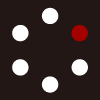
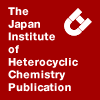
HETEROCYCLES
An International Journal for Reviews and Communications in Heterocyclic ChemistryWeb Edition ISSN: 1881-0942
Published online by The Japan Institute of Heterocyclic Chemistry
e-Journal
Full Text HTML
Received, 2nd October, 2008, Accepted, 1st December, 2008, Published online, 5th December, 2008.
DOI: 10.3987/COM-08-S(D)63
■ Antineoplastic Agents. 575. The Fungus Aspergillus phoenicis
George R. Pettit,* Jiang Du, Robin K. Pettit, John C. Knight, and Dennis L. Doubek
Department Chemistry and Biochemistry, Arizona State University, Physical Sciences bldg. PSD118 1711 S. Rural Rd., Tempe, Arizona 85287, U.S.A.
Abstract
Cancer cell line bioassay-guided separation of an extract from the fungus Aspergillus phoenicis collected in Saskatchewan, Canada, resulted in the isolation of three new constituents designated asperlactone (1), aspergillol A (2), and aspergillol B (3), accompanied by four previously known constituents: asperic acid (4), hexylitaconic acid (5), methyl 2-hydroxyphenylacetate (6), and methyl 4-hydroxyphenylacetate (7). The structure of each was determined by analyses of high-resolution mass spectra and high-field NMR data. Asperic acid (4) was found to inhibit growth of the murine lymphocytic leukemia P388 (ED50 0.18 μg/mL) and a panel of human cancer cell lines (GI50 1.7–2.0 μg/mL; pancreas, breast, CNS, lung, colon, and prostate), while aspergillol A (2) showed moderate inhibition against the breast adenocarcinoma MCF-7 (GI50 7.2 μg/mL).INTRODUCTION
Fortunately, the realization is rapidly gaining momentum that naturally occurring substances,2 especially from microorganisms as well as their recombinant modifications,3 will increasingly allow discovery of ever more useful anticancer, antiinfective, and other drugs for a broad spectrum of medical applications. Illustrative are some recent examples of substances isolated from fungi that include the antiviral anthraquinone hypericin from an endophytic fungus,4a the strong cancer cell growth inhibitory (GI50 0.039 μg, 41 mM/mL) cyclodepsipeptides pteratides 1 and 2 from a Malaysian Basidiomycete,4b and the cancer cell selective (against PTEN-negative cells) peptide, culicinin D, from an entomopathogenic fungus.4c
As one of our initiatives to discover new anticancer substances produced by marine5a and terrestrial5b microorganisms, we continued to evaluate specimens collected (2001) in Saskatchewan, Canada. That led to isolation of a fungus later identified as Aspergillus phoenicis (formerly known as Aspergillus saitoi)6 from a plant collected in September, 2001 near Mechem, Saskatchewan. The plant was found growing in a dry lake whose surface was covered with precipitated salts. Fungi of the genus Aspergillus such as A. flavus are well known producers of the powerful carcinogenic aflatoxins that are natural contaminants of human and animal foods. In general, Aspergillus species produce both very toxic6a and potentially useful7 (as well as valuable, e.g., for food processing) metabolites, such as hydroxyflavanone antioxidants7a,b and enzymes. The latter group includes a variety of important enzymes produced by A. phoenicis such as α-D-mannosidases,8a β-glucosidases,9a-d catalases,10 xylanases,11 aspartic proteinases,12 and a carboxypeptidase used for peptide sequencing.13
An example of some starkly contrasting biological properties of A. phoenicis components was displayed by the recent isolation of α-cyclopiazonic acid, a very potent indole alkaloid carcinogen/neurotoxin (seizures/death),6b and of the antioxidant mixture (FSAP) that in part reduced the liver fibrosis induced by CCl4 in rats.6c Hence, it was clear, as usual, from the outset that cancer cell line (P388 murine leukemia and human cancer cell lines) bioassay-guided isolation would be essential to direct separations. The result was isolation of three new constituents named asperlactone (1), aspergillol A (2), and aspergillol B (3), of which phenol 2 showed moderate cancer cell inhibitory activity. In addition, four previously known compounds were isolated: asperic acid (4), hexylitaconic acid (5), and methyl 2-hydroxy- and 4-hydroxyphenylacetates (6 and 7). Of these, tetrahydrofuran 4 was found to exhibit significant activity against P388 and a panel of human cancer cell lines.
RESULTS AND DISCUSSION
The scale-up production of A. phoenicis by fermentation (4 × 97-L batches) provided 388 L of broth that was extracted with ethyl acetate. The solvent was evaporated and the residue was initially separated by a solvent-partitioning sequence (hexane/9:1 MeOH–H2O → CH2Cl2/3:2 MeOH–H2O), followed by separation of the marginally active (P388 lymphocytic leukemia, ED50 10 μg/mL) CH2Cl2 fraction by a gel permeation → partition chromatographic → HPLC sequence to afford pure 1 (10.6 mg), 2 (5.3 mg), 3 (3.2 mg), 4 (6.5 mg), 5 (2.7 mg), 6 (2.0 mg) and 7 (3.4 mg).
Asperlactone (1) was obtained as a colorless oil, and its molecular formula was determined to be C16H26O4 by HRAPCIMS. The 1H NMR spectrum of 1 showed signals corresponding to five methyl groups at δ 0.86 (3H, d, J = 6.0 Hz), 1.10 (3H, d, J = 7.0 Hz), 1.29 (3H, d, J = 7.0 Hz), 1.60 (3H, s), and 2.14 (3H, s) and one olefinic proton at 5.40 (1H, t, J = 7.0 Hz). By employment of 1H–1H COSY techniques, two proton-relayed spin-spin systems [A: CH-CH2-CH2-CH (Me); B: O-CH-CH (Me)-CH2-CH(Me)-] were revealed. HMBC experiments allowed us to determine the linkages of the spin-spin systems. In system A, the signals corresponding to the methyl protons at δ 1.10 (H-16), the tertiary proton at δ 2.55 (H-10) and the methylene proton at δ 1.38, 1.75 (H-9) showed HMBC cross-peaks with a carbonyl carbon (C-11, δ 212.3). Another methyl proton at δ 2.14 also showed correlations with C-11. Based on its chemical shift and these HMBC correlations, the carbon link from C-7 to C-12 was determined. HMBC correlations were observed from the methyl protons at δ 1.60 (H-15) and the tertiary proton at δ 4.21 (1H, d, J = 10.5 Hz, H-5) to the olefinic carbon (C-7, δ 131.1), confirming a double bond linkage between system A and B. The correlations between the methyl signal at δ 1.29 (H-13) and the methylene at δ 1.40, 1.98 (H-3) to the carbonyl carbon (C-1, δ 174.5) indicated that the other end of system A was linked to a carbonyl carbon (Figure 1).
According to the molecular formula and degrees of unsaturation, the structure of 1 should be a δ lactone. 2D-ROESY experiments afforded a valuable NOE correlation from δ 4.21 (H-5) to δ 5.40 (H-7). That showed that the double bond between C-6 and C-7 should have E geometry. The NOE correlation of δ 0.86 (H-14) and δ 4.21 (H-5) indicated the methyl at C-4 and proton at C-5 to be oriented in a cis relationship. Another NOE correlation between H-2 and H-4 suggested the 2-Me and 4-Me groups to be in a cis configuration also. Both H-4 and H-5 were assigned as axial in a partial chair conformation, based on the coupling constant of H-5 (J = 10.5 Hz). Thus, the overall structure and relative configuration of 1 was assigned as shown in Figure 2.
Aspergillol A (2) was also obtained as an oil (yellow). The molecular formula was found to be C16H16O4 by HRAPCIMS. The 1H spectrum of 2 revealed eight aromatic proton signals at δ 7.10 (1H, m), 7.04 (1H, m), 6.96 (2H, d, J = 7.6 Hz), 6.78 (1H, m), 6.76 (1H, m), and 6.67 (2H, d, J = 7.6 Hz). Three methylene proton signals were observed at δ 4.19 (2H, t, J = 6.8 Hz), 3.57 (2H, s), and 2.76 (2H, t, J = 6.8 Hz) in the 1H spectrum. The 13C APT spectrum showed a signal at δ 174.3 corresponding to a carbonyl carbon, aromatic carbons at δ 156.8, 156.6, 132.1, 131 (2C), 130.2, 129.4, 122.5, 120.5, 116.2 (2C), and 115.9 and three methylene carbons at δ 66.9, 36.8, and 35.1. Based on the 1H and APT spectra, it was concluded that the structure contained two aromatic rings: one o-substituted and the other p-substituted. The 1H-1H COSY and HMBC data indicated a -CH2-CH2-O-CO-CH2- unit. HMBC correlations of H-7 (δ 3.57) to C-6 (122.5), C-1 (156.8), and C-8 (174.3) suggested that H-7 was bonded to the o-substituted aromatic ring. The H-10 signal (2.76) showed HMBC cross-peaks with C-9 (66.9) and C-11 (130.2), while H-12 and H-16 (6.96) showed HMBC correlations to C-11 (130.2), C-10 (35.1), and C-14 (156.6), which confirmed that C-10 was bonded to the p-substituted aromatic ring. From the spectral data analyses, it was clear that aspergillol A (2) corresponded to structure 2.
Aspergillol B (3) was obtained as a yellow oil corresponding to molecular formula C16H16O4, as determined by HREIMS. The 1H NMR spectrum of 3 was very similar to that of 2, except for the coupling constants of the aromatic protons. The 1H NMR spectrum of 3 showed four doublets at δ 6.68, 6.72, 6.96 and 7.02, which confirmed that both aromatic rings of 3 were p-substituted and 3 was simply an isomer of 2.
Further separation (by HPLC) of cancer cell line active fractions led to the isolation of asperic acid (4),14 hexylitaconic acid (5),14 2-hydroxyphenylacetic acid methyl ester (6),15 and 4-hydroxyphenylacetic acid methyl ester (7).16 With each of these previously known compounds, the characterization and final identification was determined by spectroscopic analyses and comparison of physical properties.
Asperic acid (4) exhibited significant cancer cell growth inhibitory activity when evaluated against the murine P388 lymphocytic leukemia cell line (ED50 0.16 μg/ml) and a minipanel of six human tumor cell lines: pancreas adenocarcinoma (BXPC-3 GI50 1.7 μg/mL), CNS glioblastoma (SF-268 GI50 1.9 μg/mL), lung large cell (NCI-H460 GI50 1.9 μg/mL), colon adenocarcinoma (KM 20L2 GI50 1.8 μg/mL), and prostate adenocarcinoma (DU-145 GI50 2.0 μg/mL). Aspergillol A (2) showed moderate inhibitory activity against breast adenocarcinoma (MCF-7 GI50 7.2 μg/mL).
EXPERIMENTAL
Solvents used for column chromatography were distilled. Sephadex LH-20 (particle size 25-100 μm) used for gel permeation and partition column chromatographic separations was obtained from Pharmacia Fine Chemicals AB, Uppsala, Sweden. The TLC plates were viewed under shortwave UV light and then developed by I2 or 3% ceric sulfate in 3N sulfuric acid spray reagent followed by heating at approximately 150 °C. Analytical HPLC was conducted with a Hewlett-Packard Model 1100 HPLC. Semipreparative HPLC was performed with a Waters Delta 600 coupled with a Waters 2487 double λ absorbance detector. High-resolution mass spectra were obtained using a JEOL LCMate magnetic sector instrument by APCI with a polyethylene glycol reference, or using a JEOL GCMate double-focusing mass spectrometer by EI. The 1H and 13C NMR, APT, 1H-1H COSY, HMQC, HMBC, and NOESY spectral data were recorded using a Varian Unity INOVA-500 spectrometer operating at 500 MHz or 400 MHz for 1H NMR as well as for 2D NMR and at 125 MHz or 100 MHz for 13C NMR, all referenced to tetramethylsilane (TMS).
COLLECTION AND FERMENTATION
Plant samples were collected (September, 2001) in clean plastic bags from dry lakes near Mechem, Saskatchewan, and shipped to our laboratory. Plant samples were rinsed in sterile H2O and disinfected in 70% EtOH prior to dilution-plating. Prior to large-scale fermentation (6-L flasks containing 4 L media), the human cancer cell line activity of the fungus was determined to be optimum in half-strength malt extract broth (final concentration: malt extract, 3g/L; maltose, 0.9 g/L; dextrose, 3g/L; yeast extract, 0.6g/L) containing 14g/L Instant Ocean, for 21 days at rt with shaking.
The fungus was identified by large subunit rRNA gene sequencing (Accugenix, Newark, DE). Results from the MicroSeq database indicated that the fungus was Aspergillus phoenicis (% difference=0, confidence level to species). Voucher specimens (ASU-B901528) are available from RKP.
EXTRACTION AND ISOLATION
The culture broth (388 L) was extracted three times with EtOAc. The combined extract was removed from the rotavapor flasks with EtOAc–water and partitioned (× 4) with EtOAc. After evaporation of solvent, the residue was dissolved in 9:1 MeOH–H2O and extracted with hexane. After separation, the MeOH–H2O layer was diluted with H2O to a ratio of 3:2. The resulting aqueous solution was extracted with CH2Cl2. The cancer cell line moderately active (P388 murine lymphocytic leukemia ED50 10 μg/mL) CH2Cl2 fraction (13.42 g) was triturated with MeOH to yield soluble and sparingly soluble phases. Successive Sephadex LH-20 column chromatographic separations employing MeOH → hexane → CH2Cl2–MeOH (5:1:1) followed by separation of active fractions using a C18 column with a linear gradient of H2O–MeCN (95:5 60 min to 50:50) as mobile phase afforded 1 (10.6 mg), 2 (5.3 mg), 3 (3.2 mg), 4 (6.5 mg), 5 (2.7 mg), 6 (2.0 mg) and 7 (3.4 mg).
Asperlactone (1): colorless oil; IR νmax 2965, 2930, 1727, 1713, 1219, 772 cm-1. 1H-NMR (CDCl3, 400 MHz) and 13C-NMR (CDCl3, 100 MHz) assignments have been summarized in Table 1; HRAPCIMS m/z 267.1726 [M+H]+ (calcd 267.1960).
Aspergillol A (2): yellow oil; 1H-NMR (MeOH-d4, 400 MHz) and 13C-NMR (MeOH-d4, 100 MHz) assignments have been recorded in Table 1; HRAPCIMS m/z 273.1140 [M+H]+ (calcd 273.1127).
Aspergillol B (3): yellow oil; for the 1H-NMR (MeOH-d4, 500 MHz) and 13C-NMR (MeOH-d4, 125 MHz) assignments, refer to Table 1; HREIMS m/z 272.1141 [M+H]+ (calcd 272.1048).
ACKNOWLEDGEMENTS
With thanks and appreciation we acknowledge the financial support provided by Grants RO1 CA 90441-01-05, 2R56-CA 09441-06A1, and 5RO1 CA 090441-07 with the Division of Cancer Treatment and Diagnosis, NCI, DHHS; the Arizona Disease Control Research Commission; the Fannie E. Rippel Foundation; Dr. Alec D. Keith; the Robert B. Dalton Endowment Fund; and Dr. William Crisp and Mrs. Anita Crisp. We also are pleased to thank the Government of Saskatchewan, Canada, and its Department of Conservation, Parks and Natural Areas Branch as well as the Forestry Branch. For other helpful assistance, we thank Dr. Jean Charles Chapuis, Felicia Craciunescu, Michael Dodson, M. Jean Pettit, and Christine Weber.
References
1. For Contribution 574 refer to: Z. Zang, J. Du, Y. Xu, D. Liso, and G. R. Pettit, in preparation.
2. D. J. Newman and G. M. Cragg, J. Nat. Prod., 2007, 70, 461. CrossRef
3. (a) D. J. Payne, M. N. Gwynn, D. J. Holmes, and D. L. Pompliano, Nature Rev. Drug Discovery, 2007, 6, 29; CrossRef (b) F. von Nussbaum, M. Brands, B. Hinzen, S. Weigand, and D. Häbich, Angew. Chem. Int. Ed., 2006, 45, 5072. CrossRef
4. (a) S. Kusari, M. Lamshöft, S. Zühlke, and M. Spiteller, J. Nat. Prod., 2008, 71, 159; CrossRef (b) C.-H. Chen, G. Lang, M. I. Mitova, A. C. Murphy, A. L. J. Cole, L. B. Din, J. W. Blunt, and M. H. G. Munro, J. Org. Chem., 2006, 71, 7947; CrossRef (c) H. He, J. E. Janso, H. Y. Yang, V. S. Bernan, S. B. Lin, and K. Yu, J. Nat. Prod., 2006, 69, 736. CrossRef
5. (a) G. R. Pettit, R. Tan, R. K. Pettit, T. H. Smith, S. Feng, D. L. Doubek, L. Richert, J. Hamblin, C. Weber, and J.-C. Chapuis, J. Nat. Prod., 2007, 70, 1069; CrossRef (b) G. R. Pettit, J. Du, R. K. Pettit, L. A. Richert, F. Hogan, V. J. R. V. Mukku, and M. S. Hoard, J. Nat. Prod., 2006, 69, 804. CrossRef
6. (a) N. G. Vinokurova, I. I. Khmel’nitskaya, B. P. Baskunov, and M. U. Arinbasarov, Appl. Biochem. Microbiol., 2003, 39, 192; CrossRef (b) N. G. Vinokurova, N. E. Ivanushkina, I. I. Khmel’nitskaya, and M. U. Arinbasarov, Appl. Biochem. Microbiol., 2007, 43, 435; CrossRef (c) H.-L. Fang, J.-J. Lai, W.-L. Lin, and W.-C. Lin, Biosci. Biotechnol. Biochem., 2007, 71, 1154. CrossRef
7. (a) Y. Miyake, K. Minato, S. Fukumoto, K. Yamamoto, T. Oya-Ito, S. Kawakishi, and T. Osawa,, Biosci. Biotechnol. Biochem., 2003, 67, 1443; CrossRef (b) H. Esaki, R. Watanabe, H. Onozaki, S. Kawakishi, and T. Osawa, Biosci. Biotechnol. Biochem., 1999, 63, 851; CrossRef (c) Y. Miyake, C. Ito, M. Itoigawa, and T. Osawa, Biosci. Biotechnol. Biochem., 2007, 71, 2515; CrossRef (d) M. Y. Jang, J. Y. Cho, J. L. Cho, J. H. Moon, and K. H. Park, Food Sci. Biotechnol., 2006, 15, 214; (e) Y. Miyake, S. Fukumoto, M. Okada, K. Sakaida, Y. Nakamura, and T. Osawa, J. Agric. Food Chem., 2005, 53, 22; CrossRef (f) H. Esaki, R. Watanabe, T. Osawa, and S. Kawakishi, J. Japanese Soc. Food. Sci. Technol., 2004, 51, 210.
8. (a) H. M. Mora-Montes, E. Lόpez-Romero, S. Zinker, P. Ponce-Noyola, and A. Flores-Carreόn, Antonie van Leeuwenhoek, 2008, 93, 61; CrossRef (b) V. I. Athanasopoulos, K. Niranjan, and R. A. Rastall, Carb. Res., 2005, 340, 609; CrossRef (c) Y. Tatara, T. Yoshida, and E. Ichishima, Biosci. Biotechnol. Biochem., 2005, 69, 2101; CrossRef (d) V. Maitlin, V. Athanasopoulos, and R. A. Rastall, Appl. Microbiol. Biotechnol., 2004, 63, 666; CrossRef (e) V. I. Athanasopoulos, K. Niranjan, and R. A. Rastall, J. Mol. Catalysis B: Enzymatic, 2004, 27, 215. CrossRef
9. (a) Z. Wen, W. Liao, and S. Chen, Process Biochem., 2005, 40, 3087; CrossRef (b) B. Flachner and K. Réczey, Chem. Biochem. Eng. Q., 2004, 18, 303; (c) O. Abdel-Fatah, M. A. Elsayed, and A. M. Elshafei, J. Basic Microbiol., 2003, 43, 439; CrossRef (d) S. Jäger, A. Brumbauer, E. Fehér, K. Réczey, and L. Kiss, World J. Microbiol. Biotechnol., 2001, 17, 455. CrossRef
10. N. Kacem-Chaouche, Z. Maraihi, J. Destain, and P. Thonart, Biotechnol. Agron. Soc. Environ., 2005, 9, 173.
11. A. C. S. Rizzatti, V. C. Sandrim, J. A. Jorge, H. F. Terenzi, and M. D. T. M. Polizeli, J. Industr. Microbiol. Biotechnol., 2004, 31, 88. CrossRef
12. S. W. Cho, N. Kim, M.-U. Choi, and W. Shin, Acta Cryst., 2001, D57, 948.
13. Y. Chiba, T. Midorikawa, and E. Ichishima, Biochem. J., 1995, 308, 405.
14. M. Varoglu and P. Crews, J. Nat. Prod., 2000, 63, 41. CrossRef
15. W. A. Ayer and L. S. Trifonov, Phytochemistry, 1995, 38, 371. CrossRef
16. Y. Venkateswarlu, M. A. Farooq Biabani, and J. V. Rao, J. Nat. Prod., 1995, 58, 269. CrossRef