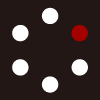
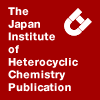
HETEROCYCLES
An International Journal for Reviews and Communications in Heterocyclic ChemistryWeb Edition ISSN: 1881-0942
Published online by The Japan Institute of Heterocyclic Chemistry
e-Journal
Full Text HTML
Received, 6th October, 2008, Accepted, 27th November, 2008, Published online, 8th December, 2008.
DOI: 10.3987/COM-08-S(D)65
■ Indole Alkaloids from the Leaves of Alstonia scholaris
Yusuke Hirasawa, Saori Miyama, Nobuo Kawahara, Yukihiro Goda, Abdul Rahman, Wiwied Ekasari, Aty Widyawaruyanti, Gunawan Indrayanto, Noor Cholies Zaini, and Hiroshi Morita*
Faculty of Pharmaceutical Sciences, Hoshi University, 2-4-41 Ebara, Shinagawa-ku, Tokyo 142-8501, Japan
Abstract
A new indole alkaloid, akuammidine-N-oxide (1) was isolated from the leaves of Alstonia scholaris (Apocynaceae) together with akuammidine (2), and the structure was elucidated by NMR spectral analysis and chemical correlation. Akuammidine (2) showed a moderate antiplasmodial activity.†Dedicated to Dr. John Daly, National Institutes of Health scientist emeritus.
INTRODUCTION
The genus Alstonia and Kopsia (Apocynaceae), which are widely distributed throughout tropical Asia, are noted for producing variety of indole alkaloids with useful biological activities.1 Recent investigation of extracts from the leaves of Alstonia angustiloba,2 Kopsia flavida,3 and Kopsia arborea3 resulted in the isolation of some new indole alkaloids with antiplasmodial and vasorelaxant activities. In this paper, we report the isolation and structure elucidation of a new indole alkaloid, akuammidine-N-oxide (1) from the leaves of Alstonia scholaris, and antiplasmodial activity of isolated indole alkaloids.
The leaves of A. scholaris were extracted with MeOH, and the MeOH extract was partitioned between EtOAc and 3% tartaric acid. Water-soluble materials, which were adjusted to pH 10 with saturated Na2CO3, were extracted with CHCl3. Water-soluble materials were extracted with BuOH. BuOH-soluble materials were subjected to an ODS column (H2O/MeOH 1:1 → 0:1) and the fractions eluted by H2O/MeOH (1:1) were subjected to silica gel columns (CHCl3/MeOH 1:0 → 7:3 and CHCl3/MeOH 1:0 → 5:1) to afford compound 1 (0.001%). CHCl3-soluble materials were subjected to an LH-20 column (CHCl3/MeOH = 1:1) and an amino silica gel column (hexane/EtOAc 8:1 → 1:1 and then CHCl3/MeOH 1:0 → 0:1) to give akuammidine4 (2, 0.04%).
RESULTS AND DISCUSSION
Compound 1{[α]D23 -39 (c 1.0, MeOH)} showed the pseudomolecular ion peak at m/z 369 (M+H)+ in ESIMS, and the molecular formula, C21H24N2O4, was established by HRESIMS [m/z 369.1828, (M+H)+ Δ +1.4 mDa]. IR spectrum suggested the presence of NH (3400 cm-1) and ester carbonyl (1720 cm-1) groups. The 13C NMR (Table 1) spectrum of 1 disclosed twenty-one carbon signals due to one ester carbonyl (δC 172.5), five sp2 quaternary carbons (δC 138.7, 134.0, 131.8, 127.2, and 104.5), one sp3 quaternary carbon (δC 54.4), five sp2 methines (δC 122.7, 120.9, 120.3, 119.0, and 112.3), three sp3 methines (δC 72.8, 67.9, and 29.6), four sp3 methylenes (δC 71.6, 68.0, 31.7, and 20.9), one methyl (δC 13.1), and one methoxy group (δC 52.2). 1H and 13C signals for 1 were assigned by detailed analysis of the HSQC spectrum. The 1H-1H COSY spectrum revealed connectivities of C-5 to C-6, C-9 to C-12, C-3 to C-14, C-14 to C-15, and C-18 to C-19 (Figure 1). HMBC correlations of H-3 (δH 4.59) to C-2 (δC 134.0), H-5 (δH 3.17) to C-3 (δC 67.9) and C-7 (δC 104.5), H2-6 (δH 3.51) to C-2 and C-7, H2-14 (δH 2.41 and 2.96) to C-20 (δC 131.8), and H-15 (δH 3.35) to C-21 (δC 71.6) revealed the presence of a dehydroquinolizidine ring (C-2 to C-7, C-14 to C-15, C-20 to C-21, and N-4). Low field chemical shifts at C-3, C-5, and C-21 around N-4 atom [δC 67.9, 72.8, and 71.6, respectively] suggested that 1 was N-oxide form at N-4. The presence of the piperidine ring (C-5, C-3, C-14 to C-16, and N-4) was deduced from the HMBC correlations of H2-14 to C-16, and H2-6 to C-16. Connection between indole ring (C-2, C-7 to C-13 and N-1) and dehydroquinolizidine ring was deduced from the HMBC correlations of H-9 (δH 7.44) to C-7. Structure of methyl 3-hydroxypropanoate moiety (C-16 to C-17, C-22, and C-23) was elucidated from HMBC correlations of H-5 to C-17 and C-22 (δH 172.5), H-15 to C-17, and H3-23 (δH 2.98) to C-22. The HMBC cross-peaks of H3-18 (δH 1.73) to C-20 indicate the ethylidene side chain at C-20. Thus, the gross structure of 1 was assigned as sarpagine-type skeletal system with an N-4 oxide.
The relative stereochemistry of 1 was elucidated by NOESY correlations as shown in computer-generated 3D drawing (Figure 2). Configuration of C-16 was elucidated by NOESY correlations among H3-23, H-6, and H-9, and between H2-17 and H-5. NOESY correlations of H-19 to H-21 indicated the geometry of C-19 - C-20 double bond was E. Thus, the relative stereochemistry of 1 was assigned as shown in Figure 2.
Treatment of compound 1 with Na2SO3 in aqueous MeOH afforded the reductive derivative, whose spectroscopic data and specific rotation were identical with akuammidine (2).4 Thus, the absolute configuration of 1 was assigned as the same as 2.
Malaria caused by parasites of the genus Plasmodium is one of the leading infectious diseases in many tropical and some temperate regions.5 The emergence of widespread chloroquine-resistant and multiple-drug-resistant strains of malaria parasites leads to the need for the development of new therapeutic agents against malaria.6 Since some indole alkaloids from Alstonia species have already been reported on inhibitory activity against some parasites.7 Akuammidine (2) showed a moderate in vitro antiplasmodial activity against Plasmodium falciparum (2: 32.6% inhibition at 10 μg/mL), whereas akuammidine-N-oxide (1) did not show (>10 μg/mL).8
EXPERIMENTAL
General Experimental Procedures. 1H and 2D NMR spectra were recorded on a JEOL ECA600 spectrometer and chemical shifts were reported using residual CD3OD (δH 3.31 and δC 49.0) as internal standards. HSQC experiments were optimized for 1JCH=145 Hz and HMBC experiments for nJCH=8Hz. Mass spectra were recorded on a Micromass LCT spectrometer.
Plant Material. The leaves of Alstonia scholaris were collected in Purwodadi Botanical Garden, East Java, Indonesia in 2006. A voucher specimen is deposited at the Purwodadi Botanical Garden, Indonesia.
Extraction and Isolation. The leaves of A. scholaris (500 g) were extracted with MeOH, and the MeOH extract was partitioned between EtOAc and 3% tartaric acid. Water-soluble materials, which were adjusted to pH 10 with saturated aqueous Na2CO3, were extracted with CHCl3. Water-soluble materials were extracted with BuOH. BuOH-soluble materials were subjected to an ODS column (H2O/MeOH 1:1 → 0:1) and the fractions eluted by H2O/MeOH (1:1) were subjected to silica gel columns (CHCl3 /MeOH, 1:0 → 7:3 and CHCl3/MeOH, 1:0 → 5:1) to afford compound 1 (0.001%).
CHCl3-soluble materials were subjected to an LH-20 column (CHCl3/MeOH, 1:1) and an amino silica gel column (hexane/EtOAc, 8:1 → 1:1 and then CHCl3/MeOH, 1:0 → 0:1) to give akuammidine4 (2, 0.04%).
Akuammidine-N-oxide (1): colorless amorphous solid; [α]D23 -39 (c 1.0, MeOH ); IR (film) νmax 3400, 2940, 2360, 1720, and 1630 cm-1; UV (MeOH) λmax 270 (ε 1000) and 205 (ε 5000) nm; 1H and 13C NMR data (Table 1); ESIMS m/z 369 (M+H)+; HRESITOFMS m/z 369.1828 [(M+H)+, calcd for C21H25N2O4, 369.1814].
Chemical conversion of akuammidine-N-oxide (1) into akuammidine (2). To a solution of akuammidine-N-oxide (1, 0.2 mg) in aqueous MeOH (0.2 mL) was added Na2SO3 (1.0 mg) and the mixture was kept at rt for 30 min. After evaporation, the residue was applied to a silica gel column (CHCl3/MeOH, 4:1) to give a compound (0.15 mg), whose spectroscopic data and [α]D value were identical with those of natural akuammidine (2).
Antiplasmodial Assay. Human malaria parasites were cultured according to the method by W. Trager et al.8 The antimalarial activity of the isolated compounds was determined by the procedure described by Budimulja et al.8 In brief, Stock solution of the samples were prepared in DMSO (final DMSO concentrations of < 0.5%) and were diluted to the required concentration with complete medium (RPMI 1640 supplemented with 10% human plasma, 25 mM HEPES and 25 mM NaHCO3) until the final concentration of samples at well culture plate were: 10; 1; 0.1; 0.01; 0.001 μg/mL. The malarial parasite P. falciparum 3D7 clone was propagated in a 24-well culture plate in the presence of a wide range of concentrations of each compound. The growth of the par¬asite was monitored by making a blood smear fixed with MeOH and stained with Geimsa solution. The antimalarial activity of each compound was expressed as an IC50 value, defined as the concentration of the compound causing 50% inhibition of parasite growth relative to an untreated con¬trol.
The percentage of growth inhibition was expressed according to following equation : Growth inhibition % = 100 - [(test parasitaemia/control parasitemia) × 100. Chloroqine: IC50= 0.0061 μg/mL.
ACKNOWLEDGMENTS
This work was supported by a Grant-in-Aid for Scientific Research from the Ministry of Education, Culture, Sports, Science, and Technology of Japan, and grants from the Research Foundation for pharmaceutical Sciences and The Open Research Center Project. We also acknowledge the financial support provided by Assessment Service Unit, Faculty of Pharmacy, Airlangga University.
References
1. (a) P. Kamarajan, N. Sekar, V. Mathuram, and S. Govindasamy, Biochem. Int., 1991, 25, 491; (b) V. saraswathi, S. Subramanian, N. Ramamoorth, V. Mathuram, and S. Govindasamy, Med. Sci. Res., 1997, 25, 167; (c) N. Keawpradub, G. C. Kirby, J. C. P. Steele, and P. J. Houghton, Planta Med., 1999, 65, 690; CrossRef (d) K. Husain, I. Jantan, N. Kamaruddin, I. M. Said, N. Aimi, and H. Takayama, Phytochemistry, 2001, 57, 603; CrossRef (e) K. Husain, I. Jantan, I. M. Said, N. Aimi, and H. Takayama, J. Asian Nat. Prod. Res., 2003, 5, 63; CrossRef (f) G. C. Jagetia and M. S. Baliga, Phytother Res., 2006, 20, 103; CrossRef (g) M. R. Khan, A. D. Omoloso, and M. Kihara, Fitoterapia, 2003, 74, 736; CrossRef (h) R. S. Gupta, A. K. Bhatnager, Y. C. Joshi, M. C. Sharma, V. Khushalani, and J. B. Kachhawa, Pharmacology, 2005, 75, 57. CrossRef
2. K. Koyama, Y. Hirasawa, K. Zaima, T. C. Hoe, K. L, Chan, and H. Morita, Bioorg. Med. Chem., 2007, 16, 6483. CrossRef
3. (a) M. Sekiguchi, Y. Hirasawa, K. Zaima, T. C. Hoe, K.-L. Chan, and H. Morita, Heterocycles, 2008, 75, 2283; CrossRef (b) M. Sekiguchi, Y. Hirasawa, K. Zaima, T. C. Hoe, K.-L. Chan, and H. Morita, Heterocycles, 2008, 76, 867; CrossRef (c) K. Zaima, Y. Matsuno, Y. Hirasawa, A. Rahman, G. Indrayanto, N. C. Zaini, and H. Morita, Heterocycles, 2008, 75, 2535. CrossRef
4. W. Boonchuay and W. E. Court, Planta Med., 1976, 29, 380. CrossRef
5. J. Wiesner, R. Ortmann, H. Jomaa, and M. Schlitzer, Angew. Chem. Int. Ed., 2003, 42, 5274. CrossRef
6. M. H. Gelb and W. G. Hol, Science, 2002, 297, 343. CrossRef
7. T. Yamauchi and F. Abe, International Congress Series 1157 (Towards Natural Medicine Research in the 21st Century): 51-58.
8. (a) W. Trager and J. B. Jensen, Science, 1976, 193, 673; CrossRef (b) A. S. Budimulja, Syafruddin, P. Tapchaisri, P. Wilairat, and S. Marzuki, Mol. Biochem. Parasitol., 1997, 84, 137. CrossRef