. Here we report a simple photolithographic approach for the creation of chemical and biochemical functionalities on the polypropylene substrate by employing photoactivatable hydrophilic polymers. Compared to benzophenone and arylazide, the photolysis of aryldiazirine group used in this report rapidly proceeds by UVA irradiation and makes a stable bound between spatially proximate molecules. These polymers have multi-diazirine units in a single molecule that makes covalent bonds to the target molecules as well as the polymer surface at the same time by irradiation.
RESULTS AND DISCUSSION
Immobilization using photoactivatable polymer
The synthesis of photoactivatable polymer (DA-polymers) has been performed by a coupling reaction of polymer and diazirine derivatives. The polymer substrates used in this report basically contain amino or carboxyl groups as functional groups: polyethyleneimine (PEI), poly(allylamine hydrochloride) (PAH), poly(L-Lysine hydrobromide) (PLH), and polyacrylic acid (PAA). Diazirine compounds 1 and 2 were used for the reaction of polyamines, and compound 3 was used for the reaction of polycarboxylic acid, respectively (Figure 1). Compound 1 was prepared according to the previous report.10 Epoxide derivative 2 was derived from compound 1 using trimethylsulfonium iodide,11 and sulfonamide derivative 3 was prepared by reaction of 3-phenyl-3-trifluoromethyl-3H-diazirine and chlorosulfonic acid followed by the treatment with ammonia gas. Incorporation of diazirine photophore into polymer was performed by use of equivalent molar of diazirine derivative against the corresponding reactive groups in polymer such as primary amino group of PEI and carboxyl group of PAA. After purification by gel filtration, the yields of diazirine-incorporation on polyamine were generally 6-39% against the number of reactive groups in polymer, which calculated by measuring of the absorptions at 360 nm and the elemental analysis. The highest yield of incorporation was given as 75% of a photoactivatable polyethyleneimine (DA1-PEI) by the reaction of aldehyde derivative 1 and PEI. Another derivative of polyethyleneimine (DA2-PEI) was obtained by the reaction of epoxide derivative 2 and PEI in 39% yield of diazirine modification. Similar method using compound 2 was applied for PAH and PLH to give corresponding photoactivatable polymers in 6, 20%, respectively. In addition, the polymer containing a hydrophilic spacer (HDA-PEI) was also synthesized by a reaction of PEI and compound 7 that was derived from 3-(3-hydroxyphenyl)-3-trifluoromethyl-3H-diazirine (4) as described in Scheme 1.
For further experiments were used DA1-PEI and DA2-PEI from the aspect of the cost of polymer and the incorporation yield of diazirine. We also prepared other photoactivatable polyamine derivatives by using 3-(4-bromomethylphenyl)-3-trifluoromethyl-3H-diazirine, the incorporation of diazirine was relatively low for three kinds of polyamines (3-14%). Other type of polymer, photoactivatable polycarboxylic acid (DA-PAA), was synthesized by a coupling reaction of compound 3 and PAA, containing N-acylsulfonamide groups. The pKa value of general N-acylsulfonamide is close to that of general carboxylic acid, which results in the formation of an anion under the physiological conditions. Whereas, another photoactivatable polycarboxylic acid synthesized by using compound 6 easily aggregated in an aqueous solution because of increasing the hydrophobicity due to diazirine moiety although it has a hydrophilic spacer resulting the low yield of diazirine incorporation (7%).
Upon irradiation of 360 nm light (15 W x 2), diazirine group produces a carbene, a high-reactive electrophilic species, and immediately reacts with spatially proximate chemical bond. Therefore, diazirine derivatives has been recognized to satisfy most of the chemical criteria required for photoaffinity labeling12 and polymer surface fabrication.5,13 The photolysis was carried out after DA-PEI or original PEI were spotted on the film following the dryness in vacuo. There was nothing left on the surface after washing in both cases without irradiation and with irradiation of original PEI. The yield of immobilization of DA-PEI was determined by measuring the amount of primary amino group after the treatment of ninhydrin reagents. The yield was depended on the concentration of deposited polymer as well as the irradiation time as shown in Figure 2, and the photolysis was completed within 40 min. By the photolysis using 1% w/v of polymer solution, the quantity was reached to ca. 9 x 104 / nm2 of amino groups immobilized on the surface. Although the cross-linking yield of diazirine is usually low since carbene species can react with water, the value is about 104-fold larger than that of commercial amine-modified chips.
Protein fabrication and the activity
This methodology was then applied for immobilization of proteins including horseradish peroxidase (HRP),14 lysozyme, and pepsin by using DA1-PEI for the former two proteins and DA-PAA for the latter one, respectively. These polymers were selected according to the stable pH region of proteins. After incubation of DA1-PEI and HRP following dryness of the film, immobilization of proteins was performed by UV irradiation on ice. Figure 3 shows the quantity of HRP immobilized on the film, which is estimated by the amount of the heme moiety extracted in organic solution according to the previous method.15 While there are small amount of products detected by the treatment from a non-irradiated spot, the amount of immobilized HRP was obviously increased with the irradiation time and then became constant after 60 minutes. It also depended on the amount of DA1-PEI and was maximized in the solution at the molar ratio of protein/polymer as 1:4 (ca. 70% of the deposited amount, Figure 3). The following photolysis in this report has been performed in a solution containing 4 equivalent moles of the photoactivatable polymer by 60-minute irradiation. The activity of immobilized HRP on a polypropylene film was determined by the method of Trinder.16 The optimum activity of immobilized HRP was obtained at pH 9 while native HRP showed it at pH 7 (data not shown). The immobilized HRP remained its activity in 40% of initial value after keeping in a buffer (0.1 M Tris-HCl, pH 9) at 4°C for a week. In addition, the stability of HRP against organic solvents was tested in 20% (v/v) of aqueous organic solvents including MeCN, acetone, and ethanol (Figure 4). The immobilized HRP exhibited rather strong resistance against such organic solvents than non-immobilized HRP, and kept more than 80% of activity in aqueous solution. This is a similar phenomenon to that of a previous report.17 However, the activity was decreased by the enzymatic reaction during repeated measurements. The immobilized HRP should be damaged by hydrogen peroxide used in the reaction. Furthermore, the relative enzyme activity of immobilized HRP against native HRP (non-immobilized HRP) was determined from the pseudo-first-order reaction rate constants in these initial reactions. It was calculated as ca. 0.1% compared to that of native HRP. It is because the reaction surface is quite small on the polymer while native HRP was homogeneously dissolved in a solution. In addition, 4-aminoantipyrine, a substrate for the enzyme reaction, may slowly diffuse into the polymer. In case using DA2-PEI instead of DA1-PEI, the immobilized HRP showed higher relative activity as ca. 2%. It might be due to the increasing of hydrophilic space produced by hydroxyl group in DA2-PEI polymer. To increase the swelling of the polymer on the film and to gain the space for the interaction, the hydrophilic spacer was added between the photophore and polymer main chain. The yield of HDA-PEI immobilization on a polypropylene film was smaller than that of DA1-PEI (Figure 5), but the relative activity of HRP immobilized with HDA-PEI increased 6-fold in comparison with that of DA-PEI (black columns in Figure 6). We also evaluated the activity by luminol emission catalyzed by HRP. This method requests a small quantity of reagents because of the high sensitive detection as well as the simple treatment. It gave similar level of the enzyme activity to that of native HRP as 75 and 98% for immobilized HRP using DA-PEI and HDA-PEI, respectively. However, the enzyme activity of HRP for oxidation is basically depended on the activity of the heme moiety. It may not be an appropriate example for evaluation of the protein fabrication. Therefore, we then applied this method for immobilization of lysozyme that catalyzes hydrolysis of beta (1-4) glycosidic bond of N-acetylmuramic acid and N-acetylglucosamine. The activity of immobilized lysozyme was evaluated by the previous method using Micrococcus lysodeikticus.18 The relative activities of immobilized lysozyme were 0.1 and 2.8% for DA-PEI and HDA-PEI, respectively (white columns in Figure 6). HDA-PEI was significantly enhanced the enzymatic reaction of lysozyme at 28-fold higher reaction rate than DA-PEI as a similar result with the case of HRP. It might be due to the larger hydrophilic space around lysozyme or increasing of the stability. Moreover, pepsin was also treated in a similar method by using DA-PAA. While pepsin was not efficiently immobilized on the film, the immobilized pepsin exhibited its activity.
CONCLUSION
The soft polymer cushion on the organic and inorganic substrate has been an important process for fabrication of DNA chip,19 biological system on membrane.20 The present photolithographic method using photoactivatable hydrophilic polymer illustrated a very simple fabrication of polymer cushion as a basis matrix as well as one-step fabrication of biological functionalities on the surface of inert polymer. It also gained higher S/N value for the analysis because the variety of functions was fabricated only within deposited area on the surface. The specific activity of fabricated enzymes increased by the introduction of a hydrophilic spacer between a photophore and polymer main chain. The flexibility and hydrophilicity of spacers could provide more spaces within a polymer to enhance the diffusion rate of the substances. This technique will contribute to control immobilization of proteins on polymer surface that is important implications in many areas of science and technology.
EXPERIMENTAL
Melting points were measured on Yanaco MP-S3 micro melting point apparatus and are uncorrected. 1H NMR spectra were recorded at 500 MHz on Varian UNITY plus 500 spectrometer. IR spectra were recorded on JASCO FT/IR-460plus. UV-Vis absorption spectra were measured on JASCO V-550. MS spectra were obtained by using a JEOL JMS-GCMATE. Chemiluminescence detection was performed by ChemiDoc system (Bio-Rad Lab. Inc.). Polyethyleneimine (PEI, molecular weight: 10,000), poly(L-Lysine hydrobromide) (PLH, molecular weight: 5,000 - 15,000), and polyacrylic acid (PAA, molecular weight: 25,000), pepsin were purchased from Wako Pure Chemical Industries, Ltd. Poly(allylamine hydrochloride) (PAH, molecular weight: 15,000) and horseradish peroxidase (HRP) were purchased from Sigma-Aldrich Corp. Polypropylene (PP) film was obtained from Shin-kobe Electric Machinery Co., Ltd. Streptavidin-HRP was obtained from GE Healthcare UK Ltd. All chemicals were of analytical grade and were used without further purification.
3-(4-Oxiranylphenyl)-3-trifluoromethyl-3H-diazirine (2): Trimethylsulfonium iodide (0.95 g, 4.67 mmol), potassium hydroxide (0.52 g, 9.34 mmol) was stirred in MeCN (4 mL) at 60 °C under nitrogen for 10 min. To the suspension was added a MeCN solution (5 mL) of 4-[3-(trifluoromethyl)-3H-diazirin-3-yl]benzaldehyde21 (1, 1.0 g, 4.67 mmol) and water (20 mL). The reaction mixture was stirred for 1 h and poured into cold Et2O. The organic phase was washed with water, brine, and dried over MgSO4. After removal of solvent, the residue was purified by chromatography (hexane:CHCl3 = 1:2) on silica gel to afford compound 3 as a pale yellow oil (0.56 g, 52%). 1H NMR (CDCl3): δ 7.31 (d, J = 8.3 Hz, 2H), 7.18 (d, J = 7.9 Hz, 2H), 3.81 (dd, J = 2.6, 3.8 Hz, 1H), 3.17 (dd, J = 5.6, 3.8 Hz, 1H), 2.75 ppm (dd, J = 2.6, 5.6 Hz, 1H); IR (liquid film): ν 1343, 1233, 1156 cm-1; UV-vis (hexane) λmax = 228 (ε 15700), 268 (ε 490), 342 (sh, ε 290), 357 (ε 390), 371 nm (ε 290); MS (m/z, %): 229 (M+, 10), 201 (100).
3-(4-Aminosulfonylphenyl)-3-trifluoromethyl-3H-diazirine (3): Chlorosulfonic acid (23.3 g, 214 mmol, caution: a hygroscopic and a powerful lachrymator) was slowly added into 3-phenyl-3-trifluoromethyl-3H-diazirine (8.0 g, 43.0 mmol) placed in a 100mL flask followed by stirring at rt for 30 min using a CaCl2 drying tube. The mixture was carefully poured on ice and the product was extracted with Et2O. Ammonia gas was passed through the ethereal solution until the solution become basic, and nitrogen was then bubbled to remove excess ammonia. After neutralization with conc. HCl, the reaction mixture was washed with water, brine, and dried over MgSO4. After removal of solvent, the residue was purified by chromatography (hexane:EtOAc = 2:1) on silica gel to afford compound 1 as a white solid. The product was recrystallized from EtOAc to give a white needle (8.6 g, 76%), mp 133-134.5 °C. 1H NMR (500 MHz, CDCl3): δ 7.97 (d, J = 8.4 Hz, 2H), 7.34 (d, J = 8.4 Hz, 2H), 4.84 ppm (s, 2H); IR (KBr): ν 3388, 3257, 3102, 1331 cm-1; UV-vis (CHCl3) λmax = 241 (ε 10000), 272 (ε 850), 280 (ε 760), 344 (ε 355), 357 nm (sh, ε 280); MS (m/z, %): 266 (M+, 20), 237 (100).
3-[2-[2-(2-tert-Butoxycarbonylaminoethoxy)ethoxy]ethoxyphenyl]-3-trifluoromethyl-3H-diazirine (5): To a solution of 3-(3-hydroxyphenyl)-3-trifluoromethyl-3H-diazirine 4 (1.12 g, 4.85 mmol), K2CO3 (0.56 g, 4.00 mmol) in DMF (5 mL) was added 2-[2-(tert-butoxycarbonylaminoethoxy)ethoxy]ethyl bromide (1.38 g, 4.00 mmol). The mixture was stirred at 60 °C overnight under argon. The solvent was removed in vacuo. The mixture was extracted with EtOAc three times, and the combined organic phase was then washed with brine and dried over MgSO4. After removal of the solvent, the residue was purified by chromatography (hexane:Et2O = 1:4) on silica gel to afford compound 1 as a yellow liquid (0.88 g, 48%). 1H NMR (CDCl3): δ 7.30 (t, J = 9.0 Hz, 1H), 6.96 (dd, J = 2.6, 9.0 Hz, 1H), 6.77 (d, J = 9.0 Hz, 2H), 6.72 (s, 1H), 4.99 (br s, 1H), 4.13 (t, J = 5.4 Hz, 2H), 3.84 (t, J = 5.4 Hz, 2H), 3.63-3.73 (m, 4H), 3.55 (t, J = 5.7 Hz, 2H), 3.28-3.36 (m, 2H), 1.43 ppm (s, 9H); IR (liquid film): ν 3359, 1712 cm-1; UV-vis (CHCl3) λmax = 240 (ε 1490), 279 (ε 1910), 355 (ε 320), 369 (sh, ε 270); MS (m/z, %): 434 (M+, 50), 334 (100).
3-[2-[2-(2-Bromoacethylaminoethoxy)ethoxy]ethoxyphenyl]-3-trifluoromethyl-3-H-diazirine (7): The compound 5 (0.43 g, 1.0 mmol) was treated with 50% TFA-CH2Cl2 (1 mL) at rt for 1 h. After evaporated the solvent, the residue was dissolved in CH2Cl2 (1 mL) and then adjusted pH to ca. 9 by addition of Et3N. α-Bromoacetyl bromide (200 μL, 2.3 mmol) was added to the mixture followed by stirring at rt for 2 h. The mixture was washed with water and dried over MgSO4. After removal of the solvent, the residue was purified by chromatography (CH2Cl2:EtOAc = 2:1) on silica gel to afford compound 1 as a yellow liquid (74 mg, 16%). 1H NMR (CDCl3): δ 7.30 (t, J = 9.0 Hz, 1H), 6.97 (dd, J = 2.9, 9.0 Hz, 1H), 6.91 (br s, 1H), 6.78 (d, J = 9.0 Hz, 1H), 6.72 (s, 1H), 4.14 (t, J = 4.8 Hz, 2H), 3.87 (t, J = 4.8 Hz, 2H), 3.86 (s, 2H), 3.71-3.74 (m, 2H), 3.66-3.69 (m, 2H), 3.60 (t, J = 5.2 Hz, 2H), 3.50 ppm (q, J = 5.4 Hz, 2H); IR (liquid film): ν 3301, 1666 cm-1; UV-vis (CHCl3) λmax = 240 (ε 1720), 279 (ε 1830), 355 (ε 360), 369 (sh, ε 300); MS (m/z, %): 453 (M+, 8), 455 (M+, 8), 346 (50), 157 (100).
Synthesis of photoactivatable polyethyleneimine using compound 1 (DA1-PEI): To a solution of PEI (6.0 g) in acetic acid (125 mL) was added a solution of 4-[3-(trifluoromethyl)-3H-diazirin-3-yl]benzaldehyde 1 (6.2 g, 30.0 mmol) in MeOH (50 mL), and the reaction mixture was stirred at 50 °C for 24 h. Excess amount of sodium cyanoborohydride and MeOH (175 mL) was added and the mixture was stirred at 50 °C for 24 h under argon. After removal of the solvent, the residue was dissolved in water (300 mL) followed by washing with CHCl3. The aqueous solution was reduced to about 80 mL and was then dialyzed (cellulose ester membrane, 1,000 molecular weight cut off). The solvent was removed in vacuo to give a pale yellow solid (6.7 g, 75% yield of diazirine modification).
Synthesis of photoactivatable polymers using compound 2 (DA2-PEI): A solution of 3-(4-oxiranylphenyl)-3-trifluoromethyl-3H-diazirine 2 (30 mg), PEI (17 mg) in CHCl3 (2 mL) was stirred at 50 °C for 24 h under dark. The reaction was monitored by thin-layer chromatography. The solvent was removed under reduced pressure, and the residue was dissolved in water (1 mL). The solution was subjected to gel filtration on Sephadex G15 (Column: inner diameter 0.75 cm, height 36 cm, volume 63.6 cm3) eluted with water. Each fraction was measured the absorption at 360 nm and 254 nm. The polyamine fractions were recovered and freeze-dried to yield 15.8 mg of yellow viscous liquid (DA2-PEI). Yield of diazirine modification on polymer was calculated against number of primary amines in polymer was 39% calculated by the absorption at 360 nm corresponding to 3-phenyl-3-trifluoromethyl-3H-diazirine moiety. Similar method was applied for PAH and PLH to give corresponding photoactivatable polymers in 6, 20%, respectively.
Synthesis of photoactivatable polymers using compound 4 (DA-PAA): A solution of PAA (18.8 mg, 0.26 mmol) and N-methylimidazol (22 mg, 0.26 mmol) in DMF solution (4 mL) was added 3-(4-sulfonamidyl)-3-trifluoromethyl-3H-diazirine 3 (35 mg, 0.13 mmol) and diisopropylcarbodiimide (66 mg, 0.52 mmol) and was stirred at 50 °C for 24 h under dark. The decrease of the compound 3 was monitored by thin-layer chromatography. The solvent was removed under reduced pressure, and the residue was dissolved in water that was washed with CHCl3. After reducing to 1 mL, it was subjected to gel filtration on Sephadex G15 eluted with water. Each fraction was measured the absorption at 360 nm and 254 nm. The polyamine fractions were recovered and freeze-dried to give 15.8 mg of pale yellow viscous liquid. The yield of diazirine modification on polymer was 39%.
Synthesis of photoactivatable polymers using compound 7 (HDA-PEI): The compound 7 (22.7 mg) and diisopropylethylamine (6.5 mg) were added to a DMF solution (2 mL) of PEI (6.5 mg), and the mixture was stirred at rt overnight. The solvent was removed under reduced pressure, and the residue was dissolved in water (1 mL). The solution was subjected to gel filtration on Sephadex G15 eluted with water. Each fraction was measured the absorption at 360 nm and 254 nm. The polymer fractions were recovered and freeze-dried to give pale yellow viscous liquid (20.5 mg, 20% yield of diazirine modification).
Immobilization of polymer on a polypropylene surface: Aqueous solution of photoactivatable polymer (1% w/v, 10 μL per dot) was spotted onto a polypropylene film (thickness 0.2 cm) and dried for 20 min under reduced pressure. After photolysis by UV irradiation at a distance of 4 cm from blacklight lamp (360 nm, 15 W × 2) for 30 min, the film was thoroughly washed with ion-exchanged water, 1 M hydrochloric acid, and was rinsed with pure water. The immobilization of polyamines was estimated by measuring of the amount of primary amine on the film by a ninhydrin reaction. In case of polycarboxylic acid, the film was treated with N-hydroxysuccimide (0.5 M) and DCC (0.5 M) in DMF for 4 h at rt, and was then washed with DMF. The film was incubated in a DMF solution of diethylene glycol bis(3-aminopropyl) Et2O overnight at rt followed by washing with DMF. The amount of amino groups was estimated by ninhydrin reaction.
Immobilization of HRP and lysozyme on a polypropylene film using DA-PEI: A mixed solution of an aqueous solution of DA1-PEI (1% w/v, 5 μL) and an aqueous solution of HRP (1% w/v, 5 μL) was spotted onto a polypropylene film and dried. After irradiation with UV for 30 min, the film was ultrasonicated for 1 min in a Tris-HCl buffer (0.1 M, pH 9) in a plastic bag. The same procedure has been performed for Lysozyme.
Enzyme assay of HRP: Firstly, chemiluminescence of luminol catalyzed by HRP on the film was detected. The amount of HRP immobilized on the film was determined by measuring the amount of heme moiety on the film.14 The film was thoroughly shaken in a cold 0.1 M HCl - 2-butanone (1: 1 v/v) for 30 min, the heme moiety was extracted twice in organic layer, and the amount was calculated from the absorption at 384 nm. The activity of HRP on the film was evaluated by the modified method of the previous report.16 The film was floated and stirred in a reaction solution (3.0 mL) containing 4-aminoantipyrine with phenol (0.625 mM) and hydrogen peroxide (0.85 mM) in a 0.1 M Tris-HCl buffer (pH 9) at 25 °C followed by monitoring the absorption at 510 nm.
Enzyme assay of lysozyme: The activity of immobilized lysozyme was evaluated by the previous method using Micrococcus lysodeikticus.18 The film was floated and stirred in a reaction solution (3.0 mL) containing Micrococcus lysodeikticus (0.87 mg) in a 0.1 M potassium phosphate buffer (pH 7) at 25 °C followed by monitoring the absorption at 410 nm.
Immobilization and enzyme assay of pepsin on a polypropylene film using DA-PAA: A mixed solution of an aqueous solution of DA-PAA (1% w/v, 40 μL) and a 0.01 M HCl solution of pepsin (1% w/v, 5 μL) was spotted onto a polypropylene film and dried. After irradiation with UV for 60 min, the film was ultrasonicated for 1 min in a sodium acetate buffer (0.1 M, pH 3) in a plastic bag. The activity of pepsin on the film was evaluated by the enzyme activity using hemoglobin according to the previous report.22 The film was floated and stirred in a reaction solution at 37 °C followed by monitoring the absorption of peptide fragments dissolved in trichloroacetic acid at 280 nm after filtration.
ACKNOWLEDGEMENTS
This research was financially supported by the Ministry of Education, Culture, Sports, Science and Technology through Special Coordination Funds for Promoting Science and Technology, and also Grants-in-Aid for Scientific Research (18390036 and 20390032), CLUSTER (Cooperative Link of Unique Science and Technology for Economy Revitalization), and the Fugaku Trust for Medicinal Research.
References
1. T. Nakayama and T. Matsuda, Macromolecules, 1996, 29, 8622. CrossRef
2. W. He, C. R. Halberstadt, and K. E. Gonsalves, Biomaterials, 2005, 25, 2055. CrossRef
3. Y. Xia and G. M. Whitesides, Angew. Chem. Int. Ed., 1998, 37, 550. CrossRef
4. M. Hengsakul and A. E. G. Cass, Bioconjugate Chem., 1996, 7, 249. CrossRef
5. Y. Hatanaka, M. Hashimoto, and Y. Kanaoka, J. Am. Chem. Soc., 1998, 120, 453. CrossRef
6. P. Reichmuth, H. Sigrist, M. Badertscher, W. E. Morf, N. F. de Rooij, and E. Pretsch, Bioconjugate Chem., 2002, 13, 90. CrossRef
7. (a) T. Matsuda and T. Sugawara, J. Biomed. Mater. Res., 1995, 29, 749; CrossRef (b) G. Chen, Y. Imanishi, and Y. Ito, Macromolecules, 1998, 31, 4379. CrossRef
8. Y. Ito, H. Hasuda, T. Yamauchi, N. Komatsu, and K. Ikebuchi, Biomaterials, 2004, 25, 2293. CrossRef
9. G. Yershov, V. Barsky, A. Belgovskiy, E. Kirillov, E. Kreindlin, I. Ivanov, S. Parinov, D. Guschin, A. Drobishev, S. Dubiley, and A. Mirzabekov, Proc. Natl. Acad. Sci. USA, 1996, 93, 4913. CrossRef
10. H. Nakashima, M. Hashimoto, Y. Sadakane, T. Tomohiro, and Y. Hatanaka, J. Am. Chem. Soc., 2006, 128, 15092. CrossRef
11. M. Nassal, J. Am. Chem. Soc., 1984, 106, 7540. CrossRef
12. (a) T. Tomohiro, M. Hashimoto, and Y. Hatanaka, Chem. Rec., 2005, 5, 385; CrossRef (b) M. Hashimoto and Y. Hatanaka, Eur. J. Org. Chem., 2008, 2513. CrossRef
13. P. Reichmuth, H. Sigrist, M. Badertscher, W. E. Morf, N. F. de Rooji, and E. Pretsch, Bioconjugate Chem., 2002, 13, 90. CrossRef
14. V. I. Silin, G. A. Balchytis, and J. J.Kulys, J. Biochem. Biophys. Methods, 1993, 26, 71. CrossRef
15. F. W. J. Teale, Biochim. Biophys. Acta, 1959, 35, 543. CrossRef
16. P. Trinder, Ann. Clin. Biochem., 1966, 6, 24.
17. K. F. Fernandes, C. S. Lima, F. M. Lopes, and C. H. Collins, Process Biochem., 2004, 39, 957. CrossRef
18. D. Shugar, Biochim. Biophys. Acta, 1952, 8, 302. CrossRef
19. J. C. Barrett and E. S. Kawasaki, Drug Discov. Today, 2003, 8, 134. CrossRef
20. E. Sackmann and M. Tanaka, Trends Biotech., 2000, 18, 58. CrossRef
21. U. Kempin, Y. Kanaoka, and Y. Hatanaka, Heterocycles, 1998, 49, 465. CrossRef
22. M. Anson, J. Gen. Physiol, 1938, 22, 79. CrossRef