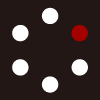
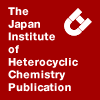
HETEROCYCLES
An International Journal for Reviews and Communications in Heterocyclic ChemistryWeb Edition ISSN: 1881-0942
Published online by The Japan Institute of Heterocyclic Chemistry
e-Journal
Full Text HTML
Received, 9th October, 2008, Accepted, 1st December, 2008, Published online, 9th December, 2008.
DOI: 10.3987/COM-08-S(D)69
■ Organic Chemistry Using Weakly Electrophilic Salts: The Reaction with Nitrogen Nucleophiles
Hiromichi Fujioka,* Ozora Kubo, Kento Senami, Kazuhisa Okamoto, Takashi Okitsu, and Yasuyuki Kita*
Graduate School of Pharmaceutical Science, Osaka University, 1-6 Yamadaoka, Suita, Osaka 560-0871, Japan
Abstract
The reaction of electrophilc salts, which were obtained by the treatment of acetals with TESOTf―2,4,6-collidine, with nitrogen nucleophiles was studied in detail. Treatment of the salts with potassium phthalimide afforded the corresponding N,O-acetals in good yields. The use of hydrazine in place of potassium phthalimide gave the corresponding hydrazones. The reaction is very mild and chemoselective, and acid-labile functional groups can tolerate these conditions.INTRODUCTION
Recently, we have been developing an organic chemistry using weakly electrophilic salts obtained by the reaction of acetal compounds under triethylsilyl triflate (TESOTf) ―2,4,6-collidine conditions.1 Based on initial results, we reported the efficient formation of O,O-mixed, O,S- and N,O-acetals (Scheme 1). The O,O-mixed, O,S-acetals were prepared by the nucleophilic attack of alcohols and thiols or lithium thiolates, respectively. On the other hand, amine nucleophiles, such as benzylamine and N-methylbenzylamine, did not give the desired N,O-acetals, and the aldehydes derived from the starting acetals were obtained. Only sodium azide or trimethylsilyl azide (TMSN3) gave the N,O-acetals, in which the nitrogen unit was an azide.1f
We then studied in detail the reaction of the salts with other nitrogen nucleophiles and obtained some interesting results. We now present our study on this subject.
†This paper is dedicated to the memory of the late Dr. John Daly.
RESULTS AND DISCUSSION
The results of the reactions using benzylamine and N-methylbenzylamine, which afforded aldehydes, not N,O-acetals, suggested two possibilities. One is the weak nucleophilicity of such amines. However, this seemed to be unlikely, because the nucleophilicity of the nitrogen atom is usually much stronger than that of the oxygen atom. Another possibility, the over reaction of N,O-acetals to iminium ions, which are easily hydrolized to aldehydes (Scheme 2 shows the reaction using N-methylbenzylamine), then seemed plausible.
To prove the hypothesis in Scheme 2, the reaction of decanal dimethyl acetal (1) and phthalimide as a nitrogen nucleophile was examined hoping that the obtainable N,O-acetal would not give the iminium salt due to the weak electron-donating ability of the nitrogen of phthalimide. TESOTf (2 equiv) was added to a solution of 1 (1 equiv) and 2,4,6-collidine (3 equiv) in CH2Cl2 (0.1 M solution), and then the mixture was stirred for 30 min at 0 °C. Treatment of the resulting solution with potassium phthalimide
(2) for 15 min at rt gave the N,O-acetal 3 in good yield (80%), whereas treatment of the resulting salt with phthalimide did not give 3 at all due to the weak nucleophilicity of the nitrogen atom (Scheme 3).
The result in Scheme 3 showed that the hypothesis in Scheme 2 was correct. This was also proved by getting hydrazone product 5, using hydrazine as a nitrogen nucleophile.2 Thus, the reaction of the salt, which was prepared in the same way as Scheme 3, with 1-aminopiperidine (4) gave the hydrazone 5 in moderate yield.
Features of our organic chemistry using weakly electrophilic salts are 1) the reaction proceeds under weakly basic conditions, which allow the presence of acid-labile functional group, and 2) the reaction is highly chemoselective. These points and the generality of the reactions in Schemes 3 and 4 were proved from the results in Table 1. The reaction using an acetal 6 having tert-butyldimethylsilyl (TBS) ether and potassium phthalimide (2) as a nitrogen nucleophile afforded the N,O-acetal 9 in good yield (entry 1). The use of 1-aminopiperidine (4) in place of 2 afforded the corresponding hydrazone 10 (entry 2). During the reactions, TBS-ether unit was remained intact. Furthermore, even compound 7 having trityl (Tr) ether was also available, and the corresponding N,O-acetal 11 and hydrazone 12 were obtained (entries 3 and 4). It is noteworthy that the acid-labile trityl ether was tolerant during the reaction. High chemoselectivity was shown from the reactions in entries 5 and 6. As a substrate, compound 8 having the acetal and ketal functions in the same molecule was used. Since we have already proved the chemoselective formation of the intermediates from the substrates having the acetal and ketal functions (see refs. 1a,b and 2), the reaction smoothly proceeded to give the corresponding N,O-acetal 13 and hydrazone 14, both of which have a ketal functional group.
CONCLUSION
We proved that electrophilic salts, prepared by the treatment of acetals with the TESOTf―2,4,6-collidine combination, could react with nitrogen nucleophiles such as benzylamine and N-methylbenzylamine, but the iminium species could not be isolated due to their instability against water. We then used phthalimide and hydrazine as nitrogen nucleophiles and got the corresponding N,O-acetal and hydrazone in moderate to good yields. The reaction is mild and highly chemoselective, and an acid-labile functional group can tolerate such conditions.
EXPERIMENTAL
General techniques
The 1H and 13C NMR spectra were measured by 500 MHz, 300 MHz or 270 MHz spectrometers with tetramethylsilane as an internal standard at 20-25 oC. IR spectra were recorded by a diffuse reflectance measurement of samples dispersed in KBr powder. Merck silica gel 60 was used for column chromatography.
Acetals 11a-1c, 1f 61b-1c, 1f, 71c, 1f, and 81f are known in the literatures.
General Procedure for Preparation of N,O-Acetals 3, 9, 11, and 13
2,4,6-Collidine (3.0 equiv) and TESOTf (2.0 equiv) were added to a solution of an acetal in CH2Cl2 (0.1 M) at 0 oC under N2. The reaction mixture was stirred for 30 min at the same temperature. After checking disappearance of the substrate on TLC, potassium phthalimide (2) (3.0 equiv) and 18-crown-6 (3.0 equiv) were added to the reaction mixture and the solution was stirred at rt. Disappearance of the polar component was ascertained by TLC. The mixture was quenched with H2O and extracted with CH2Cl2. The organic layer was dried over Na2SO4, filtered, and evaporated in vacuo. The residue was purified by flash SiO2 column chromatography to give an N,O-acetal.
General Procedure for Preparation of Hydrazones 5, 10, 12, and 14
2,4,6-Collidine (3.0 equiv) and TESOTf (2.0 equiv) were added to a solution of an acetal in CH2Cl2 (0.1 M) at 0 oC under N2. The reaction mixture was stirred for 30 min at the same temperature. After checking disappearance of the substrate on TLC, 1-aminopiperidine (4) (2.0 equiv) was added to the reaction mixture and the solution was stirred at 0 oC. Disappearance of the polar component was ascertained by TLC. The mixture was quenched with H2O (2.0 mL) and extracted with CH2Cl2. The organic layer was dried over Na2SO4, filtered, and evaporated in vacuo. The residue was purified by flash SiO2 column chromatography to give a hydrazone. It containes the aldehyde, which is difficult to be separated from the hydrazone. The yield of the hydrazone was determined by 1H NMR. Purification in several times by flash SiO2 column chromatography gave pure hydrazone.
Experiment in Scheme 3: According to the general procedure, treatment of 1 (37.0 mg, 0.183 mmol) with 2,4,6-collidine (73 μL, 0.549 mmol), TESOTf (83 μL, 0.366 mmol), 2 (101.6 mg, 0.549 mmol), and 18-crown-6 (145.0 mg, 0.549 mmol) gave 3 (48.3 mg, 80%). Eluent; hexanes-AcOEt (10/1). 3: Colorless oil; IR (KBr) 2926, 1776, 1717, 1350, 1115 cm-1; 1H NMR (300 MHz, CDCl3) δ 0.78 (3H, t, J = 6.0 Hz), 1.16-1.43 (14H, m), 2.05-2.26 (2H, m), 3.31 (3H, s), 5.24 (1H, t, J = 6.9 Hz), 7.69-7.75 (2H, m), 7.82-7.88 (2H, m); 13C NMR (68 MHz, CDCl3) δ 14.9, 23.4, 26.2, 29.8, 30.0, 30.2, 32.6, 33.1, 57.2, 84.4, 124.1, 132.1, 134.8, 168.6. Anal. Calcd for C19H27NO3: C, 71.80; H, 8.57; N, 4.41. Found: C, 72.06; H, 8.64; N, 4.29.
Experiment in Scheme 4: According to the general procedure, treatment of 1 (41.3 mg, 0.204 mmol) with 2,4,6-collidine (81 μL, 0.612 mmol), TESOTf (92 μL, 0.408 mmol), and 4 (44 μL, 0.408 mmol) gave 5 (25.2 mg, 52 %). Eluent; hexanes-AcOEt (10/1). 5: Colorless oil; IR (KBr) 2928, 2855, 1609, 1454, 1360 cm-1; 1H NMR (300 MHz, CDCl3) δ 0.88 (3H, t, J = 6.3 Hz), 1.22-1.35 (12H, m), 1.42-1.52 (4H, m), 1.66-1.74 (4H, m), 2.19-2.26 (2H, m), 2.89-2.92 (4H, t like), 6.95 (1H, t, J = 5.4 Hz); 13C NMR (68 MHz, CDCl3) δ 14.1, 22.6, 24.1, 25.3, 27.6, 29.2, 29.3, 29.4, 29.5, 31.9, 33.2, 52.9, 141.8; HRMS (FAB) calcd for C15H31N2 (M++H) 239.2487, found 239.2482.
Experiments in Table 1
Entry 1: According to the general procedure, treatment of 6 (47.2 mg, 0.131 mmol) with 2,4,6-collidine (52 μL, 0.393 mmol), TESOTf (59 μL, 262 mmol), 2 (73.3 mg, 0.396 mmol), and 18-crown-6 (102.2 mg, 0.387 mmol) gave 9 (48.3 mg, 78%). Eluent; hexanes-AcOEt (8 /1). 9: Colorless oil; IR (KBr) 2928, 1776, 1715, 1325, 1099 cm-1; 1H NMR (270 MHz, CDCl3) δ 0.04 (6H, s), 0.89 (9H, s), 1.24-1.52 (18H, m), 2.09-2.29 (2H, m), 3.35 (3H, s), 3.59 (2H, t, J = 6.4 Hz), 5.27 (1H, t, J = 7.1 Hz), 7.74-7.77 (2H, m), 7.87-7.90 (2H, m); 13C NMR (68 MHz, CDCl3) δ –5.3, 18.3, 25.4, 25.8, 26.0, 29.4 (2C), 29.5, 29.6, 32.3, 32.8, 56.5, 63.3, 83.7, 123.5, 131.6, 134.2, 168.1; HRMS (FAB) calcd for C27H46NO4Si (M++H) 476.3196, found 476.3193.
Entry 2: According to the general procedure, treatment of 6 (74.3 mg, 0.206 mmol) with 2,4,6-collidine (81 μL, 0.618 mmol), TESOTf (93 μL, 0.412 mmol), and 4 (44 μL, 0.412 mmol) gave 10 (47.1 mg, 58%). Eluent; hexanes-AcOEt (10/1). 10: Colorless oil; IR (KBr) 2855, 1603, 1556, 1454, 1097 cm-1; 1H NMR (300 MHz, CDCl3) δ 0.00 (6H, s), 0.85 (9H, s), 1.22-1.30 (14H, m), 1.37-1.48 (6H, m), 1.61-1.69 (4H, m), 2.14-2.21 (2H, m), 2.86 (t like, J = 5.4 Hz), 3.55 (t, J = 6.6), 6.90 (1H, J = 5.7 Hz); 13C NMR (75 MHz, CDCl3) δ -5.3, 18.4, 24.1, 25.3, 25.8, 26.0, 27.6, 29.2, 29.4, 29.5 (3C), 29.6, 32.9, 33.3, 53.0, 63.3, 141.8; HRMS (FAB) calcd for C23H49N2OSi (M++H) 397.3614, found 397.3610.
Entry 3: According to the general procedure, treatment of 7 (58.2 mg, 0.119 mmol) with 2,4,6-collidine (47 μL, 0.357 mmol), TESOTf (54 μL, 0.238 mmol), 2 (66.8 mg, 0.361 mmol), and 18-crown-6 (95.2 mg, 0.360 mmol) gave 11 (45.6 mg, 63%). Eluent; hexanes-AcOEt (6 /1). 11: Colorless oil; IR (KBr) 2930, 1776, 1713, 1348, 1070 cm-1; 1H NMR (270 MHz, CDCl3) δ 1.21-1.65 (18H, m), 2.04-2.31 (2H, m), 3.03 (2H, t, J = 6.4 Hz), 3.34 (3H, s), 5.27 (1H, t, J = 7.0 Hz), 7.18-7.31 (9H, m), 7.42-7.45 (6H, m), 7.72-7.75 (2H, m), 7.86-7.89 (2H, m); 13C NMR (68 MHz, CDCl3) δ 25.4, 26.2, 29.0, 29.4 (2C), 29.5 (2C), 30.0, 32.3, 56.5, 63.6, 83.7, 86.2, 123.5, 126.7, 127.6, 128.7, 131.6, 134.2, 144.5, 168.1; HRMS (FAB) calcd for C40H45NO4Na (M++Na) 626.3246, found 626.3246.
Entry 4: According to the general procedure, treatment of 7 (96.4 mg, 0.197 mmol) with 2,4,6-collidine (78 μL, 0.592 mmol), TESOTf (89 μL, 0.395 mmol), and 4 (43 μL, 0.395 mmol) gave 12 (36.1 mg, 35%). Eluent; hexanes-AcOEt (10/1). 12: Colorless oil, IR (KBr) 2930, 2855, 1597, 1448, 1069, cm-1, 1H NMR (270MHz, CDCl3) δ 1.24-1.37 (16H, m), 1.42-1.52 (2H, m), 1.55-1.73 (6H, m), 2.18 (2H, m), 2.90 (4H, t like), 3.03 (3H, t, J = 5.7 Hz), 6.94 (3H, t, J = 5.7 Hz), 7.18-7.31 (9H, m), 7.41-7.46 (6H, m); 13C NMR (68 MHz, CDCl3) δ 24.2, 25.3 (2C), 26.3, 27.6, 29.2, 29.5 (3C), 29.6, 30.0, 33.3, 53.0, 63.7, 86.2, 126.8, 127.7, 128.7, 141.8, 144.5; HRMS (FAB) calcd for C36H49N2O (M++H) 525.3845, found 525.3790.
Entry 5: According to the general procedure, treatment of 8 (33.0 mg, 0.145 mmol) with 2,4,6-collidine (57 μL, 0.435 mmol), TESOTf (65 μL, 0.290 mmol), 2 (80.3 mg, 0.434 mmol), and 18-crown-6 (114.8 mg, 0.434 mmol) gave 13 (47.0 mg, 66%). Eluent; hexanes-AcOEt (3 /1). 13: Colorless oil; IR (KBr) 2934, 1776, 1712, 1348, 1103 cm-1; 1H NMR (270 MHz, CDCl3) δ 0.54 (6H, q, J = 7.9 Hz), 0.90 (9H, t, J = 7.9 Hz), 1.23-1.55 (5H, m), 1.70-1.79 (4H, m), 2.02-2.10 (1H, m), 2.21-2.31 (1H, m), 3.51-3.59 (2H, m), 3.70-3.74 (2H, m), 3.92 (4H, s), 5.52 (1H, t, J = 7.0 Hz), 7.73-7.77 (2H, m), 7.86-7.89 (2H, m); 13C NMR (68 MHz, CDCl3) δ 4.2, 6.7, 29.9 (2C), 32.9, 34.2, 38.6, 61.7, 64.1, 70.3, 80.8, 108.8, 123.5, 131.6, 134.2, 168.0; HRMS (FAB) calcd for C26H40NO6Si (M++H) 490.2625, found 490.2603.
Entry 6: According to the general procedure, treatment of 8 (45.9 mg, 0.201 mmol) in CH2Cl2 (1.0 mL, 0.2 M) with 2,4,6-collidine (79 μL, 0.603 mmol), TESOTf (91 μL, 0.402 mmol), and 4 (65 μL, 0.603 mmol) gave the mixture of 2,4,6-collidine and 14. Since 14 was unstable and hard to be separated from 2,4,6-collidine, the yield of 14 (42%) was determined by 1H NMR. Eluent; hexanes-AcOEt (100/1 to 2/1). 14: Yellow oil; IR (KBr) 2939, 1603, 1447, 1375, 1105 cm-1; 1H NMR (300 MHz, CDCl3) δ 1.26-1.76 (15H, m), 2.18 (2H, t, J = 6.3 Hz), 2.91 (4H, m), 3.94 (4H, s), 6.96 (1H, t, J = 5.7 Hz); 13C NMR (75 MHz, CDCl3) δ 24.1, 25.3, 30.0, 34.4, 35.6, 39.5, 52.9, 64.2 (2H), 108.9, 140.0; HRMS (FAB) calcd for C15H27N2O2 (M++H) 267.2073, found 267.2076.
ACKNOWLEDGEMENTS
This work was financially supported by Grants-in-Aid for Scientific Research (A) and (B) and Grant-in-Aid for Scientific Research for Exploratory Research from Japan Society for the Promotion of Science.
References
1. a) H. Fujioka, Y. Sawama, N. Murata, T. Okitsu, O. Kubo, S. Matsuda, and Y. Kita, J. Am. Chem. Soc., 2004, 126, 11800; CrossRef b) H. Fujioka, T. Okitsu, Y. Sawama, N. Murata, R. Li, and Y. Kita, J. Am. Chem. Soc., 2006, 128, 5930; CrossRef c) H. Fujioka, T. Okitsu, Y. Sawama, T. Ohnaka, and Y. Kita, Synlett, 2006, 18, 3077; CrossRef d) H. Fujioka, T. Okitsu, T. Ohnaka, Y. Sawama, O. Kubo, K. Okamoto, and Y. Kita, Adv. Synth. Catal., 2007, 349, 636; CrossRef e) H. Fujioka, T. Ohnaka, T. Okitsu, O. Kubo, K. Okamoto, Y. Sawama, and Y. Kita, Heterocycles, 2007, 72, 529; CrossRef f) H. Fujioka, T. Okitsu, T. Ohnaka, R. Li, O. Kubo, K. Okamoto, Y. Sawama, and Y. Kita, J. Org. Chem., 2007, 72, 7898. CrossRef
2. Hydrazone compounds are more stable than imine compounds against water. The utility of the hydrazone compounds was demonstrated. For examples, see a) H. Takahashi, K. Tomita, and H. Otomasu, J. Chem. Soc., Chem. Commun., 1979, 668; CrossRef b) D. Enders, H. Shubert, and C. Nubling, Angew. Chem., Int. Ed. Engl., 1986, 25, 1109; CrossRef c) S. E. Denmark, T. Weber, and D. W. Piotrowsky, J. Am. Chem. Soc., 1987, 109, 2224. CrossRef