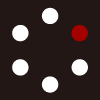
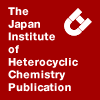
HETEROCYCLES
An International Journal for Reviews and Communications in Heterocyclic ChemistryWeb Edition ISSN: 1881-0942
Published online by The Japan Institute of Heterocyclic Chemistry
e-Journal
Full Text HTML
Received, 6th October, 2008, Accepted, 1st December, 2008, Published online, 8th December, 2008.
DOI: 10.3987/COM-08-S(D)67
■ A Synthetic Approach to Develop Peptide Inhibitors Selective for Brain-Type Sodium Channels on the Basis of Pompilidotoxin Structure
Sawana Yokote, Ritsuko Setoguchi, Eisuke Shimizu, Naoki Mishima, Kohichi Kawahara, Akihiko Kuniyasu, Tetsuya Shirasaki, Kazuo Takahama, Katsuhiro Konno, Nobufumi Kawai, Kaoru Yamaoka, Eiji Kinoshita, and Hitoshi Nakayama*
Faculty of Pharmaceutical Sciences, Kumamoto University, 5-1 Oe-hon-machi, Kumamoto 862-0973, Japan
Abstract
To develop inhibitors that are selective for brain-type sodium channels, several peptides were synthesized on the basis of pompilidotoxin structure. A peptide having N-terminal 7 amino acids and its homologs in which Phe7 is substituted into more hydrophobic amino acids, selectively inhibit sodium current of brain-type sodium channels.INTRODUCTION
In brain ischemia such as occurs in stroke, opening sodium channels (Na+ channels) is a trigger of the subsequent cytotoxic cascade that results in neuronal degeneration or neuronal death. Calcium influx via glutamate receptor(s) is one of the notable events during the cascade, and efforts had been persuaded to develop various antagonists for the receptors in order to cure acute brain ischemia.1 However, the efforts were not successful, mainly due to the fact that most of the developed antagonists showed little selectivity
Hearty dedication to late Dr. John W. Daly, who deceased on March 5, 2008 before celebrating his 75th birthday, and whom we deeply miss.
(see review2) for brain or glutamate receptor subtype that may be involved mainly in brain ischemia. There exist three types of ionotropic glutamate receptors such as NMDA receptor, AMPA receptor, and kainite receptor. Some recent papers report the promising compounds as a certain type of glutamate receptors,3,4 but whether they are selective enough to the glutamate receptors responsible for brain ischemia or they are clinically useful compounds is still under the way. Under such circumstances, we considered that selective inhibitors for brain-type Na+ channels must be more potent agents to protect or prevent from the brain ischemia, since the Na+ channel opening triggers the neuronal death under the ischemic conditions.
α−Pompilidotoxin (α-PMTX) and β-pompilidotoxin (β-PMTX) are unique peptide toxins isolated from solitary wasp venom by Konno et al.5,6 and reviewed.7 Both pompilidotoxins exhibit channel opening activity by slowing the inactivation of Na+ current8 and show highly selectivity toward brain-type Na+ channel,9-11 although they have rather simple chemical structures composed of 13 amino acids without disulfide bonds.5,6 β-PMTX is a homolog of α-PMTX with a single amino acid substitution at position 12 (Lys to Arg), but shows more potent activity than the α-counterpart. Binding site of β-PMTX and its responsible amino acid within the brain-type Na+ channel were revealed by site-directed mutagenesis.12 In addition, useful information is reported on structure-activity relationship of the pompilidotoxin13,14 to explain the channel opener activity.
We consider that peptide analogs that retain selectivity of PMTX to brain-type Na+ channels but act as antagonists, may be developed by modifying or deleting some amino acids of the β-PMTX primary structure. However, little information is available for such anatagonistic activity of PMTX analogs, except the very weak activity of short peptide β-PMTX(1-5) against Na+ current in neuromuscular synapse of lobster walking leg (K. Konnno and N. Kawai, personal communication).
Here we present design and preparation of the peptides that inhibit Na+ currents of brain-type but not of cardiac-type channels.
RESULTS AND DISCUSSION
PMTX analogs we designed and synthesized, are shown in Scheme 1. All the peptides were prepared by Merrifield solid phase synthesis using an automatic peptide synthesizer (PerSeptive Biosystems, Pioneer) according to the manufacture’s protocol based on Fmoc chemistry. After preparative HPLC purification, peptides with proper sequences were obtained in reasonable yields (about 20 % based on 0.1 mmoles of the starting resin used).
1. β-PMTX analogs, some of the constituents are modified by D-amino acids
β-PMTX selectively acts on brain-type Na+ channels but is a channel activator (or “agonist”). To develop channel inhibitors (or “antagonists”), we planed to modify some constituent amino acids of β-PMTX, which are considered to be important for channel activator activity,14 by D-amino acids. The first analog 2, substituted at Arg-12 into D-Arg, revealed that it completely lost β-PMTX activity (channel activation and duration of channel closing) and showed no activity to Na+ current (data not shown). This observation is rather expected, since α-PMTX is namely (Lys-12)-β-PMTX, in which the 12th amino acid Arg of
β-PMTX is substituted by Lys, and shows 5 times less potent than β-PMTX. The second analog, D-β-PMTX 3, in which all the 13 amino acids of β-PMTX are replaced by D-amino acids, showed no activity to Na+ current by itself (Figure 1A). However, an equimolar mixture of 3 and β-PMTX inhibited the effect of β-PMTX on Na+ current (Figure 1B). Both the channel activation and the subsequent duration of channel closing by β-PMTX (curve 2 in Figure 1B) were inhibited about 50 % (curve 3 in Figure 1B), suggesting D- β-PMTX 3 may act as a competitive inhibitor of β-PMTX to the Na+ channel
with similar binding affinity. The third analog 4, in which 6th-13th amino acids of β-PMTX are substituted into the corresponding D-amino acids, gave interesting results. Peptide 4 (100 μM) itself showed channel activation and subsequent duration of channel closing, which is qualitatively similar to β-PMTX but the efficacy is rather small (Figure 2, curves 2 vs 3). An equimolar mixture of 4 and β-PMTX (100 μM each) gave the similar curve to that of β-PMTX alone (curve 4 in Figure 2), which suggests that 4 is a much weaker activator than β-PMTX to the Na+ channel and the effect is not considered to be synergistic between them. It is interesting, however, that 4, in which 8 of the 13 amino acids are substituted into the D-series, still retains some activity of the parent compound. Further analysis is required to explain this interesting but unsolved observation.
2. Shorter peptide analog P7 having N-terminus 7 amino acids of PMTX
Previously, only the synthetic short peptide PMTX(1-5), 5, having N-terminus 5 amino acids of PMTX, exhibited very weak inhibition activity of Na+ current in neuromuscular synapse of lobster walking leg (K. Konno and N. Kawai, personal communication). In mammalian neuroblastoma 2A cells, we also observed that 5 inhibited weakly Na+ current recorded by cell-attached whole cell-clamp method (data not shown). In order to improve the inhibition potency of 5, we synthesized P7, 6, a PMTX analog having N-terminus 7 amino acids. Peptide 6 showed strong inhibition of Na+ channel activation process but no duration in
the channel closing step (Figure 3A), as expected. More than 70 % of the Na+ current was inhibited at 1 mM concentration and IC50 value was 200 μM (Figure 3B). I-V curve showed that addition of 6 did not change its voltage-dependency nor reversal potentials (ca. 50 mV), but only changed the maximal Na+ current (Figure 4). In addition, selective inhibition of 6 to the brain-type Na+ channel was shown in Figure 5. Co-addition of 6 into β-PMTX (100 μM each) inhibited only the activation process of brain-type Na+ current observed in neuroblastoma 2A cells (curve 3 in Figure 5A). By contrast, neither β-PMTX (100 μM) nor 6 (even at 500 μM concentration) showed any effects on cardiac-type Na+ current, whereas ATX-II, a peptide toxin from sea anemone which inhibits the Na+ channel closing process, largely retarded its channel closing (Figure 5B) as reported previously.15,16 Collectively, we succeed in developing an inhibitory peptide that is selective to brain-type Na+ channels.
3. P7 peptide analogs that are modified at Phe7 by more hydrophobic/bulky amino acids
To improve 6 to a better inhibitory peptide, we designed and synthesized P7 analogs that have more hydrophobic and/or bulky amino acid at position 7. This is because P7 having hydrophobic amino acids of Leu and Phe at positions 6 and 7, respectively, showed more efficient inhibition activity than P5. Peptides 7-9 having (p-Bz)-L-Phe, L-1-naphthylalanine, L-2-naphthylalanine, respectively, at peptide position 7 showed more efficient inhibition activity than peptide 6 (Figures 6 and 7). IC50 value of 9, for example, is 50 μM, indicating that 9 is a 4 times more potent inhibitor than 6 (Figure 7A). In addition, I-V curve of 9 also showed it did not change the Na+ channel properties and only inhibited the maximal Na+
current (Figure 7C). It is of note that substitution of L-Phe into L-Tyr at position 7 (peptide 10) caused a marked decrease in inhibition activity (Figures 6D and 7B), suggesting that phenolic hydroxy group may largely disturb the binding environment for the hydrophobic P7 analogs in the brain-type Na+ channels.
In conclusion, we report here the successful development of new peptide inhibitors that show selective to brain-type Na+ channels with reasonably high efficacy. These peptides have simple chemical structures of only 7 amino acids and no disulfide bond, and therefore, they may be applied as agents to protect or prevent from the brain ischemia. However, peptides hardly pass through the blood-brain barrier and are easily hydrolyzed in serum, in general. Further study to convert their structures into peptide mimetic may be necessary to apply them as the therapeutically useful agents.
EXPERIMENTALS
Preparation of peptides
Fmoc-protected amino acids, such as (p-Bz)-L-Phe-OH, L-1-naphthylalanine, L-2-naphthylalanine, and L-Tyr-OH were purchased from Watanabe Chemicals (Hiroshima, Japan). Besides other Fmoc-protected amino acids, Fmoc-Arg(Pbf)-OH and Fmoc-Lys(Boc)-OH (Watanabe Chemicals) were used.
Peptides were synthesized by Automatic Solid-phase Peptide Synthesizer (PerSeptive Biosystems, Pioneer) using Fmoc-PAL-PEG-PS resin, and synthesis was performed according to Fmoc chemistry protocol. Protected peptides were treated with TFA/tri-isopropylsilane for 2 h at rt, followed by precipitation with ice-cold Et2O. The precipitated de-blocked peptides were collected, dried, and purified by reverse-phase HPLC using 0.1 % TFA with increasing MeCN gradient. The purified peptides were confirmed by MALDI TOF-MS and sequence analyses.
Preparation of transfected HEK293 cells expressing cardiac-type Na channel
Plasmid cDNA of cardiac Na+ channel (pcDNA3.1-rHI), provided by Drs. Yamaoka and Kinoshita, was multiplied by its transforming to the competent E. coli (DH5α). After transforming and incubation according to the usual protocol, the transformed E. coli was recovered and further cultured in LB medium containing ampicillin at 37 °C in a CO2 incubator. The plasmid cDNA was then purified using QIAprep Spin Miniprep Kit (QIAGEN) by manufacturer’s protocol, and the purified plasmid cDNA was verified by agarose electrophoresis before and after digestion with ClaI. The multiplied and purified plasmid cDNA was then transfected to the HEK293 cells using SuperFect Transfection Reagent (QIAGEN) in Dulbecco’s modified Eagle’s medium (DMEM). The tranfected HEK293 cells were cultured in DMEM containing 10 % fetal calf serum (FCS).
Cell cultures
Neuroblatoma 2A cells were cultured in RPMI 1640 medium containing 10 % FCS, streptomycin (0.1 mg/ml), and penicillin (100 U/ml) at 37 °C in a 5 % CO2 incubator. The pcDNA3.1-rHI transfected HEK293 cells were maintained in DMEM containing 10 % FCS, streptomycin (0.1 mg/ml), and penicillin (100 U/ml) at 37 °C in a 5 % CO2 incubator.
Electrophysiological recordings
Cells at 80 % confluency were harvested by brief treatment with 0.25 % trypsin. Appropriate numbers of the cells were seeded in culture dishes (diameter 35 mm) and cultured for 1-2 days. For measuring the Na+ current, cell culture medium was replaced by the electrophysiological external solution not containing K+ and Ca2+ (128 mM NaCl, 10 mM MgCl2, 10 mM glucose, and 10 mM HEPES). The pH was adjusted to 7.4 with NaOH. Voltage-clamp recording was carried out with the conventional whole-cell patch clamp technique at rt.17 Briefly, the internal solution contained (in mM) CsCl 140, NaCl 10, EGTA 5 and HEPES 10. The pH was adjusted to 7.2 with CsOH. The patch-pipette was gently placed on the cell surface with a three-axis water hydraulic micromanipulator (MHW-3, Narishige, Tokyo, Japan).
Membrane currents were recorded with a whole cell recording amplifier (TM-1000, Act Me Laboratory, Tokyo, Japan), low-pass filtered at 1 kHz (3611, NF electronic instruments, Yokohama, Japan), and monitored simultaneously on an oscilloscope (CS-412, KENWOOD, Tokyo, Japan) and a thermal head recorder (Omuniace II RA1200,NEC Sanei, Tokyo, Japan). Na+ currents were activated by the brief voltage steps from the holding potential of -100 mV to -10 mV with electro-stimulator (SEN-3301, Nihon Kohden, Tokyo, Japan). Recoded currents were stored on a DAT data recorder (RD-120TE, TEAC, Tokyo, Japan) for data analysis and later acquired on the Windows computer with the Axoscope7 data acquisition software (Molecular Devices, Union city, CA, U.S.A.) after digitization at 5 kHz with a Digidata 1200B (Molecular Devices). Peptides for test were dissolved into the external solution for the Na+ current recording and rapidly applied to the cell by the “Y-tube method”.18
Data analysis was done with Origin 5 (Microcal, Northampton, MA, USA) and/or Excel 2003 (Microsoft, Redmond, WA, USA). Half inhibitory concentration (IC50) was calculated from the fitting of data to the following equation, where, I relative current amplitude, C the peptide concentration and n the Hill coefficient.
ACKNOWLEDGEMENTS
We are grateful to Prof. I. Seyama for his valuable advices. We thank Prof. S. Shoji and Dr. S. Misumi for kind arrangement of TOF-MS measurements. This work was supported by grants-in-aid from the Ministry of Education, Sports, Science and Culture of Japan and CREST.
References
1. R. P. Simon, J. H. Swan, T. Griffith, and B. S. Meldrum, Science, 1984, 226, 850. CrossRef
2. J. C. Watkins and D. E. Jane, Br. J. Pharmacol., 2006, 147, S100. CrossRef
3. L. Leanne, J. M. Sanders, N. Akiyama, M. Shoji, P. Postila, O. T. Pentikaeinen, M. Sasaki, R. Sakai, and T. J. Swanson, J. Pharmacol. Exp. Ther., 2006, 324, 484.
4. Z. Sheng, Q. Dai, M. Porok, and F. J. Castellino, Neuropharmacol., 2007, 53, 145. CrossRef
5. K. Konnno, A. Miwa, H. Terayama, M. Hisada, Y. Itagaki, H. Naoki, T. Yasuhara, and N. Kawai, Neurosc. Lett., 1997, 238, 99. CrossRef
6. K. Konno, M. Hisada, Y. Itagaki, H. Naoki, N. Kawai, A. Miwa, T. Yasuhara, and H. Takayama, Biochem. Biophys. Res. Commun., 1998, 250, 612. CrossRef
7. K. Konno and N. Kawai, Curr. Med. Chem., 2004, 4, 139.
8. Y. Sahara, M. Gotoh, K. Konno, A. Miwa, H. Tsubokawa, H. P. C. Robinson, and N. Kawai, Eur. J. Neurosci., 2000, 12, 1961. CrossRef
9. A. Harsch, K. Konno, H. Takayama, N. Kawai, and Hugh Robinson, Neurosci. Lett., 1998, 252, 49. CrossRef
10. H. Yokoyama, H. Tsubokawa, T. Miyawaki, K. Konno, H. Nakayama, T. Masuzawa, and N. Kawai, Neurosci. Res., 2001, 41, 365. CrossRef
11. T. Miyawaki, H. Tsubokawa, H. Yokota, K. Oguro, K. Konno, T. Masuzawa, and N. Kawai, Neurosci. Lett., 2002, 328, 25. CrossRef
12. E. Kinoshita, H. Maejima, K. Yamaoka, K. Konno, N. Kawai, E. Shimizu, S. Yokote, H. Nakayama, and I. Seyama, Mol. Pharmacol., 2001, 59, 1457.
13. K. Konno, M. Hisada, H. Naoki, Y. Itagaki, T. Yasuhara, Y. Nakata, A. Miwa, and N. Kawai, Neurosci. Lett., 2000, 285, 29. CrossRef
14. N. Kawai and K. Konno, Neurosci. Lett., 2004, 361, 44. CrossRef
15. G. R. Benzinger, J. W. Kyle, K. M. Blumenthal, and D. A. Hanck, J. Biol. Chem., 1988, 273, 80. CrossRef
16. P. K. Khera, G. R. Benzinger, G. Lipkind, C. L. Drum, D. A. Hanck, and K. M. Blumenthal, Biochemistry, 1995, 34, 8533. CrossRef
17. O. P. Hamill, A. Marty, E. Neher, B. Sakmann, and F. J. Sigworth, Pfluegers Arch., 1981, 391, 85. CrossRef
18. K. Murase, P. D. Ryu, and M. Randic, Neurosci. Lett., 1989, 103, 56. CrossRef