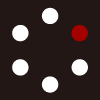
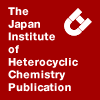
HETEROCYCLES
An International Journal for Reviews and Communications in Heterocyclic ChemistryWeb Edition ISSN: 1881-0942
Published online by The Japan Institute of Heterocyclic Chemistry
e-Journal
Full Text HTML
Received, 7th October, 2008, Accepted, 27th November, 2008, Published online, 9th December, 2008.
DOI: 10.3987/COM-08-S(D)68
■ Formal Synthesis of (5R,8R,8aS)-Indolizidine 209I via Enaminones Incorporating Weinreb Amides
Charles B. de Koning, Joseph P. Michael,* and Darren L. Riley
School of Chemistry, University of the Witwatersrand, Private Bag 3, PO WITS
2050, South Africa
Abstract
A formal enantioselective synthesis of the amphibian alkaloid (5R,8R,8aS)-(–)-indolizidine 209I (6) is reported. Control of the absolute stereochemistry at C-5 resulted from application of the Davies procedure, which entails stereoselective conjugate addition of (R)-(+)-N-benzyl-1-phenylethylamine to tert-butyl (E)-hex-2-enoate. The resulting chiral adduct 26 was converted in eight steps into a pivotal enaminone incorporating a Weinreb amide, the inherent nucleophilicity of which was exploited in a cyclisation that yielded the key bicyclic intermediate (5R)-N-methoxy- N-methyl-5-propyl-1,2,3,5,6,7-hexahydroindolizine- 8-carboxamide (38). Stereoselective catalytic hydrogenation of the alkene bond, reaction of the Weinreb amide with ethylmagnesium bromide, and epimerisation of the resulting ketone completed the formal synthesis of the target alkaloid.INTRODUCTION
The indolizidine (1-azabicyclo[4.3.0]nonane) motif is well represented among the alkaloids isolated from the skins of amphibians.1,2 This family of metabolites, most of which appear to be sequestered from insects upon which the animals feed, comprises several distinct classes, including 3,5- and 5,8-disubstituted indolizidines, for example, (–)-indolizidines 223AB (1) and 209B (2); 5,6,8-trisubstituted indolizidines such as (–)-indolizidine 223A (3); and pumiliotoxins and allopumiliotoxins such as (+)-pumiliotoxin 251D (4) and its hydroxy congener, allopumiliotoxin 267A (5) (Figure 1). The 5,8-disubstituted indolizidines are particularly abundant, and about 80 members of this class have been partially or fully characterised to date.2 These intriguing natural products, and especially those bearing a methyl substituent at C-8, have become popular targets for total synthesis.3,4 More recently identified members of the series contain ethyl, propyl, butyl, vinyl or but-3-enyl substituents at C-8, while some also bear hydroxylated alkyl substituents at this site. The only examples of these longer-chain homologues to have been synthesised are indolizidines (–)-209I (6),5,6 (–)-219F (7),7,8 (–)-221I (8),7,9 (–)-221K (9),10 (–)-223J (10),5 (–)-223V (11)11,12 and (–)-251N (12),10 as well as racemic 209I and 223J.13
In continuing investigations into the use of pyrrolidinylidene- and piperidinylidene-containing enaminones as key intermediates in the synthesis of alkaloids and other nitrogen heterocycles,14 we previously reported enantioselective total syntheses of (–)-indolizidine 209B (2)15 and a monosubstituted analogue, (–)-indolizidine 167B (13),16,17 via chiral 3-amino esters prepared by the well-known Davies protocol.18,19 In this article we present a formal synthesis of (–)-indolizidine 209I (6) by a route that expands upon features introduced in our syntheses of 2 and 13.
RESULTS AND DISCUSSION
Steps in our prior enantioselective synthesis of (–)-indolizidine 209B (2)15 that are relevant to the present report are shown in Scheme 1. The homochiral amine (–)-14, prepared by the Davies procedure from tert-butyl (E)-oct-2-enoate and (R)-N-benzyl-1-phenylethylamine followed by hydrogenolytic removal of the benzyl substituents, was converted in several steps into the thiolactam (+)-15. Eschenmoser sulfide contraction20,21 with ethyl bromoacetate yielded the enaminone (vinylogous urethane) intermediate (+)-16, after which chemoselective reduction of the saturated ester produced the alcohol (–)-17. The bicyclic core of the alkaloid was then constructed by a cycloalkylation that took advantage of the nucleophilic reactivity of the enaminone. The synthesis was completed by chemoselective and reasonably diastereoselective reduction of the alkene bond of the bicyclic enaminone (+)-18, catalytic hydrogenation to (–)-19, and epimerisation of the ester group to give (–)-20. The ester finally served as the source of the methyl substituent at C-8.
A similar route can be envisaged for the synthesis of indolizidine 209I and other analogues, but in this case homologation of the C-8 substituent would be required in order to introduce the longer chains. In preliminary experiments, attempts to replace the ester group of the vinylogous urethane by appropriate ketones gave disappointing results. As an alternative, we turned to Weinreb amides, which have become standard intermediates for the production of ketones by virtue of their ready reaction with organometallic reagents.22 However, we are aware of only three prior examples23-25 of enaminones containing Weinreb amides (effectively, vinylogous ureas). Since the reactivity of such rare systems could not be predicted with confidence, we first investigated a simple model system to ascertain the feasibility of working with this type of compound.
The simple vinylogous urea 22 was readily prepared in 85% yield by alkylating 3-(2-thioxo-1-pyrrolidinyl)propyl acetate (21), a known compound,26 with N-methoxy-N-methyl- 2-bromoacetamide,24 after which extrusion of sulfur was effected by treatment with triphenylphosphine and triethylamine in acetonitrile at ambient temperature (Scheme 2). The acetate was cleaved with potassium carbonate in methanol to give the alcohol 23 in 83% yield. The (E)-geometry of the double bond in these products was inferred from the chemical shift of the hydrogen atoms at C-3 in the ring (δ ca. 3.2), the downfield shift of about 0.6 ppm relative to (Z)-analogues20 arising from the anisotropic deshielding effect of the carbonyl group. In situ conversion of the free alcohol into the corresponding iodide with iodine, triphenylphosphine and imidazole in a mixture of toluene and acetonitrile27 and heating the reaction mixture under reflux produced the desired but hard-to-purify bicyclic product, 24, in a moderate 64% yield. Unfortunately, this compound failed to undergo the typical alkylation reaction of Weinreb amides when treated with a variety of organometallic reagents, including methyllithium, n-butyllithium, ethylmagnesium bromide and allylmagnesium bromide; in all cases, only unreacted 24 was recovered. This disappointing result was in line with the only previously reported attempt to alkylate Weinreb amides incorporated into an enaminone backbone with organometallics.24 However, catalytic hydrogenation of 24 over Adams catalyst in glacial acetic acid produced the saturated compound 25 predominantly as one diastereomer (95 : 5, by NMR), although the overall yield based on alcohol 23 was only 25%. Nonetheless, these results served to establish proof of concept for the synthesis of indolizidine 209I.
We next embarked on the synthesis of indolizidine 209I by modifying the strategy shown in Scheme 1. The procedure commenced with (R)-(+)-26, the homochiral adduct from the Davies reaction of tert-butyl (E)-hex-2-enoate and (R)-N-benzyl-1-phenylethylamine.17 The sequence shown in Scheme 3 (upper line) proceeded smoothly up to the formation of thiolactam (+)-27, an intermediate that featured in our prior synthesis of (–)-indolizidine 167B.17 Alkylation on sulfur with N-methoxy-N-methyl-2-bromoacetamide followed by Eschenmoser reaction afforded the unstable vinylogous urea 28 in 77% yield. However, all attempts to reduce the tert-butyl ester selectively with lithium aluminium hydride under various conditions were largely unsuccessful, the best yield of the desired alcohol (+)-29 being 11%. Over-reduction of the vinylogous urea appears to be a serious competitor, in contrast to the relative robustness of analogous vinylogous urethanes. This setback, not entirely unexpected, necessitated a change of tactics.
Our prior experience with another very labile enaminone28 suggested that the problem could be resolved by reduction of the saturated ester at a much earlier stage of the synthesis. In this case, the Davies adduct 26 was reduced with lithium aluminium hydride in 97% yield to give the alcohol (–)-30, which was protected as the tert-butyl(dimethylsilyl) ether (+)-31 (Scheme 3, lower line). Hydrogenolytic removal of the benzyl groups was achieved by treatment with palladium on carbon under a moderate pressure of hydrogen (7 atmospheres) to give the primary amine (+)-32, which was immediately acylated with 4-chlorobutanoyl chloride. The resulting unstable amide underwent cyclisation to the lactam (+)-33 on careful treatment with potassium tert-butoxide in dry tert-butyl alcohol. However, since the silyl ether subsequently did not survive attempted thionation of 33 under a variety of conditions – a problem that we had previously encountered in a related system28 – yet another change of plans was required.
The solution, undoubtedly clumsy, required a change of protecting groups for the alcohol (Scheme 4). The silyl ether 33 was cleaved with aqueous hydrofluoric acid, after which the liberated alcohol (–)-34 was acetylated to give (+)-35 in an overall yield of 76% based on 33. The lactam was then successfully thionated with phosphorus pentasulfide by the Brillon procedure,29 giving (+)-36 in 91% yield. Salt formation with N-methoxy-N-methyl-2-bromoacetamide followed by sulfide contraction afforded the vinylogous urea intermediate 37, slightly contaminated with phosphorus-containing impurities that were difficult to remove. However, on hydrolysis of the acetate with potassium carbonate in methanol, the pure alcohol (+)-29 could be isolated in an overall yield of 64% based on 36. This product was identical in all respects with that prepared by the abortive route shown in Scheme 3.
The stage was now set for the construction of the indolizidine nucleus. In situ conversion of the free alcohol 29 into the corresponding iodide and heating the reaction mixture under reflux produced the desired bicyclic product, (5R)-N-methoxy-N-methyl-5-propyl-1,2,3,5,6,7-hexahydroindolizine-8- carboxamide 38, in a somewhat disappointing yield of 47% (Scheme 5). However, hydrogenation over platinum dioxide was stereoselective, and gave the expected cis-hydrogenated product (–)-39 as the only isolable isomer in 80% yield. The diastereofacial selectivity is in accord with what we have previously observed with related bicyclic vinylogous urethanes in both indolizidine15 and quinolizidine28 systems. In line with our expectations, there appears to be an equatorial preference for the propyl side chain in the developing chair conformation of the six-membered ring, and this in turn directs the reductant towards the less hindered distal face of the double bond. Support for the cis-relationship of the hydrogen atoms at C-5 and C-8a in the product was provided by a Bohlmann band30 at ca. 2790 cm–1 in the FTIR spectrum, a feature that also implies a trans-disposition of the lone pair and 8a-H across the ring junction. At this point we took advantage of the characteristic reactivity of the Weinreb amide. Treating 39 with ethylmagnesium bromide in tetrahydrofuran followed by hydrolysis of the adduct with dilute hydrochloric acid furnished the 8-propanoylindolizidine (+)-40 in 83% yield, without apparent epimerisation at C-8. Of course, the target alkaloid requires inversion of configuration at this site. This inversion was readily achieved by heating 40 with sodium methoxide in methanol at reflux. After workup and purification, an 80% recovery of the epimerised ketone (–)-41 was obtained. This product has previously been reported by Ma and co-workers6 as an intermediate in their synthesis of (–)-indolizidine 209I (6). Our product gave nuclear magnetic resonance spectra that agreed with those reported by Ma to within 0.03 and 0.3 delta units for the 1H and 13C signals, respectively. Our synthesis thus constitutes a formal synthesis of (–)-indolizidine 209I, since Ma converted 41 into the target alkaloid in 53% overall yield by treatment of the corresponding dithioacetal (–)-42 with Raney nickel.
Our formal route to (–)-indolizidine 209I (6) is, in principle, suitable for making most of the known members of the 5,8-disubstituted indolizidine family of alkaloids. The Davies procedure for preparing chiral 3-amino esters is versatile enough to permit introduction of many of the substituents found at C-5 simply by commencing with appropriate (E)-enoate esters at the outset. More importantly, we have shown that enaminones that incorporate a Weinreb amide unit provide a useful extension to our general approach to alkaloid synthesis; by treating late-stage intermediates such as 39 with organometallic reagents of different chain lengths, most of the substituents found at C-8 in the natural products should be accessible.
EXPERIMENTAL
Tetrahydrofuran (THF) was distilled from Na/benzophenone; acetonitrile, N,N-dimethylformamide (DMF), dichloromethane and triethylamine from CaH2; pyridine from potassium hydroxide; and toluene from Na metal. Commercially available chemicals were used as received. Melting points, recorded on a Reichert hot-stage microscope apparatus, are uncorrected. TLC was performed on aluminium-backed Alugram Sil G/UV254 plates pre-coated with 0.25 mm silica gel 60. Column chromatography was carried out on silica gel 60, particle size 0.063–0.200 mm (conventional columns) or Whatman Partisil Prep 40, particle size 0.040–0.063 mm (flash columns). FTIR spectra were recorded on a Bruker Vector 22 spectrometer. NMR spectra were recorded on a Bruker Avance 300 spectrometer (300.139 MHz for 1H, 75.035 MHz for 13C) in CDCl3 as solvent and with TMS as internal standard. DEPT and CH-correlated spectra were routinely used for assignment of signals. J values are given in Hz. High-resolution mass spectra were recorded at 70 eV on a VG 70 SEQ mass spectrometer at 70 eV and 200 μA with a MASPEC II data system. Optical rotations were measured on a Jasco DIP-370 polarimeter; [α]D values are given in 10–1 deg cm2 g–1. Precursors 26 and 27 were prepared as described previously.17
3-[(2E)-2-{2-[Methoxy(methyl)amino]-2-oxoethylidene}pyrrolidin-1-yl]propyl acetate (22)
3-(2-Thioxo-1-pyrrolidinyl)propyl acetate26 (21) (6.73 g, 33.5 mmol) and 2-bromo-N-methoxy- N-methylacetamide (6.39 g, 35.1 mmol) were stirred together in dry CH2Cl2 (67 cm3) for 5 h. The solvent was removed under high vacuum and the resulting slurry was stirred at rt for a further 18 h. The salt was dissolved in MeCN (60 cm3) and a homogeneous solution of PPh3 (9.21 g, 35.1 mmol) and NEt3 (3.55 g, 4.90 cm3, 35.1 mmol) in MeCN (60 cm3) was added in one portion. After 5 h the solution was filtered through a pad of celite and evaporated in vacuo. The residue was taken up in EtOAc (300 cm3), triturated for 30 min and again filtered through a pad of celite. The filtrate was extracted with HCl (2 M, 3 × 300 cm3), the aqueous extracts were basified to pH 11 with conc. aq. NH3 solution (35%) and back-extracted with CH2Cl2 (3 × 100 cm3). The organic extracts were combined, dried (anhydrous MgSO4), filtered and evaporated in vacuo. Purification by column chromatography with CH2Cl2-MeOH (9 : 1) as eluent afforded 3-[(2E)-2-{2-[methoxy(methyl)amino]-2-oxoethylidene}pyrrolidin-1-yl]propyl acetate (22) as a light yellow oil (7.73 g, 85%); Rf (EtOAc–MeOH 4 : 1) 0.81; vmax (film)/cm−1 2939 (C-H, m), 1734 (ester C=O, s), 1646 (amide C=O, s), 1426 (m), 1367 (m) 1233 (s), 1042 (s), 998 (m); δH (300 MHz; CDCl3) 5.10 (1H, s, C=CH), 4.11 (2H, t, J = 6.3 Hz, CH2OAc), 3.67 (3H, s, OMe), 3.36 and 3.31 (4H, 2 × overlapping t, J = 7.1 Hz, J = 7.3 Hz, ring and chain CH2N), 3.21 (2H, t, J = 7.7 Hz, CH2C=), 3.15 (3H, s, NMe), 2.07 (3H, s, OCOMe), 1.94 (4H, coincident quintets, J = 6.8 Hz, remaining CH2); δC (75 MHz; CDCl3) 171.8, 170.8, 164.3, 76.7, 61.9, 60.8, 52.4, 43.1, 33.0, 32.6 , 25.4, 21.2, 20.8; m/z (EI) 270 (M+, 1%), 211 (13), 210 (100), 168 (13), 148 (12), 74 (22). HRMS (EI) Found: M+, 270.1562. C13H22N2O4 requires 270.1580.
(2E)-2-[1-(3-Hydroxypropyl)pyrrolidin-2-ylidene]-N-methoxy-N-methylethanamide (23)
3-[(2E)-2-{2-[Methoxy(methyl)amino]-2-oxoethylidene}pyrrolidinyl]propyl acetate (22) (7.73 g, 28.6 mmol) and K2CO3 (7.91 g, 57.2 mmol) were stirred in MeOH (100 cm3) for 3 h. The mixture was filtered through celite. The filtrate was evaporate in vacuo, and then taken up in CHCl3 (300 cm3) and washed with a saturated sodium chloride solution (300 cm3). The aqueous phases were back extracted with CHCl3 (3 × 250 cm3), dried (anhydrous MgSO4), filtered and evaporated in vacuo to afford the crude product. Purification by column chromatography with CH2Cl2-MeOH (9 : 1) as eluent gave (2E)-2-[1-(3-hydroxypropyl)pyrrolidin-2-ylidene]-N-methoxy-N-methylethanamide (23) (5.45 g, 83%) as a yellow oil; Rf 0.85 (CH2Cl2-MeOH 7 : 3); vmax (film)/cm−1 3353 (O-H, v br, m), 2938 and 2874 (C-H, m) 1646 (s), 1613 (s), 1438 (m), 1423 (m), 1360 (m), 1170 (m), 1055 (m), 918 (m), 828 (m), 810 (m), 720 (m), 660 (m); δH (300 MHz; CDCl3) 5.14 (1H, s, C=CH), 3.68 and 3.67 (5H, overlapping t and s, J = 6.0 Hz, CH2OH and OMe), 3.38 and 3.36 (4H, 2 × overlapping t, J = 7.0 Hz, 6.9 Hz, ring and chain CH2N), 3.22 (2H, t, J = 7.8 Hz, CH2C=), 3.14 (3H, s, NMe), 2.04 (1H, s, OH), 1.93 (2H, quintet, J = 7.1 Hz, chain CH2CH2CH2), 1.84 (2H, quintet, J = 6.6 Hz, ring 4-H); δC (75 MHz; CDCl3) 172.1, 164.8, 76.3, 60.9, 59.9, 52.4, 42.9, 33.1, 32.7, 29.1, 21.2; m/z (EI) 228 (M+, 2%), 169 (12), 168 (100), 150 (5), 120 (5) 110 (5), 108 (5). HRMS (EI) Found, M+, 228.1467. C11H20N2O3 requires 228.1474.
N-Methoxy-N-methyl-1,2,3,5,6,7-hexahydroindolizine-8-carboxamide (24)
A solution of (2E)-2-[1-(3-hydroxypropyl)pyrrolidin-2-ylidene]-N-methoxy-N-methylethanamide (23) (0.776 g, 2.79 mmol) was dissolved in a mixture of MeCN (17 cm3) and PhMe (8.5 cm3). To this was added PPh3 (1.46 g, 5.58 mmol) and imidazole (0.380 g, 5.58 mmol) followed by I2 (1.42 g, 5.58 mmol). The solution was heated at reflux for 1 h. The reaction was quenched by the addition of a saturated solution of aq. NaHCO3 (30 cm3), and the aqueous residues were extracted with EtOAc (3 × 30 cm3). The combined organic fractions were washed with saturated aq. Na2S2O3 solution (30 cm3). The organic washings were dried (anhydrous MgSO4), filtered and evaporated in vacuo to yield the crude product. Purification by flash column chromatography with EtOAc-EtOH (19 : 1) as eluent yielded N-methoxy-N-methyl-1,2,3,5,6,7- hexahydroindolizine-8-carboxamide (24) (0.375 g, 64%); Rf (CH2Cl2-MeOH 19 : 1) 0.19; δH (300 MHz; CDCl3) 3.62 (3H, s, OMe), 3.26 (2H, t, J = 7.0 Hz, 3-H or 5-H), 3.18 (2H, t, J = 5.8 Hz, 3-H or 5-H), 3.06 (3H, s, NMe), 3.01 (2H, t, J = 7.8 Hz, 1-H), 2.38 (2H, t, J = 6.0 Hz, 7-H), 1.90 and 1.83 (4H, 2 × overlapping quintet, J = 5.8 Hz, J = 5.8 Hz, 2-H and 6-H); δC (75 MHz; CDCl3) 174.4, 157.7, 90.0, 59.7, 52.6, 45.0, 34.3, 31.7, 23.6, 21.9, 21.2. HRMS (EI) Found: M+, 210.1352. C11H18N2O2 requires 210.1368.
rel-(8R,8aS)-N-Methoxy-N-methyloctahydroindolizine-8-carboxamide (25)
A solution of bicyclic vinylogous urea 24 (0.173 g, 0.823 mmol) in glacial acetic acid (4.5 cm3) containing a suspension of Adams’ catalyst (41 mg) was hydrogenated for 24 h at 1 atmosphere. The mixture was filtered through celite and washed copiously with EtOH, after which the filtrates were evaporated in vacuo. Purification of the residue by column chromatography with CH2Cl2-MeOH (19 : 1) as eluent afforded rel-(8R,8aS)-N-methoxy-N-methyloctahydroindolizine-8-carboxamide (25) and an inseparable diastereomer (95 : 5) as a yellow oil (43 mg, 25%); vmax (film)/cm−1 2928 (C-H, s), 1663 (C=O, s), 1441 (m), 1378 (m), 1342 (m), 1160 (m), 1100 (m), 1039 (w), 998 (s), 963 (m); δH (300 MHz; CDCl3; major isomer) 3.67 (3H, s, OMe), 3.26-3.20 (1H, M, 3-H eq), 3.18 (3H, s, NMe), 3.08-2.94 (2H, m, 8-H and 8a-H), 2.38-2.31 (1H, m), 2.26-1.98 (3H, m), 1.95-1.43 (7H, m); δC (75 MHz; CDCl3) 174.7 (C=O), 63.5 (C-8a), 61.2 (OMe), 54.4 (C-3), 51.8 (C-5), 37.0 (C-8), 29.6 (NMe), 26.1 (C-1), 25.2 (C-7), 22.8 (C-2), 20.4 (C-6). HRMS (EI) Found: M+, 212.1518. C11H20N2O2 requires 212.1525.
tert-Butyl (3R)-3-[(2E)-2-{2-[methoxy(methyl)amino]-2-oxoethylidene}pyrrolidin-1-yl]hexanoate (28)
tert-Butyl (3R)-3-(2-thioxopyrrolidin-1-yl)hexanoate17 (27) (0.530 g, 1.95 mmol) and 2-bromo-N-methoxy-N-methylacetamide24 (0.430 g, 2.34 mmol) were dissolved in dry MeCN (4.00 cm3, 2.00 cm3 mmol−1). The mixture was stirred for 16 h at rt, after which time the solvent was removed in vacuo to afford a white salt. This was re-dissolved in dry MeCN (4.00 cm3), and to this was added PPh3 (0.614 g, 2.34 mmol) followed by NEt3 (0.237 g, 0.330 cm3, 2.34 mmol). The solution was stirred for 3 h, during which time a white precipitate formed. The solution was filtered through a celite pad, the solvent was removed in vacuo and the resulting residue was triturated in EtOAc for 30 min. The solution was again filtered through celite, and the filtrate was then extracted with aq. HCl (2.0 M, 3 × 50 cm3). The aqueous extracts were basified to ~ pH 10 with aq. NH3, and then extracted with CH2Cl2 (3 × 50 cm3). The combined organic extracts were dried (anhydrous MgSO4), filtered and evaporated in vacuo to yield a yellow oil. The crude oil was purified by column chromatography with MeOH-CH2Cl2 (1 : 19) as eluent to afford tert-butyl (3R)-3-[(2E)-2-{2-[methoxy(methyl)amino]-2-oxoethylidene}-pyrrolidin-1-yl]-hexanoate (28) as a light yellow oil (0.509 g, 77%). vmax (film)/cm−1 3084, 3062 and 3027 (=C-H, w), 2970, 2932 and 2872 (C-H, w), 1724 (C=O, s), 1493 (m), 1454 (m), 1367 (s), 1297 (m), 1230 (m), 1217 (m), 1143 (s), 1094 (m), 1026 (w), 749 (m), 698 (s); δH (300 MHz; CDCl3) 5.26 (1H, s, C=CH), 4.17-4.07 (1H, m, NCH), 3.68 (3H, s, OMe), 3.32-3.19 (4H, m, ring NCH2 and CH2C=), 3.14 (3H, s, NMe), 2.44 (2H, dd, J = 6.0 and 7.1 Hz, NCHCH2CO), 1.88 (2H, quintet, J = 7.3 Hz, ring CH2CH2CH2), 1.67-1.45 (2H, m, NCHCH2Et), 1.41 (9H, s, CMe3), 1.36-1.20 (2H, m, CH2Me), 0.93 (3H, t, J = 7.3 Hz, CH2Me); δC (75 MHz; CDCl3) 172.1, 170.1, 164.7, 80.8, 77.7, 60.8, 51.3, 45.6, 39.0, 34.3, 33.0, 32.6, 27.8, 21.1, 19.3, 13.7.
(2E)-2-{1-[(1R)-1-(2-Hydroxyethyl)butyl]pyrrolidin-2-ylidene}-N-methoxy-N-methylethanamide (29)
tert-Butyl (3R)-3-[(2E)-2-{2-[methoxy(methyl)amino]-2-oxoethylidene}pyrrolidin-1-yl]hexanoate (28) (0.167 g, 0.490 mmol) was added to a slurry of LiAlH4 (0.022 g, 0.590 mmol) in Et2O (1.00 cm3) at 0 °C. The slurry was warmed to rt and stirred for 16 h. The reaction was quenched by the sequential addition of H2O (0.1 cm3), aq. NaOH solution (0.1 cm3, 15% w/v) and finally H2O (0.2 cm3). The solids were filtered off by passing the mixture through a thin pad of celite and washing several times with CH2Cl2. The filtrate was dried (anhydrous Na2SO4), filtered and evaporated in vacuo to yield a brown-orange oil. Purification of the oil by column chromatography with EtOH-CH2Cl2 (1 : 19) as eluent afforded (2E)-2-{1-[(1R)-1-(2-hydroxyethyl)butyl]pyrrolidin-2-ylidene}-N-methoxy-N-methylethanamide (29) as a clear oil (0.0150 g, 11%). Rf 0.59 (EtOH-CH2Cl2 1 : 9); [α]D20 +3.85 (c 1.04 abs. EtOH); vmax (film)/cm−1 3358 (OH, v br, m), 2955, 2931 and 2870 (C-H, m), 1617 (C=O, m), 1553 (s), 1411 (m), 1279 (m), 1238 (m); δH (300 MHz; CDCl3) 5.25 (1H, s, NC=CH), 3.91 (1H, br quintet, J = ca 7.2 Hz, NCH), 3.66 and 3.60-3.50 (4H, overlapping s and m, OMe and CHaHbOH), 3.50-3.35 (1H, m, CHaHbOH), 3.26 (2H, t, J = 7.9 Hz, ring NCH2), 3.19 (2H, dt, J = 6.6 and 2.7 Hz, ring CH2C=), 3.14 (3H, s, NMe), 1.90 (2H, quintet, J = 7.3 Hz, ring CH2CH2CH2), 1,80-1.60 (3H, m, CH2 and OH), 1.60-1.20 (4H, m), 0.92 (3H, t, J = 7.3 Hz, CH2Me); δC (75 MHz; CDCl3) 172.4, 165.9, 76.4, 60.9, 59.4, 50.6 , 45.3, 35.0, 34.8, 33.1, 32.9, 21.2, 19.5, 13.9.
(3R)-3-{Benzyl[(1R)-1-phenylethyl]amino}hexan-1-ol (30)
LiAlH4 (1.36 g, 35.8 mmol) was added to a stirred solution of tert-butyl (3R)-3-((2E)-2-{2-[methoxy(methyl)amino]-2-oxoethylidene}pyrrolidinyl)hexanoate17 (26) (12.4 g, 32.6 mmol) in Et2O (65.0 cm3) at 0 °C. The mixture was warmed to rt and stirred for 16 h. The reaction was quenched by the sequential addition of H2O (7.2 cm3), aq. NaOH solution (7.2 cm3, 15% w/v) and finally H2O (21.7 cm3). The solids were removed by passing the mixture through a thin celite pad. The filtrate was dried (anhydrous Na2SO4), filtered and evaporated in vacuo to yield a light yellow oil. The solids and celite were recovered and dried in a desiccator, once dry they were ground to a fine powder. The powder was stirred in CH2Cl2 (100 cm3), filtered and evaporated in vacuo to afford more of the light yellow oil. The crude oils were combined and purified by column chromatography with hexane-EtOAc (4 : 1) as eluent to give (3R)-3-{benzyl[(1R)-1-phenylethyl]amino}hexan-1-ol (30) (9.84 g, 97%) as a clear oil. Rf 0.82 (hexane-EtOAc 1 : 1); [α]D19 −32.1 (c 1.09, CHCl3); vmax (film)/cm−1 3367 (OH, v br, w), 3084, 3062 and 3027 (ArC-H, w), 2956, 2931 and 2870 (C-H, m), 1602 (w), 1493 (m), 1452 (m), 1373 (m), 1204 (w), 1140 (w), 1052 (m), 1027 (m), 905 (w), 744 (s), 697 (s); δH (300 MHz; CDCl3) 7.40-7.19 (10H, m, Ar-H), 3.95 (1H, q, J = 6.9 Hz, NCHPh), 3.84 (1H, d, J = 13.7 Hz, NCHaHbPh), 3.68 (1H, d, J = 13.7 Hz, NCHaHbPh), 3.52-3.45 (1H, m, CHaHbOH), 3.24-3.17 (1H, m, CHaHbOH), 2.83-2.76 (1H, m, NCHCH2Et), 2.64 (1H, br s, OH), 1.74-1.49 (2H, m, NCH2CH2OH), 1.45-1.23 and 1.39 (7H, overlapping m and d, J = 6.9 Hz, CH2CH2Me and PhCHMe), 0.93 (3H, t, J = 7.1 Hz, CH2Me); δC (75 MHz; CDCl3) 143.9, 140.8, 129.0, 128.3, 128.1, 128.0, 126.9 (2 signals), 61.8, 56.7, 54.8, 49.9, 34.9, 33.7, 20.8, 15.1, 14.4. HRMS (EI) Found, M+, 311.2253. C21H29NO requires 311.2249.
(3R)-N-Benzyl-1-{[tert-butyl(dimethyl)silyl]oxy}-N-[(1R)-1-phenylethyl]hexan-3-amine (31)
tert-Butyldimethylsilyl chloride (5.04 g, 33.1 mmol) in DMF (18.0 cm3) was added dropwise to a stirred solution of (3R)-3-{benzyl[(1R)-1-phenylethyl]amino}hexan-1-ol (30) (9.37 g, 30.1 mmol) and imidazole (4.11 g, 60.1 mmol) in DMF (36.0 cm3). The mixture was then stirred for 24 h. The reaction mixture was washed with ice/water (180 cm3), and the aqueous residues were extracted with CH2Cl2 (5 × 180 cm3). The combined organic residues were dried (anhydrous Na2SO4), filtered and evaporated in vacuo. The residue was re-dissolved in CH2Cl2 (180 cm3) and washed with H2O (4 × 180 cm3). The organic extract was dried (anhydrous Na2SO4), filtered and evaporated in vacuo to yield a crude yellow oil. Purification by column chromatography with hexane-EtOAc (9 : 1) as eluent afforded (3R)-N-benzyl-1-{[tert-butyl- (dimethyl)silyl]oxy}-N-[(1R)-1-phenylethyl]hexan-3-amine (31) (11.2 g, 88%), as a clear oil. Rf 0.72 (hexane-EtOAc 9 : 1); [α]D20 +18.9 (c 1.27, CHCl3); vmax (film)/cm−1; 3085, 3063 and 3028 (ArC-H, w), 2955, 2929 and 2857 (C-H, m), 1602 (w), 1493 (m), 1454 (m), 1373 (m), 1362 (m), 1253 (s), 1205 (w), 1144 (m), 1089 (s), 1027 (m), 1005 (m), 980 (m), 938 (m), 834 (s), 774 (s), 747 (s), 697 (s), 663 (m); δH (300 MHz; CDCl3) 7.40-7.16 (10H, m, Ar-H), 3.87 (1H, q, J = 6.9 Hz, NCHPh), 3.78 (1H, d, J = 14.9 Hz, NCHaHbPh), 3.64 (1H, d, J = 14.8 Hz, NCHaHbPh), 3.46 (1H, m, CHaHbOSi), 3.27 (1H, m, CHaHbOSi), 2.68 (1H, quintet, J = 6.1 Hz, NCHCH2Et), 1.63-1.41 (2H, m, NCH2CH2OSi), 1.38-1.20 and 1.29 (7H, overlapping m and d, J = 6.9 Hz, CH2CH2Me and PhCHMe), 0.89-0.82 and 0.85 (12H, overlapping m and s, CH2Me and SiCMe3), −0.02 (6H, s, SiMe2); δC (75 MHz; CDCl3) 144.9, 142.7, 128.3, 128.1, 128.0, 127.9, 126.6, 126.3, 61.9, 58.1, 53.8, 50.2, 35.3, 34.5, 26.0, 20.5, 18.9, 18.3, 14.3, −5.3. HRMS (EI) Found, M+, 425.3097. C27H43NOSi requires 425.3114.
(3R)-1-{[tert-Butyl(dimethyl)silyl]oxy}hexan-3-amine (32)
10% Palladium on carbon (3.97 g), was added to a mixture of (3R)-N-benzyl-1- {[tert-butyl(dimethyl)silyl]oxy}-N-[(1S)-1-phenylethyl]hexan-3-amine (31) (11.1 g, 26.1 mmol) in absolute EtOH (104 cm3). The stirred mixture was hydrogenated at 7 atm for 3 d at ambient temperature. The mixture was then filtered through celite and washed copiously with absolute EtOH, after which the combined organic phases were evaporated in vacuo to yield a grey oil. The oil was purified by column chromatography with EtOAc as the eluent to afford (3R)-1-{[tert-butyl(dimethyl)silyl]oxy}hexan-3-amine (32) (5.11 g, 85%) as a clear oil. The unstable product was used immediately in the next reaction after cursory characterisation. Rf 0.38 (EtOAc); [α]D21 +1.43 (c 0.70, CHCl3); vmax (film)/cm−1 3377 (N-H, br, s), 2935 and 2871 (C-H, s), 1737 (w), 1615 (m), 1550 (s), 1460 (m), 1386 (m), 1310 (m), 1170 (s), 1099 (s), 1053 (s), 994 (s); δH (300 MHz; CDCl3) 3.77-3.67 (2H, m, CH2OSi), 2.90-2.84 (1H, m, NCH), 1.67-1.23 and 1.50 (8H, overlapping m and br s, 3 × CH2 and NH2), 0.89 and 0.87 (12H, overlapping t and s, J = 6.5 Hz, CH2Me and SiCMe3), 0.03 (6H, s, SiMe2).
1-[(1R)-1-(2-{[tert-Butyl(dimethyl)silyl]oxy}ethyl)butyl]pyrrolidin-2-one (33)
(a) 4-Chlorobutanoyl chloride (1.95 g, 1.10 cm3, 13.8 mmol, 1.2 eq.) was added dropwise to a solution of (3R)-1-{[tert-butyl(dimethyl)silyl]oxy}hexan-3-amine (32) (2.67 g, 11.5 mmol) and NEt3 (2.91 g, 4.00 cm3, 28.8 mmol) in dry CH2Cl2 (46.0 cm3), causing a vigorous evolution of hydrogen chloride gas. The mixture was stirred for 30 min, after which time the reaction was quenched with H2O (50 cm3) and evaporated in vacuo. The residue was dissolved in CH2Cl2 (50 cm3) and washed with H2O (50 cm3) and brine (50 cm3). The aqueous extracts were back-extracted with CH2Cl2 (3 × 50 cm3). The organic extracts were combined, dried (anhydrous Na2SO4), filtered and evaporated in vacuo to yield an orange oil. The crude oil was purified by column chromatography with hexane-EtOAc (1 : 1) as eluent to yield the unstable (1R)-1-(2-{[tert-butyl(dimethyl)silyl]oxy}ethyl)butyl]-4-chlorobutanamide (3.89 g, 11.6 mmol, 100%) as a yellow oil that was used directly in the next reaction after cursory characterisation. [α]D23 −1.75 (c 2.28, abs. EtOH); vmax (film)/cm−1 3281 (N-H, v br, m), 3075 (w), 2957 and 2932 (C-H, s), 2873 (C-H, m), 1727 (m), 1642 (C=O, s), 1546 (s), 1462 (m), 1442 (m), 1369 (m), 1306 (w), 1254 (m), 1155 (m), 1048 (m), 876 (m), 774 (m), 667 (m); δH (300 MHz; CDCl3) 6.10 (1H, br d, J = ca. 7.3 Hz, NH), 4.11-3.97 (1H, m, NCH), 3.81-3.72 and 3.72-3.63 (2 × 1H, 2 × m, CHaHbOSi), 3.57 (2H, td, J = 6.2 and 1.9 Hz, CH2Cl), 2.28 (2H, t, J = 7.1 Hz, CH2C=O), 2.08 (2H, quintet, J = 6.2 Hz, CH2CH2Cl), 1.84-1.70 and 1.69-1.53 (2 × 1H, 2 × m, CHaHbCH2OSi), 1.51-1.39 (2H, m, NCHCH2Et), 1.37-1.19 (2H, m, CH2Me), 0.92-0.85 and 0.89 (11H, overlapping m and s, CH2Me and SiCMe3), 0.05 (6H, s, SiMe2).
(b) Potassium tert-butoxide (1.80 g, 16.1 mmol) was added in portions (~0.100 g per addition) to a solution of the preceding chlorobutanamide (3.60 g, 10.7 mmol) in dry tert-butyl alcohol (32.0 cm3) over 5 h. The mixture was neutralized with glacial AcOH, and the solvent was removed in vacuo. The resulting residue was dissolved in CH2Cl2 (100 cm3) and washed with H2O (100 cm3). The aqueous extracts were extracted with CH2Cl2 (3 × 100 cm3), and the combined organic extracts were dried (anhydrous Na2SO4), filtered and evaporated in vacuo to afford an orange oil. Purification of the crude oil by column chromatography with hexane-EtOAc (7 : 3) as eluent yielded 1-[(1R)-1-(2-{[tert- butyl(dimethyl)silyl]oxy} ethyl)butyl]pyrrolidin-2-one (33) (3.00 g, 94%) as a light yellow oil. Rf 0.58 (hexane-EtOAc 1 : 1); [α]D19 −9.86 (c 0.71, CHCl3); vmax (film)/cm−1 2954, 2929 and 2857 (C-H, m), 1738 (w), 1668 (C=O, s), 1463 (m), 1423 (m), 1285 (m), 1253 (s), 1095 (s), 1007 (w), 942 (w), 834 (s), 774 (s), 663 (m); δH (300 MHz; CDCl3) 4.21-4.05 (1H, m, NCH), 3.63-3.46 (2H, m, CH2OSi), 3.32-3.18 (2H, m, NCH2), 2.36 (2H, t, J = 8.1 Hz, ring CH2C=), 1.96 (2H, quintet, J = 7.4 Hz, ring 4-H), 1.72-1.65 (2H, m, CH2CH2OSi), 1.50-1.39 (2H, m, NCHCH2Et), 1.28-1.19 (2H, m, CH2Me), 0.88 and 0.86 (12H, overlapping t and s, J = 7.3 Hz, CH2Me and SiCMe3), 0.02 and 0.01 (6H, 2 × s, diastereotopic SiMe2); δC (75 MHz; CDCl3) 174.9, 60.7, 48.5, 42.3, 35.6, 34.7, 31.5, 25.9, 19.4, 18.2 (2 overlapping signals), 13.8, −5.39 and – 5.44 (diastereotopic SiMe2). HRMS (EI) Found, M+, 299.2229. C16H33NO2Si requires 299.2281.
1-[(1R)-1-(2-Hydroxyethyl)butyl]pyrrolidin-2-one (34)
Aq. HF solution (40%, 10.1 cm3) was added slowly to a solution of 1-[(1R)-1-(2-{[tert-Butyl(dimethyl)silyl]oxy}ethyl)butyl]pyrrolidin-2-one (33) (1.89 g, 6.32 mmol) in MeOH (240 cm3). The reaction mixture was stirred at rt for 2 h before the careful addition of saturated aq. NaHCO3 solution (380 cm3), whereupon effervescence was observed. The reaction mixture was then extracted with EtOAc (3 × 200 cm3) and the combined organic extracts were dried (anhydrous Na2SO4), filtered and evaporated in vacuo to yield a light yellow oil. The crude oil was purified by column chromatography with EtOAc as eluent to give 1-[(1R)-1-(2-hydroxyethyl)butyl]pyrrolidin-2-one (34) (1.06 g, 5.69 mmol, 90%) as a clear oil; Rf 0.30 (EtOAc); [α]D23 −0.61 (c 11.5, abs. EtOH); vmax (film)/cm−1 3395 (O-H, v br, m), 2954 and 2872 (C-H, m), 1738 (m), 1655 (C=O, s), 1463 (m), 1424 (m), 1367 (m), 1289 (m), 1229 (m), 1217 (m), 1112 (w), 1048 (m), 1011 (w), 903 (w), 731 (w), 651 (w); δH (300 MHz; CDCl3) 4.23-4.14 (1H, m, NCH), 3.52 (1H, ddd, J = 11.8, 5.3 and 3.2 Hz, CHaHbOH), 3.33 (1H, dd, J = 10.7 and 3.5 Hz, CHaHbOH), 3.28-3.11 and ca 3.11 (3H, overlapping m and br s, NCH2 and OH), 2.41 (2H, td, J = 8.0 and 2.5 Hz, CH2C=O), 1.99 (2H, quintet, J = 7.5 Hz, ring 4-H), 1.76-1.64 (1H, m, CHaHbCH2OH), 1.56-1.35 (3H, m, CHaHbCH2OH and NCHCH2Et), 1.21 (2H, sextet, J = 7.3 Hz, CH2Me), 0.87 (3H, t, J = 7.3 Hz, CH2Me); δC (75 MHz; CDCl3) 176.5, 58.4, 47.3, 41.9, 34.6, 34.4, 31.2, 19.5, 18.1, 13.7. HRMS (EI) Found: M+, 185.1404. C10H19NO2 requires 185.1416.
(3R)-3-(2-Oxopyrrolidin-1-yl)hexyl acetate (35)
A solution of acetic anhydride (1.37 g, 1.30 cm3, 13.5 mmol) in dry pyridine (0.710 g, 0.800 cm3, 8.97 mmol) was added dropwise to a stirred solution of 1-[(1R)-1-(2-hydroxyethyl)butyl]pyrrolidin-2-one (34) (1.66 g, 8.97 mmol) in pyridine (1.06 g, 1.10 cm3, 13.5 mmol). The mixture was stirred at rt for 16 h, after which time the reaction mixture was diluted with EtOAc (45 cm3) and washed with saturated aq. NH4Cl solution (3 × 55 cm3), which was then made basic to pH 10 with aq. NH3 solution. The combined aqueous extracts were extracted further with CH2Cl2 (3 × 55 cm3). The combined organic extracts were dried (anhydrous Na2SO4), filtered and evaporated in vacuo to yield a crude yellow oil. The crude oil was purified by column chromatography with hexane-EtOAc (3 : 2) as eluent to yield (3R)-3-(2-oxopyrrolidin-1-yl)hexyl acetate (35) (1.72 g, 84%) as a clear oil. Rf 0.33 (hexane-EtOAc 1 : 1); [α]D23 +1.89 (c 11.1, CHCl3); vmax (film)/cm−1 2957 and 2934 (C-H, s), 2873 (C-H, m), 1736 (ester C=O, s), 1678 (lactam C=O, s), 1462 (m), 1423 (m), 1367 (m), 1284 (m), 1232 (s), 1036 (m), 648 (m); δH (300 MHz; CDCl3) 4.26-4.16 (1H, m, NCH), 4.01 (2H, t, J = 6.7 Hz, CH2OAc), 3.33-3.20 (2H, m, NCH2), 2.40 (2H, t, J = 8.0 Hz, CH2C=O), 2.04 and 2.02 (5H, overlapping s and quintet, J = 7.4 Hz, OCOMe and ring 4-H), 1.88-1.76, 1.57-1.37 and 1.34-1.20 (3 × 2H, 3 × m, remaining CH2), 0.91 (3H, t, J = 7.2 Hz, CH2Me); δC (75 MHz; CDCl3) 175.1, 171.0, 61.5, 47.9, 41.9, 34.5, 31.3, 31.2, 20.9, 19.3, 18.2, 13.8. HRMS (EI) Found: (M–H)+, 226.1439. C12H20NO3 requires 226.1438.
(3R)-3-(2-Thioxopyrrolidin-1-yl)hexyl acetate (36)
Phosphorus pentasulfide (4.87 g, 21.9 mmol) and Na2CO3 (1.17 g, 11.0 mmol) were dissolved in dry tetrahydrofuran (55.0 cm3); the reaction was exothermic, and effervescence was observed. Once a homogeneous solution had formed, (3R)-3-(2-oxopyrrolidin-1-yl)hexyl acetate (35) (1.66 g, 7.31 mmol) was slowly added. The solution was stirred at rt for 3 h, after which the reaction was quenched by the addition of aq. Na2CO3 solution (10%, 55 cm3). Vigorous effervescence was again observed. The solution was stirred for a further 10 min before adding EtOAc (40 cm3) and hexane (13 cm3). The organic phase was separated and the aqueous phase was further extracted with CH2Cl2 (3 × 30 cm3). The combined organic phases were dried (anhydrous Na2SO4), filtered and evaporated in vacuo to yield a yellow oil. The crude oil was purified by column chromatography hexane-EtOAc (7 : 3) as eluent to give (3R)-3-(2-thioxopyrrolidin-1-yl)hexyl acetate (36) (1.61 g, 91%) as a yellow oil. Rf 0.25 (hexane-EtOAc 7 : 3); [α]D17 +23.7 (c 1.69, CHCl3); δH (300 MHz; CDCl3) 5.19-5.10 (1H, br quintet, J = ca 7.5 Hz, NCH), 4.03 (2H, t, J = 6.7 Hz, NCH2), 3.62-3.47 (2H, m, CH2OAc), 3.03 (2H, t, J = 7.8 Hz, CH2C=S), 2.06 and 2.05 (5H, overlapping quintet and s, J = 7.5 Hz, ring 4-H and OCOMe), 1.93-1.79, 1.62-1.50 and 1.39-1.15 (3 × 2H, 3 × m, remaining CH2), 0.94 (3H, t, J = 7.2 Hz, CH2Me); δC (75 MHz; CDCl3) 202.3, 171.0, 61.2, 53.1, 48.6, 45.1, 34.7, 31.5, 21.0, 20.0, 19.2, 13.9. HRMS (EI) Found: M+, 243.1285. C12H21NO2S requires 243.1293.
(3R)-3-[(2E)-2-{2-[Methoxy(methyl)amino]-2-oxoethylidene}pyrrolidin-1-yl]hexyl acetate (37)
(3R)-3-(2-Thioxopyrrolidin-1-yl)hexyl acetate (36) (1.58 g, 6.51 mmol) and 2-bromo-N-methoxy- N-methylacetamide (2.13 g, 11.7 mmol, 1.8 eq.) were dissolved in dry MeCN (26.0 cm3) and stirred at rt for 16 h. The solvent and excess bromoacetamide were removed in vacuo, and the residue was re-dissolved in MeCN (26.0 cm3). To this was added PPh3 (2.57 g, 9.77 mmol), followed by NEt3 (0.988 g, 1.36 cm3, 9.77 mmol). The mixture was stirred at rt for 3 h, during which time a white precipitate formed. The reaction mixture was filtered through a thin pad of celite, and the solids were washed with EtOAc (100 cm3) The solvent was removed in vacuo, and the residue was triturated with EtOAc (150 cm3) for 30 min before being again filtered through a thin pad of celite. The filtrate was extracted with aq. HCl solution (2 M, 3 × 50 cm3), and the aqueous extracts were basified to pH 10 with aq. NH3 solution. The basic phase was then extracted with CH2Cl2 (3 × 100 cm3), and the combined organic extracts were dried (anhydrous Na2SO4), filtered and evaporated in vacuo to afford a crude orange oil. Spectroscopic analysis showed a mixture of (3R)-3-[(2E)-2-{2-[methoxy(methyl)amino]-2-oxoethylidene}-pyrrolidin-1-yl]hexyl acetate (37) and phosphorus-containing residues, which were inseparable by column chromatography, and as such the mixture was carried forward crude (~ 2g) after cursory characterisation; δH (300 MHz; CDCl3) 5.19 (1H, s, C=CH), 4.11-4.01 and 4.00-3.90 (2 × 1H, 2 × m, CHaHbOAc), 3.85-3.75 (1H, m, NCH), 3.66 (3H, s, OMe), 3.28-3.19 and 3.24 (4H, m and t, J = 7.4 Hz, ring 5-H and 3-H), 3.14 (3H, s, NMe), 2.04 (3H, s, OCOMe), 1.95-1.83 (4H, m, ring 4-H and , CH2CH2Ac), 1.67-1.39 (2H, m, NHCH2Et), 1.28 (2H, sextet, J = 7.4 Hz, CH2Me), 0.92 (3H, t, J = 7.3 Hz, CH2Me); δC (75 MHz; CDCl3) 172.1, 170.8, 165.4, 76.9, 61.4, 60.7, 50.8, 45.3, 34.4, 32.8, 31.2 , 33.0, 21.1, 20.8, 19.4, 13.8.
(2E)-2-{1-[(1R)-1-(2-Hydroxyethyl)butyl]pyrrolidin-2-ylidene}-N-methoxy-N-methylethanamide (29)
K2CO3 (1.35 g, 9.77 mmol) was added to a stirred solution of crude (3R)-3-[(2E)-2-{2-[methoxy(methyl)amino]-2-oxoethylidene}pyrrolidin-1-yl]hexyl acetate (37) (~ 2g, ~6.5 mmol) in dry MeOH (10.4 cm3). The mixture was stirred at rt for 3 h, after which time it was filtered through a thin pad of celite. The filtrate was evaporated in vacuo to afford an orange oil. The crude orange oil was purified by column chromatography with CH2Cl2-MeOH (19 : 1) as eluent to afford (2E)-2-{1-[(1R)-1-(2-hydroxyethyl)butyl]pyrrolidin-2-ylidene}-N-methoxy-N-methylethanamide (29) (1.05 g, 64%, 3 steps from 36) as a clear oil; characterisation as described previously.
(5R)-N-Methoxy-N-methyl-5-propyl-1,2,3,5,6,7-hexahydroindolizine-8-carboxamide (38)
Imidazole (0.0790 g, 1.14 mmol, 3.0 eq.) and PPh3 (0.301 g, 1.14 mmol, 3.0 eq.) were added to a stirred solution of (2E)-2-{1-[(1R)-1-(2-hydroxyethyl)butyl]pyrrolidin-2-ylidene}-N-methoxy-N-methylethanamide (29) (0.103 g, 0.379 mmol) in MeCN-PhMe (2.30 cm3 : 1.10 cm3). The solution was stirred for 30 min, after which time I2 (0.192 g, 0.758 mmol) was added in one portion. The resulting homogenous solution was heated at reflux for 1 h. The reaction was quenched with saturated aq. NaHCO3 solution (4 cm3), and extracted with EtOAc (3 × 20 cm3). The combined organic fractions where washed with saturated aq. Na2S2O3 solution (20 cm3), separated, dried (anhydrous Na2SO4), filtered and evaporated in vacuo to give a yellow solid. The crude solid was purified by column chromatography, initially eluting the unreacted PPh3 with CH2Cl2, then eluting the product with CH2Cl2-MeOH (19 : 1). (5R)-N-Methoxy-N-methyl- 5-propyl- 1,2,3,5,6,7-hexahydroindolizine-8-carboxamide (38) was obtained as a light yellow oil (0.045 g, 47%); vmax (film)/cm−1 2928 and 2857 (C-H, m), 1630 (C=O, m), 1555 (C=C, s), 1438 (m), 1401 (m), 1361 (m), 1281 (s), 1194 (m), 1154 (m), 1118 (m), 1024 (m), 1003 (m), 725 (m), 696 (m); δH (300 MHz; CDCl3) 3.63 (3H, s, OMe), 3.48 (1H, td, J = 8.5 and 5.2 Hz, 3eq-H), 3.24-3.05 (2H, m, 3ax-H and 5-H), 3.07 (3H, s, NMe ), 2.34 (2H, br t, J = 6.2 Hz, 1-H or 7-H), 1.98-1.82 (2H, m, 1-H or 7-H), 1.80-1.52 (4H, m, 2-H and 6-H), 1.45-1.21 (4H, m, CH2CH2Me), 0.95 (3H, t, J = 6.9 Hz, CH2Me); δC (75 MHz; CDCl3) 174.4, 157.4, 90.1, 59.7, 53.8, 51.0, 35.1, 34.4, 32.1, 25.3, 21.5, 20.6, 19.0, 14.1. HRMS (EI) Found: M+, 252.1828. C14H24N2O2 requires 252.1838.
(5R,8S,8aS)-N-Methoxy-N-methyl-5-propyloctahydroindolizine-8-carboxamide (39)
Adams catalyst (34 mg) was added to a solution of (5R)-N-methoxy-N-methyl- 5-propyl-1,2,3,5,6,7-hexahydroindolizine-8-carboxamide (38) (0.169 g, 0.670 mmol) in glacial AcOH (3.70 cm3). This was stirred at rt under an atmosphere of H2 for 24 h. The solution was filtered through a pad of celite, washed several times with EtOH, and evaporated in vacuo to afford a grey oil. The crude oil was taken up in H2O (50 cm3) and neutralized with saturated aq. NaHCO3 solution. The neutralised aqueous fraction was extracted into CH2Cl2 (3 × 50 cm3), the combined organic extracts were then dried (anhydrous Na2SO4), filtered and evaporated in vacuo to afford a grey oil. The crude oil was purified by column chromatography with CH2Cl2-MeOH (19 : 1) as eluent to yield (5R,8S,8aS)-N-methoxy-N-methyl- 5-propyloctahydro-8-indolizinecarboxamide (39) as a clear oil (0.136 g, 80%); [α]D21 −57.3 (3.07, abs. EtOH); vmax (film)/cm−1 2955, 2930 (C-H, m), 2880 (w), 2790 (w, Bohlmann band), 1668 (C=O, s), 1459 (m), 1372 (m), 1097 (m), 996 (s), 801 (m), 678 (s); δH (300 MHz; CDCl3) 3.65 (3H, s, OMe), 3.33 (1H, td, J = 8.4 and 2.2 Hz, 3eq-H), 3.17 (3H, s, NMe), 2.14-2.07 (1H, m), 2.04-1.91 (2H, m), 1.86-1.76 (4H, m), 1.74-1.50 (5H, m), 1.48-1.33 (4H, m), 0.90 (3H, t, J = 7.0 Hz, CH2Me); δC (75 MHz; CDCl3) 174.9, 65.7, 64.2, 61.0, 51.6, 43.9, 36.7, 35.8, 28.1, 27.3, 27.1, 19.9, 18.9, 14.5. HRMS (EI): no M+ discernible.
1-[(5R,8S,8aS)-5-Propyloctahydroindolizin-8-yl]propan-1-one (40)
Ethylmagnesium bromide in THF (1.24 M, 1.20 cm3, 1.49 mmol) was added slowly to a solution of (5R,8S,8aS)-N-methoxy-N-methyl-5-propyloctahydroindolizine-1-carboxamide (39) (0.0760 g, 0.300 mmol) in THF (3.00 cm3) at 0 °C. The reaction mixture was allowed to warm to rt, then was stirred for a further 24 h. The reaction was then quenched with aq. HCl solution (6 M) and evaporated in vacuo. The residue was taken up in H2O (50 cm3), basified with conc. aq. NH3 solution, and extracted with Et2O (3 × 50 cm3). The combined organic extracts were dried (anhydrous Na2SO4), filtered and evaporated in vacuo to afford a light yellow oil. The crude oil was purified by passing it through a short plug of silica, with CH2Cl2-MeOH (19 : 1) as eluent. 1-[(5R,8S,8aS)- 5-Propyloctahydroindolizin-8-yl]propan-1-one (40) was obtained as a clear oil (0.056 g, 83%). Rf 0.50 (CH2Cl2-MeOH 19 : 1); [α]D19 +48.3 (c 0.95, CHCl3); δH (300 MHz; CDCl3) 3.28 (1H, br t, J = ca 8.3 Hz, 3eq-H), 2.85-2.78 (1H, m, 8-H), 2.63 (1H, dq, J = 18.0 and 7.2 Hz, CHaHbC=O), 2.52-2.39 (1H, m, CHaHbC=O), 2.15-2.02 (1H, m, 3ax-H, 5-H), 1.96-1.14 (15H, m), 1.01 (3H, t, J = 7.4 Hz, CH2Me), 0.91 (3H, t, J = 7.0 Hz, CH2Me); δC (75 MHz; CDCl3) 213.6, 65.2, 63.9, 51.7, 48.4, 37.5, 36.8, 27.9, 27.3, 26.8, 20.4, 18.6, 14.5, 7.6. HRMS (EI) Found: M+, 223.1936. C14H25NO requires 223.1936.
1-[(5R,8R,8aS)-5-Propyloctahydroindolizin-8-yl]propan-8-one (41)
A solution of NaOMe was prepared by adding metallic Na (0.010 g, 0.042 mmol) to dry MeOH (5.0 cm3). To this solution was added 1-[(5R,8S,8aS)-5-propyloctahydroindolizin-8-yl]propan-1-one (40) (9.4 mg, 0.042 mmol) in one portion. The reaction mixture was heated at reflux for 3 h, then cooled to rt. The solvent was removed in vacuo, and the resulting residue was re-dissolved in H2O (10 cm3) and extracted with Et2O (3 × 20 cm3). The combined organic extracts were dried (anhydrous Na2SO4), filtered and evaporated in vacuo to give a yellow oil. The crude oil was purified by column chromatography CH2Cl2-MeOH (19 : 1) containing a few drops of propylamine as eluent. 1-[(5R,8R,8aS)-5-Propyloctahydroindolizin-8-yl]- propan-1-one (41) was obtained as a clear oil (7.5 mg, 80%). Rf 0.50 (CH2Cl2-MeOH 9 : 1); [α]D17 −74.3 (c 0.35, CHCl3), lit.,6 [α]D22 −84.4 (c 1.0, CHCl3); vmax (film)/cm−1 2958, 2930, 2873 (C-H, m), 2783 (Bohlmann band, m), 1715 (C=O, s), 1693 (s), 1458 (m), 1373 (m), 1262 (m), 1192 (m), 1120 (s), 1019 (m), 800 (m); δH (300 MHz; CDCl3) 3.27 (1H, br t, J = ca 8.3 Hz, 3eq-H), 2.59-2.35 (3H, m, CH2C=O and 5-H), 2.15-1.70 (5H, m), 1.70-1.55 (3H, m), 1.45-1.20 (7H, m), 1.04 (3H, t, J = 7.3 Hz, CH2Me), 0.91 (3H, t, J = 7.1 Hz, CH2Me); δC (75 MHz; CDCl3) 213.5, 65.5, 62.8, 54.6, 51.0, 36.7, 36.0, 28.7 (2 overlapping signals), 28.4, 20.5, 18.9, 14.5, 7.6. HRMS (EI) Found: M+, 223.1925, C14H25NO requires 223.1936.
ACKNOWLEDGEMENTS
This work was supported by grants from the National Research Foundation, Pretoria (grant number 2053652) and the University of the Witwatersrand.
References
1. J. W. Daly, H. M. Garraffo, and T. F. Spande, ‘Alkaloids: Chemical and Biological Perspectives’, Vol. 13, ed. by S. W. Pelletier, Pergamon Press, Amsterdam, 1999, pp.1-161.
2. J. W. Daly, T. F. Spande, and H. M. Garraffo, J. Nat. Prod., 2005, 68, 1556. CrossRef
3. J. P. Michael, Nat. Prod. Rep., 2008, 25, 139, and previous reviews in the series. CrossRef
4. J. P. Michael, ‘The Alkaloids. Chemistry and Pharmacology’, Vol. 55, ed. by G. A. Cordell, Academic Press, New York, 2001, pp. 91-258.
5. D. Enders and C. Thiebes, Synlett, 2000, 12, 1745.
6. S. Yu, W. Zhu, and D. Ma, J. Org. Chem., 2005, 70, 7364. CrossRef
7. N. Toyooka, Z. Dejun, H. Nemoto, H. M. Garraffo, T. F. Spande, and J. W. Daly, Tetrahedron Lett., 2006, 47, 581. CrossRef
8. N. Toyooka, D. Zhou, H. Nemoto, H. M. Garraffo, T. F. Spande, and J. W. Daly, Beilstein J. Org. Chem., 2007, 3, no. 29, (doi: 10.1186/1860-5397-3-29). CrossRef
9. S. Kobayashi, N. Toyooka, D. Zhou, H. Tsuneki, T. Wada, T. Sasaoka, H. Sakai, H. Nemoto, H. M. Garraffo, T. F. Spande, and J. W. Daly, Beilstein J. Org. Chem., 2007, 3, no. 30, (doi: 10.1186/1860-5397-3-30). CrossRef
10. N. Toyooka, Z. Dejun, H. Nemoto, H. M. Garraffo, T. F. Spande, and J. W. Daly, Heterocycles, 2006, 70, 541. CrossRef
11. N. Toyooka, K. Tanaka, T. Momose, J. W. Daly, and H. M. Garraffo, Tetrahedron, 1997, 53, 9553. CrossRef
12. N. Toyooka, H. Nemoto, and M. Kawasaki, Tetrahedron, 2005, 61, 1187, (Corrigendum: Tetrahedron, 2005, 61, 5139). CrossRef
13. P. Michel, A. Rassat, J. W. Daly, and T. F. Spande, J. Org. Chem., 2000, 65, 8908. CrossRef
14. J. P. Michael, C. B. de Koning, D. Gravestock, G. D. Hosken, A. S. Howard, C. M. Jungmann , R. W. M. Krause, A. S. Parsons, S. C. Pelly, and T. V. Stanbury, Pure Appl. Chem., 1999, 71, 979. CrossRef
15. J. P. Michael and D. Gravestock, J. Chem. Soc., Perkin Trans. 1, 2000, 1919.
16. J. P. Michael and D. Gravestock, Eur. J. Org. Chem., 1998, 865. CrossRef
17. J. P. Michael and D. Gravestock, S. Afr. J. Chem., 1998, 51, 146.
18. S. G. Davies and O. Ichihara, Tetrahedron: Asymmetry, 1991, 2, 183; CrossRef J. F. Costello, S. G. Davies, and O. Ichihara, Tetrahedron: Asymmetry, 1994, 5, 1999. CrossRef
19. Review: S. G. Davies, A. D. Smith, and P. D. Price, Tetrahedron: Asymmetry, 2005, 16, 2833. CrossRef
20. M. Roth, P. Dubs, E. Götschi, and A. Eschenmoser, Helv. Chim. Acta, 1971, 54, 710. CrossRef
21. K. Shiosaki, in ‘Comprehensive Organic Synthesis’, Vol. 2, ed. by B. M. Trost, Pergamon Press, Oxford, 1991, pp. 865-892.
22. S. Nahm and S. M. Weinreb, Tetrahedron Lett., 1981, 22, 3815, Review: J. Singh, N. Satyamurthi, and I. S. Aidhen, J. Prakt. Chem., 2000, 342, 340. CrossRef
23. M. Inglesi, M. Nicola, and S. Magnetti, Farmaco, 1990, 45, 1327. CrossRef
24. M. F. Mechelke and A. I. Meyers, Tetrahedron Lett., 2000, 41, 4339. CrossRef
25. J. P. Michael, C. B. de Koning, and C. W. van der Westhuyzen, Org. Biomol. Chem., 2005, 3, 836. CrossRef
26. J. P. Michael and A. S. Parsons, S. Afr. J. Chem., 1993, 46, 65.
27. P. J. Garegg and B. Samuelsson, J. Chem. Soc., Perkin Trans. 1, 1980, 2866. CrossRef
28. C. Accone, C. B. de Koning, J. P. Michael, and C. W. van der Westhuyzen, Beilstein J. Org. Chem., 2008, 4, no. 5, (doi: 10.1186/1860-5397-4-5). CrossRef
29. D. Brillon, Synth. Commun., 1990, 20, 3085. CrossRef
30. F. Bohlmann, Chem. Ber., 1958, 91, 2157. CrossRef