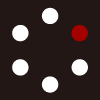
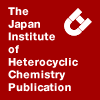
HETEROCYCLES
An International Journal for Reviews and Communications in Heterocyclic ChemistryWeb Edition ISSN: 1881-0942
Published online by The Japan Institute of Heterocyclic Chemistry
e-Journal
Full Text HTML
Received, 6th October, 2008, Accepted, 4th November, 2008, Published online, 7th November, 2008.
DOI: 10.3987/REV-08-647
■ Recent Advances in the Total Synthesis of Xanthanolide Sesquiterpenoids
Kozo Shishido*
Graduate School of Pharmaceutical Sciences, University of Tokushima, Sho-machi, Tokushima 770-8505, Japan
Abstract
Recent advances in the total synthesis of xanthanolide/dinorxanthanolide sesquiterpenoids are described. This family of natural products can be divided into two structural classes according to the stereochemistry at C8 of the basic carbon framework, the oxabicyclo[5.3.0]decene core, i.e., the cis- and trans-fused γ-butyrolactone series. This article reviews the successful total syntheses of the five natural products, 8-epi-xanthatin (1) and sundiversifolide (2) (the cis-series), and 11α,13-dihydroxanthatin (3), xanthatin (4) and diversifolide (5) (the trans-series).Xanthanolide sesquiterpenoids1 are a class of natural products isolated primarily from the genus Xanthium (Compositae). They possess a characteristic oxabicyclo[5.3.0]decene core as their common basic carbon framework and exhibit a wide variety of biological activities, e.g. anti-tumor,2 anti-malarial3 and anti-bacterial, particularly against MRSA.4 Two other dinorxanthane sesquiterpene lactones, sundiversifolide (2)5 and diversifolide (5),6 have also been isolated, sundiversifolide from the sunflower (Helianthus annuus L., Asteraceae) and diversifolide from the perennial herb (Tithonia T. diversifolia (Hernsl.) A. Gray, Comositae), respectively. Sundiversifolide (2), which has been found to contain a plant growth-inhibiting substance (allelochemical), potentially could become a lead for the development of environmentally benign herbicides. Structurally, the xanthanolide and dinorxanthanolide sesquiterpenoids can be divided into either the cis- or the trans-fused γ-butyrolactone series, depending on the stereochemistry at C8. Not surprisingly, the promising biological profiles and intriguing chemical structures of these compounds have stimulated intense interest in these natural products resulting in numerous synthetic studies being directed towards their total synthesis over the past several years. In this review, we focus on the recent advances in the successful total syntheses of five kinds of xanthanolide and dinorxanthanolide sesquiterpenoids: 8-epi-xanthatin (1) and sundiversifolide (2) (the cis-series), and 11α,13-dihydroxanthatin (3), xanthatin (4) and diversifolide (5) (the trans-series). (Figure 1)
Total synthesis of (+)-8-epi-xanthatin9
8-epi-Xanthatin (1), commonly isolated from the extracts of the aerial parts of various species in the genus Xanthium,7 has also been obtained upon elimination of acetic acid from xanthumin (8-epi-2-acetoxy-3-hydroxanthatin).8 It has been shown to inhibit larval growth of Drosophila melanogaster (fruit fly)7c and the in vivo proliferation of several cultured human tumor cell lines.2 Not only does it display anti-malarial activity,3 but it was also found to inhibit the farnesylation process of human lamin-B by farnesyltransferase in a dose-dependent manner in vitro.2b A representative of the cis-fused γ-lactone series possessing a characteristic (E)-conjugated dienone functionality, this natural product was synthesized in 2005, by Marin et al. in the first reported total synthesis of 1.9 Its key features are 1) a sequence for palladium-catalyzed carbonylation of an enol triflate10 and lactonization to construct the α-methylene-γ-butyrolactone functionality, and 2) a fascinating domino enyne ring closing metathesis (RCM)/cross metathesis (CM)11 process to elaborate the seven-membered carbocycle with its pendant enone array (6 → 1). The lactone 6 with the requisite stereochemistry would be prepared via an asymmetric aldol coupling of 7 with the aldehyde 8. (Scheme 1) The synthesis οf the trans-fused xanthanolide, 11α,13-dihydroxanthatin 3, was also reported at approximately the same time by the group of Morken 12 employing a similar key strategy, i.e., enyne RCM13 followed by CM,14 for the construction of the seven-membered ring and the conjugated dienone moiety.
The total synthesis of the 8-epi-xanthatin (1) is shown in Scheme 2. The enantiomerically pure 9 was converted via the dibromoalkene 10 to the acetylenic aldehyde 8 which was treated with 7 to give the aldol adduct 11 with high diastereoselectivity. The enyne 14 with the requisite stereochemistry was prepared by palladium-catalyzed carbomethoxylation10 of the enol triflate 13, formed using the Comins triflimide15 via the Weinreb amide 12.16 Desilylation of 14 with TBAF led to the spontaneous formation of the α-methylene-γ-butyrolactone 6. Finally, the pivotal domino enyne RCM/CM reaction11 of 6 in the presence of methyl vinyl ketone and the Grubbs-Hoveyda catalyst 1517 was realized to give (+)-1 in 83% yield. The total synthesis was completed efficiently by a route that required only 14 steps in the longest linear sequence and proceeded in an overall yield of 5.5%. (Scheme 2)
First total synthesis of (+)-sundiversifolide18
Sundiversifolide (2) was isolated from the exudates of Helianthus annuus L., germinating sunflower seeds.5 This compound inhibited shoot and root growth of cat’s-eye by about 50% at a concentration of 30 ppm and also showed species-selective activity in the shoot and root growth of various tested plants, e.g. tomato, crabgrass and barnyard grass. Although sundiversifolide has been recognized as having an allelopathic function in sunflowers, interestingly, it did not inhibit shoot growth of the sunflower itself.
The first total synthesis was accomplished by our group in 2007,18 the key features of which include (i) the use of sequential RCM14 and diastereoselective reduction (18 → 17), (ii) the Eschenmoser-Claisen rearrangement19 followed by iodolactonization, reduction and acetalization (17 → 16) to install the cis-fused oxabicyclo[5.3.0]decene core, and (iii) the use of the Maruoka-Yamamoto methylaluminum bis(2,6-diphenylphenoxide) (MAPH)-mediated carbonyl ene-reaction20 of 16 to construct the olefinic ethanol functionality. (Scheme 3)
The RCM of the optically pure diene 18, which was prepared starting from an asymmetric aldol reaction of 19 and 20, using the second-generation Grubbs catalyst 22, provided efficiently the seven-membered enone 23. Reduction with Red-Al® produced the alcohol 17 with the (R)-configuration in a highly diastereoselective fashion. The Eschenmoser-Claisen rearrangement resulted in the formation of 24 as a single product. Construction of the bicyclic core with the olefinic ethanol appendage was achieved by a sequential iodolactonization, Peterson olefination,21 and the MAPH-mediated carbonyl ene-reaction to give 28 in good overall yield. Installation of the β-methyl group at C11 of 29 was realized via successive α-selective methylation and epimerization by kinetic protonation to give a 2.8:1 separable mixture of sundiversifolide 2 and the epimer 30. Thus the first total synthesis of the natural enantiomer (+)-2 was completed in an overall yield of 13.3% (21 steps from 19). (Scheme 2)
Second total synthesis of (+)- and (–)-sundiversifolide22
Another synthesis of sundiversifolide has been described by Shindo et al.22 Among its interesting aspects should be noted 1) the intramolecular acylation23 of the organolithium species 33 for the elaboration of the functionalized cycloheptanone 32 and a sequential intramolecular Wittig reaction of 31, and 2) the diastereoselective reduction of the resulting butenolide for the construction of the cis-fused α-methyl-γ-lactone moiety with the requisite stereochemistry in 2. (Scheme 5)
The synthesis began with the conversion of 3-butyn-1-ol 34 to the iodide 35, which was subjected to asymmetric alkylation with 20 to give 36 with high diastereoselectivity. Asymmetric dihydroxylation using ADmix-β produced the hydroxy lactone 37 efficiently. A pivotal cyclization of the iodo lactone 38 using tBuLi provided an equilibrium mixture of 39 and 40,24 which was converted to the hydroxy hemiacetal 41 by a five-step sequence of reactions. Treatment of 41 with the Wittig reagent (Ph3P=C(Me)CO2CH2CF3) provided the butenolide 43 presumably via transesterification giving the intermediate 42 followed by intramolecular Wittig olefination. The structure of 43 was unambiguously established by X-ray crystallographic analysis. After epoxidation, the epoxide 44 was treated with nickel boride (generated in situ from NiCl2 and NaBH4)25 to give the bicyclic cis-lactone 45 (81%) along with the C11-epimer (14%). Dehydration followed by regioselective cleavage of the epoxide in 46 with ZnI2 and NaBH3CN26 provided (+)-sundiversifolide 2. (Scheme 6) The total synthesis was completed efficiently in 25% overall yield. In addition, the unnatural enantiomer (–)-2 was also synthesized in a similar manner, using the chiral oxazolidinone ent-20 derived from L-Phe in the asymmetric alkylation and ADmix-α in the asymmetric dihydroxylation. It should be emphasized that the absolute structure of the natural sundiversifolide was established by this work, i.e., HPLC analysis using a chiral column indicated that the natural 2 was identical with the synthetic (+)-2.
Third total synthesis of (–)-sundiversifolide31
In the course of our program directed toward the search for promising lead compounds for the development of new allelochemicals and antibacterial drugs, we decided to undertake the synthesis of the unnatural enantiomer of sundiversifolide (–)-2 employing a new strategy for the construction of the key intermediate 25 in the synthesis of (+)-2. From a retrosynthetic perspective, (–)-2 would be derived from the bicyclic lactone ent-25 via the procedure we developed in the synthesis of the natural enantiomer (+)-2 (Scheme 4). For the synthesis of (–)-25, the diastereoselective 1,3-dipolar [3+2] cycloaddition reaction27 of the nitrile oxide (48 → 47) was used as the key reaction step.
The known aldol product 49 was converted to compound 52 possessing the oxime and the conjugate ester moieties via 50 and 51. Treatment of 52 with a 7% aqueous solution of NaOCl in CH2Cl2 provided the isoxazoline 47 as the major product (dr=7:1). The diastereoselectivity can be explained by postulating that the transition state has the conformation shown in 48.28 After reductive hydrolysis, the resulting keto alcohol 53 was sequentially treated with Zn(BH4)229 and p-TsOH to give the α-hydroxy-γ-butyrolactone 54, which was exposed to SmI230 providing the requisite ent-25. According to the protocol for the synthesis of (+)-2, ent-25 was converted to ent-2. (Scheme 6)31
Total synthesis of (±)-sundiversifolide32
A concise synthesis of racemic sundiversifolide was reported by Takikawa et al.32 The retrosynthetic analysis is shown in Scheme 9. The key step of the synthesis is a Lewis acid-mediated Claisen rearrangement33 (56 → 55) for the construction of the cycloheptenone core, with substrate 56 being prepared from the known keto ester 58 via an iodoetherification of the dienyl alcohol 57. (Scheme 9)
The keto ester 58 was converted in four steps into 59 and sequential vinylation, iodoetherification and β-elimination provided the cyclic allyl vinyl ether 60. Treatment of 60 with triisobutylaluminium (TIBAL) in toluene34 (necessary to promote higher yields) resulted in the formation of the cycloheptenol 61 (51% yield from 59) through Claisen rearrangement and reduction of the resulting ketone 55. The kinetically controlled regioselective introduction of the acetate unit at C7 (sundiversifolide numbering) was realized to give a 1:1 diastereomeric mixture of the keto esters 62 in 68% yield. Reduction with L-Selectride® produced a diastereomeric mixture of the cis-lactones 63 and 64 in 39% and 38% yield, respectively. Methylation followed by desilylation provided (±)-30, which was exposed to kinetic protonation conditions to give a separable 1.1:1 mixture of (±)-2 and (±)-30 in good yield. The overall yield of (±)-2 was 3.5% (14 steps from 58). (Scheme 10)
Total synthesis of (–)-11α,13-dihydroxanthatin12
11α,13-Dihydroxanthatin 3 was isolated from Xanthium strumarium as a representative of the trans-fused xanthanolide sesquiterpene lactones.35 The chemical structure of 3 contains a β-oriented methyl group instead of the exocyclic methylene unit present in xanthatin 4, at the α position of the γ-butyrolactone ring. Although the biological profile of 3 has never been reported, a comparison of the antifungal activity of xanthatin 4 with that of dihydroxanthatin 3, which lacks any antifungal activity at a dose of 250 µg, clearly indicates that the α-methylene-γ-butyrolactone functionality is essential for the biological activity.36 In 2005, for the first time, an asymmetric total synthesis of 11α,13-dihydroxanthatin 3 was completed by Morken et al.12 and includes as key features 1) the use of a sequential enyne RCM and CM14c for the construction of the seven-membered carbocycle with the dienone functionality and 2) an application of the stereoselective Oshima-Utimoto reaction37 to elaborate the trans-fused butyrolactone moiety (66 → 65). (Scheme 11)
The commercially available bromoalcohol 67 was converted via a seven-step sequence to the chiral enone 69, which was reduced to 66 using the R-enantiomer of the Itsuno-Corey oxazaborolidine 70.38 The allyl alcohol 66 was subjected to the catalytic Oshima-Utimoto reaction, namely, treatment with butyl vinyl ether and Cu(OAc)2 in the presence of 10 mol% of Pd(OAc)2 to give 65, via transition state 71, in 68% yield with >12:1 diastereoselectivity. After homologation followed by deprotection, the resulting 72 was oxidized with Dess-Marin periodinane, treated with the Gilbert-Seyferth reagent39 and oxidized with chromic acid to give the enyne lactone 73. Treatment of 73 with the Grubbs catalyst 22 provided the bicyclic diene 74. Methylation with LDA and MeI gave the β-methylated lactone 75 diastereoselectively (dr=>20:1). 40 Finally, the CM of 75 with methyl vinyl ketone using the same catalyst 22 as in the case of the enyne RCM provided (–)-11α,13-dihydroxanthanolide 3 in an overall yield of 5.6% (19 steps from 67). (Scheme 12)
Total synthesis of (–)-xanthatin45
Xanthatin 4 was first isolated from Xanthium pennsylvanicum by Little et al. in their examination of the cocklebur for its antibacterial properties.41 Its structure was established by Geissman et al.42 who suggested that 4 would be derived from xanthinin (2-acetoxy-3-hydroxanthatin) during isolation, particularly on column chromatography.42a Xanthatin has also been isolated from X. strumarium,42 X. sibiricum,4 and X. macrocarpum,43 and exhibits potent antibacterial activity against the Staphylococcus aureus species, including MRSA.4 It was recently reported that xanthatin suppressed the expression of iNOS and COX-2 and the activity of NF-κB through the inhibition of LPS-induced I-κB-α degradation in microglia.44 The first total synthesis was accomplished in 2008 by our group based on the strategy outlined in Scheme 13.45 As the key intermediate for the total synthesis, the bicyclic dienyl lactone 76, a penultimate intermediate in Morken’s total synthesis of 3,12 was selected. It was thought that the exocyclic methylene could be installed via a syn-elimination of a selenoxide by taking into account the stereochemical nature of 76 for the diastereoselective introduction of the electrophile from the β-face of the lactone enolate; the total synthesis would be completed by CM of 76 with methyl vinyl ketone. The intermediate 76 would be prepared via the Mitsunobu inversion at C8 of the cis-fused lactone 26, which is a key intermediate in our total synthesis of sudiversifolide.18 (Scheme 13)
The lactone ring opening of 26 was achieved by treatment with Et2NH and AlCl346 to give the amide alcohol 77, which was exposed to the Mitsunobu reaction using p-nitrobenzoic acid,47 diisopropyl azodicarboxylate and Ph3P to provide the benzoate 78 with inversion of configuration at the future C8. After hydrolysis and acidification, the resulting trans-fused lactone 79 was converted in five steps to 80. This compound was then subjected to the MAPH-catalyzed carbonyl ene-reaction followed by regeneration of the lactone ring to furnish 82. Desilylation and subsequent dehydration of the primary alcohol moiety by the Nishizawa-Grieco protocol48 provided the diene 75, which was methylated to give 76 as a single diastereoisomer. The spectral properties of 75 and 76 were identical with those reported by Morken indicating that an alternative synthesis of 11α,13-dihydroxanthatin has been formally accomplished at this stage. Phenylselenylation49 of 76 provided diastereoselectively the selenide 83, with the β-oriented phenylselenyl function, which was oxidized with H2O2 leading to the spontaneous formation of the α-methylene lactone 84 in good yield. Final CM was achieved using the Grubbs-Hoveyda catalyst 1517 to complete the total synthesis of (–)-xanthatin 4. The overall yield was 5.4% (18 steps from 26). (Scheme 14)
Total synthesis and structural revision of (–)-diversifolide50
Diversifolide 5 was isolated from the perennial herb Tithonia T. diversifolia (Hernsl.) A. Gray (Comositae) by Kuo et al. in 1999.6 Its aerial parts have been used as a traditional treatment for hepatitis and hepatoma. Although a biological profile of diversifolide itself has never been reported, the compound was nonetheless expected to possess some biological activity. Kuo, in his report, did not establish the absolute configuration of 5 but, on spectroscopic analysis, assigned the structure to be a trans-fused dinorxanthanolide sesquiterpenoid. Because of the structural similarity of sundiversifolide and its potential biological activities, the Shindo and Shishido groups directed their efforts towards the total synthesis of 5,50 starting from the key intermediates 4422 and 82,45 in the syntheses of sundiversifolide 2 and xanthatin 4, respectively, as shown in Scheme 15.
As shown in the synthesis of sundiversifolide, when the bicyclic butenolide 44 was reduced with nickel boride, the two cis-lactones 45 and the C11-epimer 85 were obtained as a chromatographically separable mixture in 81% and 14% yield, respectively. The minor diastereomer 85 was converted via 30 (11-epi-sundiversifolide) to the ring-opened keto amide 86, which was reduced with SmI2 to give the alcohol 87 with the desired configuration at C8. After lactonization by acidic treatment and deprotection, diversifolide 5 was obtained. Alternatively, 5 was synthesized from the bicyclic trans-lactone 82. Treatment of 82 with LDA and MeI provided the undesired C11-epimer 88 as the sole product. Attempted inversion at C11 using kinetic protonation conditions produced a separable 1:1 mixture of diastereoisomers, the desired isomer of which was desilylated to give 5. However, the spectral data (1H and 13C NMR) of 5 did not match those for the natural material reported by Kuo. Since the stereochemistry was confirmed by the NOE spectrum of 88, Kuo’s proposed stereochemistry has proven to be incorrect. After careful examination of the NMR spectra of the synthetic intermediates, we noticed that all the spectral data of the synthetic intermediate 30, 11-epi-sundiversifolide (the C8-epimer of Kuo’s assigned structure 5), were identical with the reported values. Although there is a slight discrepancy in the chemical shift, we concluded that natural diversifolide is most likely the compound represented by 30. The optical rotation allowed us to determine the absolute configuration of the diversifolide as shown in 30. (Scheme 16)
CONCLUSION
In this article, we have described the successful total syntheses of the xanthanolide/dinorxanthanolide sesquiterpenoids, focusing on the five natural products of this family that have been reported since 2005. Because of their complex structural features and biological importance, the challenge to synthesize these interesting sesquiterpene lactones has tested the ingenuity of the synthetic community resulting in much interesting, important and novel chemistry. We think that even more valuable data will be forthcoming in the continuing, concerted efforts to synthesize the xanthanolide terpenoids.
ACKNOWLEDGEMENTS
The work accomplished in our laboratories were supported financially by a Grant-in-Aid for Scientific Research (A) (No. 16209001) from the Japan Society for the Promotion of Science. The author thanks his graduate students, Hiromasa Yokoe and Makoto Kanematsu, for their help in preparing this manuscript.
References
1. B. M. Fraga, Nat. Prod. Rep., 1999, 16, 711. CrossRef
2. (a) J.–W. Ahn, Z. No, S.–Y. Ryu, O.–P. Zee, and S.–K. Kim, Nat. Prod. Sci., 1995, 1, 1; (b) Y. S. Kim, J. S. Kim, S.-H. Park, S.-U. Choi, C. O. Lee, S.-K. Kim, S. H. Kim, and S. Y. Ryu, Planta Med., 2003, 69, 375. CrossRef
3. S. P. Joshi, S. R. Rojatkar, and B. A. Nagasampagi, J. Med. Aromatic Plant Sci., 1997, 19, 366.
4. Y. Sato, H. Oketani, T. Yamada, K. Shingyouchi, T. Ohtsubo, M. Kihara, H. Shibata, and. T. Higuti,, J. Pharm. Pharmacol., 1997, 49, 1042.
5. S. Ohno, K. Tomita-Yokotani, S. Kosemura, M. Node, T. Suzuki, M. Amano, K. Yasui, T. Goto, S. Yamamura, and K. Hasegawa, Phytochemistry, 2001, 56, 577. CrossRef
6. Y.-H. Kuo and B.-Y. Lin, Chem. Pharm. Bull., 1999, 47, 428.
7. (a) N. M. Ghazy, A. A. Omar, E. M. Elrashidy, and A. M. Metwally, Egypt. J. Pharm. Sci., 1988, 29, 39; (b) C. McMillan, P. I. Chavez, and T. J. Mabry, J. Biochem. Syst. Ecol., 1975, 3, 137; CrossRef (c) K. Kawazu, S. Nakajima, and M. Ariwa, Experientia, 1979, 35, 1294; CrossRef (d) F. Bohlmann, P. Singh, K. C. Joshi, and C. L. Singh, Phytochemistry, 1982, 21, 1441; CrossRef (e) A. A. Ahmed, J. Jakupovic, F. Bohlmann, H. A. Regaila, and A. M. Ahmed, Phytochemistry, 1990, 29, 2211; CrossRef 8-epi-Xanthatin (1) has also been isolated from de-etiolated sunflower (Helianthus annuus) seedlings as a plant growth-inhibiting substance, see: K. Yokotani-Tomita, J. Kato, S. Kosemura, S. Yamamura, M. Kushima, H. Kakuta, and K. Hasegawa, Phytochemistry, 1997, 46, 503. CrossRef
8. H. Minato and I. Horibe, J. Chem. Soc., 1965, 7009. CrossRef
9. (a) D. A. Kumar, J. B. Brenneman, and S. F. Martin, Org. Lett., 2005, 7, 4621; CrossRef (b) D. A. Kumar, J. B. Brenneman, and S. F. Martin, Tetrahedron, 2006, 62, 11437. CrossRef
10. S. Cacchi, E. Morera, and G. Ortar, Tetrahedron Lett., 1985, 26, 1109. CrossRef
11. (a) S. Randl, N. Lucas, S. J. Connon, and S. Blechert, Adv. Syn. Catal., 2002, 344, 631; CrossRef (b) F. Royer, C. Vilain, L. Elkaim, and L. Grimaud, Org. Lett., 2003, 5, 2007; CrossRef (c) H.-Y. Lee, H. Y. Kim, H. Tae, B. G. Kim, and J. Lee, Org. Lett., 2003, 5, 3439; CrossRef (d) J. S. Clark, F. Elustondo, and M. C. Kimber, Chem. Commun., 2004, 2470; CrossRef (e) T. Kitamura, Y. Sato, and M. Mori, Tetrahedron, 2004, 60, 9649; CrossRef (f) S. S. Salim, R. K. Bellingham, and R. C. D. Brown, Eur. J. Org. Chem., 2004, 800. CrossRef
12. M. A. Evans and J. P. Morken, Org. Lett., 2005, 7, 3371. CrossRef
13. For a review, see: S. T. Diver and A. J. Glessert, Chem. Rev., 2004, 104, 1317. CrossRef
14. For reviews on methathesis, see: (a) T. M. Trnka and R. H. Grubbs, Acc. Chem. Res., 2001, 34, 18; CrossRef A. Furstner, Angew. Chem. Int. Ed., 2000, 39, 3012; CrossRef S. J. Connon and S. Blechert, Angew. Chem. Int. Ed., 2003, 42, 1900; CrossRef R. H. Schrock and A. H. Hoveyda, Angew. Chem. Int. Ed., 2003, 42, 4592; CrossRef R. H. Grubbs, Tetrahedron, 2004, 60, 7117; CrossRef Y. Schrodi and R. L. Pederson, Aldrichimica Acta, 2007, 40, 45; A. H. Hoveyda and R. R. Zhugralin, Nature, 2007, 450, 243. CrossRef
15. D. L. Comins and A. Dehghani, Tetrahedron Lett., 1992, 33, 6299. CrossRef
16. (a) A. Basha, M. Lipton, and S. M. Weinreb, Tetrahedron Lett., 1977, 48, 4171; CrossRef (b) J. I. Levin, E. Turos, and S. M. Weinreb, Synth. Commun., 1982, 12, 989; CrossRef (c) D. A. Evans and W. C. Black, J. Am. Chem. Soc., 1993, 115, 4497. CrossRef
17. S. B. Garber, J. S. Kingsbury, B. L. Gray, and A. H. Hoveyda, J. Am. Chem. Soc., 2000, 122, 8168. CrossRef
18. H. Yokoe, H. Sasaki, T. Yoshimura, M. Shindo, M. Yoshida, and K. Shishido, Org. Lett., 2007, 9, 969. CrossRef
19. (a) A. Eschenmoser, A. E. Wick, S. Felix, and K. Steen, Helv. Chim. Acta, 1964, 47, 2425; CrossRef (b) J. Mulzer, A. Galkina, and M. W. Gilbert, Synlett, 2004, 14, 2558. CrossRef
20. K. Maruoka, A. B. Concepcion, N. Murase, M. Oishi, N. Hirayama, and H. Yamamoto, J. Am. Chem. Soc., 1993, 115, 3943. CrossRef
21. (a) D. J. Peterson, J. Org. Chem., 1968, 33, 780; CrossRef (b) A. Vazquez and R. M. Williams, J. Org. Chem., 2000, 65, 7865. CrossRef
22. K. Ohtsuki, K. Matsuo, T. Yoshikawa, C. Moriya, K. Tomita-Yokotani, K. Shishido, and M. Shindo, Org. Lett., 2008, 10, 1247. CrossRef
23. A relevant protocol using SmI2 has been reported, see: (a) G. A. Molander, Acc. Chem. Res., 1998, 31, 603; CrossRef K. Kawamura, H. Hinou, G. Matsuo, and T. Nakata, Tetrahedron Lett., 2003, 44, 5259. CrossRef
24. A similar type of intramolecular Barbier reaction has been reported by Nakata et al. (Scheme 17) T. Saito, T. Takeuchi, M. Matsuhashi, and T. Nakata, Heterocycles, 2007, 72, 151. CrossRef
25. F. Kido, K. Tsutsumi, R. Murata, and A. Yoshikoshi, J. Am. Chem. Soc., 1979, 101, 6420. CrossRef
26. L. M. Finkielsztein, J. M. Aguirre, B. Lantano, E. N. Alesso, and G. Y. Moltrasio Iglesias, Synth. Commun., 2004, 34, 895. CrossRef
27. For reviews, see: P. N. Confalone and E. M. Huie, Org. React., 1988, 36, 1420; V. Nair and T. D. Suja, Tetrahedron, 2007, 63, 12247. CrossRef
28. (a) O. Duclos, A. Dureault, and J. C. Depezay, Tetrahedron Lett., 1992, 33, 1059; CrossRef (b) K. Shishido, K. Umimoto, and M. Shibuya, Heterocycles, 1994, 38, 641; CrossRef (c) A. Nivlet, L. Dechoux, T. L. Gall, and C. Mioskowski, Eur. J. Org. Chem., 1999, 3251; CrossRef (d) M.–C. P. Yeh, C.–F. Jou, W.-T. Yeh, D.–Y. Chiu, and N. R. K. Reddy, Tetrahedron, 2005, 61, 493. CrossRef
29. T. Nakata, Y. Tani, M. Hatozaki, and T. Oishi, Chem. Pharm. Bull., 1984, 32, 1411.
30. L. A. Paquette, C. K. Seekamp, A. L. Kahane, D. G. Hilmey, and J. Gallucci, J. Org. Chem., 2004, 69, 7442. CrossRef
31. H. Sasaki, H. Yokoe, M. Shindo, M. Yoshida, and K. Shishido, Heterocycles, 2009, 77, 773. CrossRef
32. T. Hashimoto, T. Tashiro, M. Sasaki, and H. Takikawa, Biosci. Biotechnol. Biochem., 2007, 71, 2046. CrossRef
33. I. Mori, K. Takai, K. Oshima, and H. Nozaki, Tetrahedron, 1984, 40, 4013. CrossRef
34. (a) E. Sisu, M. Sollogouh, J.-M. Mallet, and P. Sinay, Tetrahedron, 2002, 58, 10189; CrossRef (b) C. Jia, Y. Zhang, and L. Zhang, Tetrahedron: Asymmetry, 2003, 14, 2193. CrossRef
35. A. A. Mahmoud, Planta Medica, 1998, 64, 724. CrossRef
36. B. Pinel, A. Landreau, D. Seraphin, G. Larcher, J.-P. Bouchara, and P. Richomme, J. Enz. Inhibit. & Med. Chem., 2005, 20, 575.
37. (a) K. Fugami, K. Oshima, and K. Utimoto, Tetrahedron Lett., 1987, 28, 809; CrossRef (b) K. Fugami, K. Oshima, and K. Utimoto, Bull. Chem. Soc. Jpn., 1989, 62, 2050. CrossRef
38. (a) S. Itsuno, K. Ito, A. Hirao, and S. Nakahama, J. Chem. Soc., Chem. Commun., 1983, 469; CrossRef (b) E. J. Corey, R. K. Bakshi, and S. Shibata, J. Am. Chem. Soc., 1987, 109, 5551. CrossRef
39. (a) J. C. Gilbert and U. Weerasooriya, J. Org. Chem., 1982, 47, 1837; CrossRef (b) D. Seyferth, R. S. Marmor, and P. Hilbert, J. Org. Chem., 1971, 36, 1379. CrossRef
40. A. A. Devreese, P. J. DeClercq, and M. Vandewalle, Tetrahedron Lett., 1980, 21, 4767. CrossRef
41. J. E. Little, M. W. Foote, and D. B. Johnstone, Arch. Biochem., 1950, 27, 247.
42. (a) T. A. Geissman, P. G. Deuel, E. K. Bonde, and F. A. Addicott, J. Am. Chem. Soc., 1954, 76, 685; CrossRef (b) P. G. Deuel and T. A. Geissman, J. Am. Chem. Soc., 1957, 79, 3778. CrossRef
43. B. Pinel, G. Audo, S. Mallet, M. Lavault, F. D. L. Poype, D. Seraphin, and P. Richomme, J. Chromatogr. A, 2007, 1151, 14. CrossRef
44. H. H. Yoon, H. J. Lim, H. J. Lee, H.-D. Kim, R. Jeon, and J.-H. Ryu, Bioorg. Med. Chem. Lett., 2008, 18, 2179. CrossRef
45. H. Yokoe, M. Yoshida, and K. Shishido, Tetrahedron Lett., 2008, 49, 3504. CrossRef
46. N. Hanryu, T. Aoki, T. Mino, M. Sakamoto, and T. Fujita, Tetrahedron: Asymmetry, 2000, 11, 4127. CrossRef
47. S. F. Martin and J. A. Dodge, Tetrahedron Lett., 1991, 32, 3017. CrossRef
48. P. A. Grieco, S. Gilman, and M. Nishizawa, J. Org. Chem., 1976, 41, 1485. CrossRef
49. P. A. Grieco and M. Miyashita, J. Org. Chem., 1974, 39, 120. CrossRef
50. K. Matsuo, H. Yokoe, K. Shishido, and M. Shindo, Tetrahedron Lett., 2008, 49, 4279. CrossRef