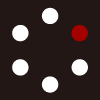
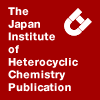
HETEROCYCLES
An International Journal for Reviews and Communications in Heterocyclic ChemistryWeb Edition ISSN: 1881-0942
Published online by The Japan Institute of Heterocyclic Chemistry
e-Journal
Full Text HTML
Received, 10th October, 2008, Accepted, 5th December, 2008, Published online, 11th December, 2008.
DOI: 10.3987/COM-08-S(D)70
■ Synthetic Studies on the Fluorinated Analogs for the Putative Oxindole-Type Metabolites of 5-Halotryptamines
Tomoya Fujiwara, Takayuki Seki, Masaru Miura, and Yoshio Takeuchi*
Graduate School of Pharmaceutical Sciences, Toyama University, 2630 Sugitani, Toyama, Toyama 930-0194, Japan
Abstract
The suitably protected precursors for direct fluorination, Nb-Boc di-protected 5-fluorotryptamine (13), Nb-acetyl-Nb-Boc protected 5-halotryptamines (15a–c), were treated with SelectfluorTM in MeCN/water in the presence of NaHCO3 to give the corresponding 3-fluorooxindoles 14 and 16a–c in good yields. Removal of the protecting groups of 14 and 16a–c produced (3,5-difluorooxindol-3-yl)ethylamine (8) and N-acetyl-(3-fluoro-5-halooxindol-3-yl)ethylamines (9a–c) in excellent yields, respectively. These compounds are potentially non-epimerizable analogs for the putative metabolites of 5-fluorotryptamine (6) and Nb-acetyl-5-halotryptamines (7a–c).INTRODUCTION
The 3-fluorooxindole derivatives 1 have received much attention as a synthetic target for development of novel medicinal agents since BMS-204532 (Maxipost, 2)1 was discovered as an effective calcium-dependent potassium channel opener. Fluorinated biomolecules and medicinals can be recognized by enzymes and receptors as well as the nonfluorinated molecules because the replacement of a hydrogen with a fluorine brings about the minimal steric alterations of the molecules.2 It should be noted that the fluorinated derivatives can be non-epimerizable analogs when the labile proton at the stereogenic center is replaced by a fluorine. Indeed we previously reported the synthesis of 3’-fluorothalidomide as a non-epimerizable analog of thalidomide.3 Since the stereoelectronic properties of fluorine are also similar to those of hydroxy group, isosteric analogs of prototype molecules can be obtained by substituting the hydroxy group with fluorine.2 Moreover, such substitution will prevent further metabolization which would be subjected otherwise.2 Thus 3-fluorooxindole derivatives 1 can be employed as mimics for both oxindoles 3 and 3-hydroxyoxindoles 4, which are often found in natural products and as metabolites of some indole-containing biomolecules. As a part of our studies on the design, synthesis, and biological evaluation of chiral fluorinated bioorganic molecules,3,4 we synthesized 3-(3-fluorooxindol-3-yl)-L-alanine (5).5 Here we report the synthesis of (3,5-difluorooxindol-3-yl)ethylamine (8) and N-acetyl-(3,5-difluorooxindol-3-yl)ethylamine (9a–c) as potential analogs for the putative oxindole-type metabolites of 5-fluorotryptamine (6) and Nb-acetyl-5-halotryptamines (7a–c), which have agonistic activities toward serotonin 5-HT3 receptor and melatonin MT1 and MT2 receptors, respectively.6 It should be noted that oxindoles 3 may epimerize under physiological conditions.7 Therefore, compounds 8 and 9a–c can be the non-epimerizable analogs, which are effective tools for investigating the relationship between the biological behavior and the stereochemistry of the metabolites.
RESULTS AND DISCUSSION
We previously reported the direct fluorination using SelectfluorTM for preparation of the tryptophan isostere 5. In this procedure we found that use of the precursor with di-protected α-amino moiety is essential for successful fluorination. For synthesis of the fluorooxindole-containing ethylamine 8, we employed Nb,Nb-di-(tert-butoxycarbonyl)tryptamine (10) as a model compound in order to examine the optimum reaction conditions for the fluorination. The suitably protected precursor 10, prepared from tryptamine according to the literature,5,8 was treated with 3 equiv of SelectfluorTM in MeCN/water (1/1) at rt for 16 –24 h9 to produce the corresponding 3-fluorooxindole 11. However, the yield of 11 was quite low owing to the formation of mono-deprotected product 12 (Table 1, entry 1). This was presumably due to the rather acidic conditions employed for the reaction. We then attempted the fluorination in the presence of a base.10 Fluorination of 10 in the presence of 3 equiv of NaHCO3 gave 3-fluorooxindole 11 in 58% yield without formation of 12 (entry 2). Use of increased or decreased amount of NaHCO3 did not give any better results (entries 3–6). Excess amount of SelectfluorTM led to increase of the yield of 11 (entry 7). The best result was obtained when the fluorination was carried out using 4 equiv of SelectfluorTM and 4 equiv of NaHCO3 to afford 11 in 73% yield (entry 7).
The 5-fluoro analog 13 was prepared from 6 according to the literature.5,8 We employed above reaction conditions for direct fluorination of 13 and the corresponding 3-fluorooxindole 14 was successfully obtained in 73% yield (Scheme 1).11 Treatment of 14 with HBr/AcOH smoothly produced the deprotected ethylamine 8 as HBr salt in excellent yield.12
We next attempted synthesis of Nb-acetyl protected fluorooxindole-containing ethylamine 9a–c. Although direct fluorination of 7b with SelectfluorTM gave 9b, the yield was unsatisfactory owing to concomitant formation of side products.13 We then focused on the Nb-di-protected structure. The precursors 15a–c, prepared in a similar manner to the synthesis of 13, were treated with SelectfluorTM in the presence of NaHCO3 to give the corresponding 3-fluorooxindoles 16a–c in good yields (Scheme 2). Removal of the Boc groups of 16a–c with HBr/AcOH successfully produced the Nb-acetyl protected fluorooxindole-containing ethylamine 9a–c in excellent yields.14
Preparation of the individual enantiomers of 8 and 9a–c is in progress for investigation of the relationship between the biological behavior and the stereochemistry.
In summary, we achieved the synthesis of fluorooxindole-containing ethylamine 8 as a metabolic analog of 6 by fluorination of the Nb-di-protected tryptamine derivative 13 with SelectfluorTM in the presence of NaHCO3 followed by deprotection. Similarly, Nb-acetyl protected fluorooxindole-containing ethylamine 9a–c were successfully synthesized as metabolic analogs of 7a–c.
References
1. P. Hewawasam, V. K. Gribkoff, Y. Pendri, S. I. Dworetzky, N. A. Meanwell, E. Martinez, C. G. Boissard, D. J. Post-Munson, J. T. Trojnacki, K. Yeleswaram, L. M. Pajor, J. Knipe, Q. Gao, R. Perrone, and J. E. Starrett, Jr., Bioorg. Med. Chem. Lett., 2002, 12, 1023. CrossRef
2. a) J. Bégué and D. Bonnet-Delpon, ‘Bioorganic and Medicinal Chemistry of Fluorine’, John Wiley & Sons, New York, 2008; b) I. Ojima, J. R. McCarthy, and J. T. Welch, ‘Biomedical Frontiers of Fluorine Chemistry’, ACS Symposium Series 639, American Chemical Society, Washington, DC, 1996; c) R. Filler and Y. Kobayashi, ‘Biomedicinal Aspects of Fluorine Chemistry’, Kodansha/Elsevier Biomedical, Tokyo, 1982.
3. Y. Takeuchi, T. Shiragami, K. Kimura, E. Suzuki, and N. Shibata, Org. Lett., 1999, 1, 1571. CrossRef
4. a) Y. Takeuchi, N. Shibata, E. Suzuki, Y. Iimura, T. Kosasa, T. Yamanishi, and H. Sugimoto, PCT Int. Appl. WO 2002020482 ; b) Chem. Abstr. 2002, 136, 247496v ; c) H. Fujisawa, T. Fujiwara, Y. Takeuchi, and K. Omata, Chem. Pharm. Bull., 2005, 53, 524; CrossRef d) Y. Takeuchi, H. Fujisawa, T. Fujiwara, M. Matsuura, H. Komatsu, S. Ueno, and T. Matsuzaki, Chem. Pharm. Bull., 2005, 53, 1062. CrossRef
5. T. Fujiwara, B. Yin, M. Jin, K. L. Kirk, and Y. Takeuchi, J. Fluorine Chem., 2008, 129, 829. CrossRef
6. a) K. S. Bower, K. L. Price, L. E. C. Sturdee, M. Dayrell, D. A. Dougherty, and S. C. R. Lummis, Eur. J. Pharmacol., 2008, 580, 291; CrossRef b) G. Spadoni, C. Balsamini, A. Bedini, A. Carey, G. Diamantini, B. D. Giacomo, A. Tontini, G. Tarzia, R. Nonno, V. Lucini, M. Pannacci, B. M. Stankov, and F. Fraschini, Med. Chem. Res., 1998, 8-9, 487.
7. a) R. S. Phillips, E. W. Miles, and L. A. Cohen, Biochemistry, 1984, 23, 6228; CrossRef b) Idem, J. Biol. Chem., 1985, 260, 14665; c) D. M. Kiick and R. S. Phillips, Biochemistry, 1988, 27, 7339; CrossRef d) M. Roy, E. W. Miles, R. S. Phillips, and M. F. Dunn, ibid., 1988, 27, 8661; CrossRef e) R. S. Phillips, S. L. Bender, P. Brzovic, and M. F. Dunn, ibid., 1990, 29, 8608; CrossRef f) R. B. Labroo and L. A. Cohen, J. Org. Chem., 1990, 55, 4901. CrossRef
8. Y. Kiso, M. Inai, K. Kitagawa, and T. Akita, Chem. Lett., 1983, 739. CrossRef
9. Y. Takeuchi, T. Tarui, and N. Shibata, Org. Lett., 2000, 2, 639. CrossRef
10. T. Umemoto, M. Nagayoshi, K. Adachi, and G. Tomizawa, J. Org. Chem., 1998, 63, 3379. CrossRef
11. General procedure for the fluorination of the protected tryptamines: SelectfluorTM (283.4 mg, 0.800 mmol) was added to a solution of Nb,Nb-di-(tert-butoxycarbonyl)-5-fluorotryptamine (13) (75.7 mg, 0.200 mmol) in a mixture of MeCN and water (1:1, 4 mL) at rt. After stirring for 20 h, the mixture was concentrated and extracted with EtOAc. The organic layer was washed with brine, dried over Na2SO4, and concentrated in vacuo. The residue was purified by silica gel column chromatography (eluent; hexane/EtOAc = 3/1) to give the corresponding 3-fluorooxindole 14 (60.5 mg, 0.147 mmol, 73%) as a colorless glass, together with the corresponding oxindole derivative (4.1 mg, 0.010 mmol, 5%) as a colorless oil: IR (KBr) ν 3430, 3315, 2979, 2933, 1759, 1734, 1701, 1676, 1631, 1487 cm-1; 1H NMR (400 MHz, CDCl3) δ 1.48 (18H, s), 2.35–2.50 (2H, m), 3.71–3.78 (2H, m), 6.88 (1H, ddd, J = 8.5, 4.1, 0.9 Hz), 7.05 (1H, tt, J = 9.2, 2.3 Hz), 7.16 (1H, dt, J = 7.3, 2.3 Hz), 8.62 (1H, br s); 19F NMR (376 MHz, CDCl3) δ –119.46 (1F, dddd, J = 9.2, 7.3, 4.1, 1.8 Hz), –159.14 (1F, t, J = 16.2 Hz); MS (EI) m/z: 412 (M+), 356 (M+–C4H8), 300 (M+–C8H16); HRMS (EI) calcd for C20H26F2N2O5 (M+): 412.1810; found 412.1794.
12. Synthesis of 8: To a solution of 14 (23.7 mg, 0.058 mmol) in AcOH (0.5 mL) was added 25% HBr/AcOH (0.5 mL) at 0 oC. After stirring at rt for 10 min, the mixture was concentrated in vacuo to give HBr salt of 8 (16.7 mg, 0.057 mmol, 99%) as a pale yellow solid: mp 136 oC (decomp.); IR (KBr) ν 3700–3100 (br), 3215, 3033, 1739, 1634, 1490 cm-1; 1H NMR (400 MHz, CD3OD) δ 2.31 (1H, dddd, J = 27.0, 14.9, 7.8, 6.9 Hz), 2.58 (1H, dddd, J = 14.9, 13.5, 7.8, 6.9 Hz), 3.29 (2H, br m), 6.94 (1H, ddd, J = 8.5, 4.1, 1.4 Hz), 7.17 (1H, dddd, J = 9.2, 8.7, 2.7, 1.8 Hz), 7.31 (1H, dt, J = 7.8, 2.7 Hz); 19F NMR (376 MHz, CD3OD) δ –119.67 (1F, dddd, J = 9.2, 7.8, 4.1, 1.8 Hz), –160.00 (1F, m); MS (FAB+) m/z: 213 (M+–Br); HRMS (FAB+) calcd for C10H11F2N2O (M+–Br): 213.0840; found 213.0854, (FAB–) calcd for C10H10BrF2N2O (M+–H): 290.9945; found 290.9942.
13. The Nb-acetyl moiety will be subjected to electrophilic fluorination thus giving unidentified oxidized products.
14. General procedure for the acid deprotection of the Boc group of 16a–c: To a solution of 16a (19.9 mg, 0.056 mmol) in AcOH (1 mL) was added 25% HBr/AcOH (1 mL) at 0 oC. After stirring for 10 min at rt, the mixture was poured into saturated aqueous NaHCO3 and then extracted with EtOAc. The organic layer was washed with brine, dried over Na2SO4, and concentrated in vacuo. The residue was purified by silica gel column chromatography (eluent; EtOAc/MeOH = 40/1) to give 9a (13.2 mg, 0.052 mmol, 93%) as a colorless solid: mp 170–172 oC; IR (KBr) ν 3441, 3353, 1732, 1722, 1653, 1636, 1554, 1489 cm-1; 1H NMR (400 MHz, CD3OD) δ 1.82 (3H, s), 2.34 (2H, dt, J = 15.1, 7.3 Hz), 3.20 (1H, dt, J = 13.7, 7.3 Hz), 3.25 (1H, dt, J = 13.7, 7.3 Hz), 6.90 (1H, dd, J = 7.8, 3.7 Hz), 7.11 (1H, tt, J = 8.7, 1.8 Hz), 7.27 (1H, dt, J = 7.8, 1.8 Hz); 19F NMR (376 MHz, CD3OD) δ –120.28 (1F, m), –155.90 (1F, t, J = 15.3 Hz); MS (EI) m/z: 254 (M+), 192 (M+–F–CH3CO); HRMS (EI) calcd for C12H12F2N2O2 (M+): 254.0867; found 254.0869.