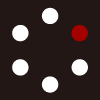
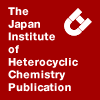
HETEROCYCLES
An International Journal for Reviews and Communications in Heterocyclic ChemistryWeb Edition ISSN: 1881-0942
Published online by The Japan Institute of Heterocyclic Chemistry
e-Journal
Full Text HTML
Received, 14th October, 2008, Accepted, 18th December, 2008, Published online, 22nd December, 2008.
DOI: 10.3987/REV-08-SR(D)9
■ 3-Methyl-2,5-dihydro-1-benzoxepins and 3-Methyl-2,5-dihydrooxepins
Seiji Yamaguchi*
Department of Chemistry, Graduate School of Science and Engineering, Toyama University, Gofuku 3190, Toyama 930-8555, Japan
Abstract
Three preparative procedures of seven-membered O-heterocyclic 3-methyl-2,5-dihydro-1-benzoxepin derivatives are summarized. For the Z-selective formation of C=C double bond, the first approach used the Grubbs ring-closing methathesis, the second approach used the intramolecular Mitsunobu cyclization of corresponding Z-diols prepared using Stille coupling of the benzyl bromide with (Z)-vinylstannane, and the third approach used the revised synthesis of the Z-diols using Z-selective Ando-Horner-Emmons condensation and the following DIBAL-H reduction. Some naturally occurring 3-methyl- 2,5-dihydro-1-benzoxepin derivatives were synthesized using these procedures. In these studies, some preparations of 3-methyl-2,5-dihydrooxepin derivatives were also developed.INTRODUCTION
Few preparative procedures of seven-membered O-heterocyclic 3-methyl-2,5-dihydro-1-benzoxepin derivatives (1) have been reported. The Z-selective formation of C=C double bond was need in the unstable position, none-conjugated with the π electron of benzene or the none-bonding electrons on the ring oxygen. The first approach was the Grubbs ring-closing methathesis for the Z-selective cyclization.1 The second approach was our method which used the intramolecular cyclization of corresponding Z-diols prepared by Stille coupling of the benzyl bromide with (Z)-vinylstannane.2 For the third approach, we revised the synthesis of the Z-diols using Z-selective Ando-Horner-Emmons condensation and the following DIBAL-H reduction.3 In these studies, some preparations of 3-methyl-2,5-dihydrooxepin derivatives (2) were also developed via the ring expansions of 2-isopropenyl- cyclopropane-1-carbaldehydes and 2-isopropenyl-2,3-dihydrofurans.
Naturally Occurring 3-Methyl-2,5-dihydro-1-benzoxepins)
Many naturally occurring 3-methyl-2,5-dihydro-1-benzoxepins (1), as shown in the structure in Figure 1, were isolated from various kinds of plants, especially from Japanese liverwort, Radula sp., as shown in Figure 2.4a-f
In 1966, D. H. A. Taylor et al.4a reported the first isolation of a naturally occurring 3-methyl- 2,5-dihydro-1-benzoxepin derivative, ptaeroxylin, from Ptaeroxylon obliquum, where the angular oxepinochromone structure of 10-hydroxy-3,7-dimethyl-2,5-dihydropyrano[2,3-g]benzoxepin- 9-one was proposed, but the structure was soon corrected to the linear oxepinochromone structure of 6-hydroxy-3,9-dimethyl-2,5-dihydropyrano[3,2-h]benzoxepin-7-one by P. H. McCabe et al.,4b where a new linear oxepinochromone karenin, 6-hydroxy-9-hydroxymethyl-3-methyl-2,5-dihydropyrano[3,2-h]- benzoxepin-7-one was isolated and the desoxykarenin was shown to be identical with ptaeroxylin. I. T. Eshiett et al.4c reported the isolation of ptaeroxylin from Cedrelopsis grevei, botanically close to Ptaeroxylon. Y. Asakawa et al.5a-f isolated many 3-methyl-2,5-dihydro-1-benzoxepin derivatives, radulanin A, B, E, H, radulanolide, and variabilin, from Japanese moss Radula sp. M. Takasugi et al.6 isolated two oxepinobenzofuran derivatives, moracin G, H, from Morus alba L. (Moraceae) infected with Fusarium solani. M. Breuer et al.7 isolated an 9-hydroxy-3-methyl-2,5-dihydro-1-benzoxepin- 7-carboxylic acid, from Hemizonia lobbii (Compositae). S. McCormick et al.8 also isolated three oxepino-flavonoids, 7-(3,4-dihydroxyphenyl)-10-hydroxy-3-methyl-2,5,7,8-tetrahydropyrano[2,3-g]- benzoxepin-9-one (7,8-dihydrooxepinoeriodictyol), 8,10-dihydroxy-7-(3,4-dihydroxyphenyl)-3-methyl- 2,5,7,8-tetrahydropyrano[2,3-g]benzoxepin-9-one (7,8-dihydrooxepinodihydroqurcetin), 1-(6,8- dihydroxy-3-methyl-1-benzoxepin-7-yl)-3-(3,4-dihydroxyphenyl)propa-2-en-1-one (3’,4’-dihydro- oxepino- 6’-hydroxybutein), from Wyethia sp. (Compositae).
They might be biogenetically derived from o-prenylphenols (5); an oxidative seven-membered cyclization gives corresponding 3-methyl-2,5-dihydro-1-benzoxepins (1); while a similar five- or six-membered cyclization gives corresponding 2-isopropenyl-2,3-dihydrobenzofurans (4) or 2,2-dimethyl- 2H-chromenes (3). Because most of the benzene ring of naturally occurring 3-methyl-2,5-dihydro- 1-benzoxepins (1) might be derived via polyketides derived from acetyl coenzyme A, most of naturally occurring 3-methyl-2,5-dihydro-1-benzoxepins (1) have some oxygen-functions on the benzene ring, especially on 6 and/or 8 position. For the synthesis of naturally occurring 3-methyl- 2,5-dihydro-1-benzoxepins (1), the effective preparative methods of 3-methyl-2,5-dihydro-1-benzoxepins (1), especillay methoxy-substituted 3-methyl-2,5-dihydro-1-benzoxepins (1b-e) were needed.
RESULTS AND DISCUSSION
1 REPORTED APPROACHES FOR 3-METHY-2,5-DIHYDRO-1-BENZOXEPINS
1-1 DEHYDRATION OF 3-METHYL-2,3,4,5-TETRAHYDRO-1-BENZOXEPIN-3-OL
In 1980, K. Baird et al.9 first reported the formation of seven-membered 3-methyl-2,5-dihydro- 1-benzoxepin by dehydration of 3-methyl-2,3,4,5-tetrahydro-1-benzoxepin-3-ol (8), a minor cyclized product from alkaline cyclization of o-(3,4-epoxy-3-methylbutyl)phenol (6), which gave an unseparable mixture of 3-methyl-2,5-dihydro-1-benzoxepin (1a), 3-methyl-4,5-dihydro-1-benzoxepin (9), and 3-methylene-2,3,4,5-tetrahydro-1-benzoxepin (10) in dehydration using thionyl chloride in pyridine.
1-2 PHOTO-RING EXPANSION OF 2,2-DIMETHYL-2H-CHROMENES AND
2-ISOPROPENYL-2,3-DIHYDROBENZOFURANS
The second formation was the photochemical ring-expansion of 2,2-dimethyl-2H-chromene derivatives, reported by A. Chakrabarti et al.,10 and F. Dallacker et al.11; the former reported the photochemical ring-expansion of some 3,3,5-trimethylpyrano[2,3-a]carbazoles giving corresponding 3,6-dimethyl- 1,4-dihydroxepino[2,3-a]carbazoles and the latter reported the ring-expansion of 6-methoxy- 2,2,5,7,8-pentamethyl-2H-chromene giving 7-methoxy-3,6,8,9-tetramethyl-2,5-dihydro-1-benzoxepin. We also observed the similar photochemical ring-expansion of 6-methoxy- 2,2-dimethyl-2H-chromene (3c) and also of 5-methoxy-2-isopropenyl-2,3-dihydrobenzofuran (4c) giving 7-methoxy-3-methyl- 2,5-dihydro-1-benzoxepin (1c).12 However, these photochemical ring-expansions of six-membered 2,2-dimethyl-2H-chromene or five-membered 2-isopropenyl-2,3-dihydrobenzofuran derivatives were found to be restricted in compounds having an electron donating group at position 6 in 2H-chromenes or at position 5 in dihydrobenzofurans. A. Chakrabarti et al.10 and F. Dallacker et al.11 proposed the mechanism of the ring-expansions mechanism via a quinine-dimethylallid (11) in their papers, but there were no descriptions about the necessity of the electron donating methoxy substituent. So, we proposed another ring expansion mechanism starting the cleavage of the C-O bond. The electron donating substituent might accelerate the C-O bond cleavage. In 2-isopropenyl-2,3-dihydrobenzofuran 4c, the 5-methoxy substituent accelerates the furan-ring C-O bond cleavage, and the following [1,3] sigmatropy might lead to to the corresponding benzoxepin 1c. In 2,2-dimethyl-2H-chromene 3c, the 6-methoxy substituent accelerates the pyran-ring C-O bond cleavage, and might cause valence isomerization to 12 and [1,3] sigmatropy giving an isopropenylcyclopropane intermediate 13, and the following [3,3] sigmatropy might lead to the benzoxepin 1c.
1-3 ONE-STEP SEVEN-MEMBERED CYCLIZATION
The third formation was the one-step cyclization of 2,6-dihydroxyacetophenone or 2,4,6-trihydroxy- acetophenone with 1,4-dibromo-2-methylbut-2(E)-ene giving a separable mixture of corresponding seven-membered 9-acetyl-3-methyl-2,5-dihydro-1-benzoxepin-8-ols (1f,g) and five-membered 7-acetyl- 2-isopropenyl-2,3-dihydrobenzofuran-6-ols (4f,g).13a,b In these cyclizations, very interestingly, considerable amounts of seven-membered cyclization, having a Z double bond, were obtained from E-1,4-dibromo-2-butene (14). So, we proposed the mechanism, via [3,3] sigmatropy of isopropenylcyclopropane derivatives (16c-f), formed by double C-alkylation. However, these one-step cyclizations were also founded to be restricted in compounds having two hydroxyl groups at position 2 and 6 in acetophenones.
Inspite of the early isolation, only few synthetic reports might be due to the synthetic difficulties of the formation of the Z C=C double bond in the unstable seven-membered O-heterocyclic ring and the unstable 3,4-position, having no conjugation with lone-paired electrons on the ring oxygen or with π-electrons of the benzene ring.
2 RING EXPANSIONS FOR 3-METHYL-2,5-DIHYDROOXEPINS
2-1 RING EXPANSION OF 2-ISOPROPENYLCYCLOPROPANE-1-CARBALDEHYDE
In our one-step cyclization, described above, we studied a new ring expansion via isopropenylcyclopropane intermediate (16).14 To prove the mechanism, an isopropenylcyclopropane intermediate, dimethyl 2-isopropenylcyclopropane-1,1-dicarboxylate (17a) was prepared from dimethyl malonate and 1,4-dibromeo-2-methyl-2(E)-butene (14). The conversion of dicarboxylate 17a to the corresponding dialdehyde (19a) was studied by LiAlH4 reduction giving dimethanol (18a) followed by Swern oxidation. Swern oxidation of dimethanol 18a did not give the corresponding dialdehyde 19a, but readily caused the ring expansion to give the corresponding seven-membered 6-methyl- 4,7-dihydrooxepin-3-carbaldehyde (2a).
A DIBAL reduction of dicarboxylate 17a gave a mixture of two stereoisomeric hydroxymethyl- carboxylates (18b,c). Swern oxidation of the isomer 18b caused ring-expansion to give a seven-membered methyl 6-methyl-4,7-dihydrooxepin-3-carboxylate (2b), while Swern oxidation of the isomer 18c gave a three-membered methyl 2-isopropenyl-1-formylcyclopropane-1-carboxylate (19c). These might show the relation between the isopropenyl group and the hydroxymethyl was cis in the isomer 18b and was trans in the isomer 18c.
Similar reaction of methyl cyanoacetate with 1,4-dibromo-2-methyl-2(E)-butene (14) gave a single isomeric methyl 2-isopropenyl-1-cyanocyclopropane-1-carboxylate (17d). DIBAL reduction of 17d gave a corresponding cyanomethanol (18d), and the following Swern oxidation gave a three-membered cyanoaldehyde 19d. This might show that the aldehyde in the isomer 19d, the methoxycarbonyl in 17d, and the hydroxymethyl in 18d, were all trans to the isopropenyl group. Also, the photoisomerization of three-membered cyanoaldehyde 19d readily caused the cis-trans isomerization to give the corresponding seven-membered 6-methyl-4,7-dihydrooxepin-3-carbonitrile (2d) via 19e.
2-2 RING EXPANSIONS OF ISOPROPENYLDIHYDROFURANCARBOXYLIC ACIDS
The similar reaction of diethyl oxaloacetate and 1,4-dibromo-2-methyl-2(E)-butene (14) caused a five-membered cyclization to give diethyl 5-isopropenyl-4,5-dihydrofuran-2,3-dicarboxylate (20f).15a,b Thermal alkaline hydrolysis of dicarboxylate 20f caused the decomposition of the furan–ring. But, mild alkaline hydrolysis of 20f using excess amount of 5% aqueous sodium hydroxide solution gave a corresponding dicarboxylic acid (21f), and another mild alkaline hydrolysis of 20f using an equivalent amount of 1.4% aqueous sodium hydroxide solution gave a single half-ester, 3-ethoxycarbonyl- 5-isopropenyl-4,5-dihydrofuran-2-carboxylic acid (20g), which was converted to the corresponding amide carboxylic acid, 2-carbamoyl-5-isopropenyl-4,5-dihydrofuran-3-carboxylic acid (21h) via a corresponding amide-ester ethyl 2-carbamoyl-5-isopropenyl-4,5-dihydrofuran-3-carboxylate (20h).
The regio-selective hydrolysis of 2-ester in diester 20f was explained as follows. The 3-ester was deactivated by conjugation with the lone-paired electrons on the oxygen in the furan ring, and, in IR spectrum, shows the 3-carbonyl lower (1710 cm-1) and the 2-carbonyl higher (1750 cm-1).
The LiBH4 reduction of diester 20f also caused a regio-selective reduction to give a single hydroxymethyl-ester, ethyl 2-hydroxymethyl-5-isopropenyl-4,5-dihydrofuran-3-carboxylate (20i), which was converted to the corresponding hydroxymethyl carboxylic acid, 2-hydroxymethyl-5-isopropenyl- 4,5-dihydrofuran-3-carboxylic acid (21i). The Grignard alkylation of diester 20f with methylmagnesium iodide also caused a regio-selective alkylation to give ethyl 2-(1-hydroxy-1-methylethyl)- 5-isopropenyl-4,5-dihydrofuran-3-carboxylate (20k), which was converted to the corresponding hydroxymethyl carboxylic acid, 2-(1-hydroxy-1-methylethyl)-5-isopropenyl-4,5-dihydrofuran- 3-carboxylic acid (21k). The Grignard alkylation of diester 20f with methylmagnesium iodide at low temperature caused a regio-selective mono-alkylation to give ethyl 2-acetyl-5-isopropenyl- 4,5-dihydrofuran-3-carboxylate (20n), which was reduced to ethyl 2-(1-hydroxyethyl)- 5-isopropenyl-4,5-dihydrofuran-3-carboxylate (20j). The 2-(1-hydroxyethyl)-3-carboxylate (20j) was also prepared from 20i by Swern oxidation giving ethyl 2-formyl-5-isopropenyl- 4,5-dihydrofuran-3-carboxylate (20m) and following mild-methylation with methylzinc bromide. The ethyl 2-(1-hydroxyethyl)-3-carboxylate (20j), thus obtained, was hydrolyzed to the corresponding hydroxymethyl carboxylic acid, 2-(1-hydroxyethyl)-5-isopropenyl-4,5-dihydrofuran-3-carboxylic acid (21j).
The furandicarboxylic acid (21f) was subjected to the conversion to the corresponding 5-isopropenyl- 4,7-dihydrofuran-2,3-dicarboxic anhydride (22f) in usual methods, but treating 21f with acetic anhydride under mild heating at 72 oC did not give isopropenyldihydrofuran anhydride 22f, but caused the ring expansion to give 6-methyl-4,7-dihydrooxepin-2,3-dicarboxylic anhydride (2f).13 The similar ring expansion was observed in 2-carbamoyl-3-carboxylic acid 21h; treatment of 21h with refluxing thionyl chloride did not give a corresponding imide, 5-isopropenyl-4,7-dihydrofuran-2,3-dicarboximide (22h), but caused the ring expansion to give 6-methyl-4,7-dihydrooxepin-2,3-dicarboxylimide (2h). These ring expansions might due to the ring strain of the fused two five-membered rings, and the ring strain caused the heterolytic cleavage of the C5-O bond in the dihydrofuran ring to give a stable enolate cation, where the enolated anion stabilized with the electron withdrawing group in position 3 and the cation also stabilized by conjugation with isopropenyl, and the stable recyclization formed the corresponding seven-membered dihydrooxepins 2f,h.
The five-membered lactone ring formation of three 2-hydroxymethylfuran-3-carboxylic acids 21i,j,k were similarly attempted.15b The lactone ring formation of 21i,j,k with dicyclohexylcarbodimide (DCC) at room temperature did not cause the ring expansion to give corresponding five-membered lactones 22i,j,k, which readily caused ring expansion by heating at 100 oC to give the corresponding seven-membered dihydrooxepins 2i,j,k.
3 EFFECTIVE APPROACH FOR 3-METHYL-2,5-DIHYDRO-1-BENZOXEPINS
3-1 Ring Closing Metathesis
In 1998, M. Stefinovic et al.1 reported the first effective preparation of 3-methyl-2,5-dihydro- 1-benzoxepin derivatives; where they used a Grubbs ring closing metathesis for Z-selective C=C bond formation. They reported the synthesis of natural radulanin A,B (1h,i) via this ring closing methathesis of corresponding o-allylphenol 2-methylallylethers (23h,i).
3-2 STILLE COUPLING & MITSUNOBU CYCLIZATION
We reported another preparative procedure;2a where we used a Stille coupling of the benzyl bromide (26), prepared from the corresponding salicyl aldehydes (24), with 2-methylprop-1(Z)-enyl trimethylstanane (27), prepared by regio- and stereo-selective methylation of propargyl alcohol with methylmagnesium bromide followed by treatment with iodine, dihydropyran, and trimethyltin chloride, and the intramolecular Mitsunobu cyclizations of the Z-diols (29), prepared by deprotection of the Stille coupling products, effectively gave corresponding 3-methyl-2,5-dihydro-1-benzoxepins (1).
As shown in Scheme 8b, we reported the synthesis of natural radulanin A,B (1h,i), using this Stille coupling and following Mitsunobu intramolecular cyclization.2a,b The starting material, 3,5-dimethoxy- 1-(2-phenylethyl)benzene (30), prepared from 3,5-dimethoxybenzaldehyde via Wittig condensation with benzyltriphenylphosphonium bromide and following hydrogenation, was converted to 2,6-dimethoxy-4-phenylethylbenzaldehyde (30) via lithiation with n-butyllithium and following formylation with dry DMF. The dimethoxybenzaldehyde 24a was converted to the salicyl aldehydes (24h,i), respectively; the demethylation by refluxing with magnesium iodide diethyl etherate in dry benzene caused mono-demethylation to give 24h, while the demethylation with boron tribromide at -78 oC caused di-demethylation to give 24i. The salicylaldehydes 24h,i, thus obtained, were converted to the corresponding benzyl bromides (26h,k), via MOM protection, reduction, and bromination, respectively. Stille coupling of the benzyl bromides 26h,k with the vinyl stananne 27 using Pd(II) and Ph3As gave the corresponding coupling products (28h,k), which were then deprotected to the corresponding (Z)-diols (29h,i). Also, the intramolecular Mitsunobu cyclizations of the (Z)-diols (29h,i) effectively gave the corresponding 3-methyl-2,5-dihydro-1-benzoxepins, radulanin A (1i) and radulanin B (1h).
As shown in Scheme 8c, we also reported the synthesis of two naturally occurring benzoxepincarboxylic acids (1l,p) using this Stille coupling and following Mitsunobu intramolecular cyclization.2b
Radulanin E, 6-hydroxy-3-methyl-8-phenylethyl-2,5-dihydro-1-benzoxepin-9-carboxylic acid (1l) was synthesized via Vielsmeier formylation of 6-methoxymethyloxy-3-methyl-8-phenylethyl-2,5-dihydro- 1-benzoxepin (1k). The 6-methoxymethyloxy derivative 1k was prepared from radulanin A (1i) by protection with MOMCl. Vilsmeier formylation of 1k treating with DMF-POCl3 gave 9-carbaldehyde, which was oxidized to 6-hydroxy-3-methyl-8-phenylethyl-2,5-dihydro-1-benzoxepin-9-carboxylic acid (1l) treating with Ag2O.
Synthesis of naturally occurring 9-hydroxy-3-methyl-2,5-dihydro-1-benzoxepin-7-carboxylic acid (1m) was first planned via similar formylation of 3-methyl-2,5-dihydro-1-benzoxepin-9-ol (1n) via MOM protection and following Vielsmeier formylation.
2,3-Di(MOMoxy)benzaldehyde 24q, prepared from di-MOM protected catechol by lithiation followed by formylation with DMF, was converted to the corresponding benzyl bromide (26q) by reduction with NaBH4 followed by bromination of the benzyl alcohol (25q) with NBS-Ph3P. Stille coupling of the benzyl bromide (26q) with vinyl stananne 27 using Pd(II) and Ph3As gave the corresponding coupling products (28q), which were then converted to the corresponding 3-methyl-2,5-dihydro-1-benzoxepin-9-ol (1n) via deprotection and following Mitsunobu cyclization, and then converted to 1o by MOM protection. However, Vilsmeier formylation of 1o or 1e did not cause any formylation and recovered the starting material.
Then, synthesis of 1m was planned in another procedure. The corresponding 5-formylbenzyl bromide (26s), was prepared from 5-bromo-2,3-dihydroxybenzaldehyde via 5-bromo-2,3-di(MOMoxy)- benzyl alcohol 27n. The bromobenzyl alcohol 25r was prepared by di-MOM protection of 5-bromo-2,3-dihydroxybenzaldehyde with MOMCl giving 24r followed by reduction with NaBH4. The bromobenzyl alcohol 25r, thus obtained, was then converted to 5-formylbenzyl bromide 26s by 1) formylation via lithiation with n-butyllithium giving the 5-lithio derivative, 2) treatment with dry DMF giving 25s, bromination with NBS-Ph3P. Stille coupling of the benzyl bromide (26s) with vinyl stananne 27 gave the corresponding coupling products (28s), which were then converted to the corresponding Z-diol (29p) by deprotection. Mitsunobu cyclization of diol 29p gave 9-hydroxy-7-carbaldehyde (1p), which was readily oxidized to 9-hydroxy-3-methyl-2,5-dihydro- 1-benzoxepin-7-carboxylic acid (1m) with Ag2O.
3-3 ANDO-HORNER-EMMONS CONDENSATION & MITSUNOBU CYCLIZATION
Recently, as shown in Scheme 9a, we found another preparative procedure of the Z-diols (29) starting from o-allylphenols (30); where the Ando-Horner-Emmons condensations of phenylacetaldehydes (31) with ethyl α-(diphenylphosphono)propionate (32) were used for a Z-selective C=C bond formation giving Z-conjugated ester (33), and the following DIBAL-H reductions effectively gave corresponding Z-diols (29).
So, the synthesis of naturally occurring radulanin H (1t) and moracin G (1u), were planned using this Ando coupling and following reduction and Mitsunobu intramolecular cyclization, as shown in Scheme 9b.3 For both naturally occurring benzoxepins 1t and 1u, 3-methyl-2,5-dihydro-1-benzoxepin- 7-methanols (1v,w) might be key intermediates, and they might be prepared by Ando coupling of the corresponding phenylacetaldehydes 31v,w with 32, followed by DIBAL reductions and Mitsunobu cyclizations.
The phenylacetaldehydes 31v,w for moracin G and radulanin H were prepared from corresponding 2,4-dihydroxybenzaldehydes, as shown in Scheme 9c, via allylation giving 3-allyl-2,4-dihydroxy- benzaldehyde, selective protections, LiAlH4 reduction, O-acetylation giving 30v,w, and following ozone cleavage. The Ando condensation of phenylacetaldehydes 31v,w with reagent 32 gave corresponding 2(Z)-conjugated butenoates 33v,w, which were then reduced to the corresponding (Z)-diols 29v,w by DIBAL reduction followed by deprotections with TBAF. In addition, the intramolecular Mitsunobu cyclizations of the (Z)-diols 29v,w effectively gave 6-methoxymethyloxy-3-methyl-2,5-dihydro- 1-benzoxepin-7-methanols (1v,w).
Conversion of 1w to the corresponding 6-hydoxy-7-carbaldehyde 1x was effective by oxidation with PDC followed by deprotection with concd. HCl in methanol, and the aldehyde 1x was converted to naturally occurring radulanin H (1t) by the mild oxidation with sodium chlorate.17
Oxidation of 1v with PDC effectively gave corresponding aldehyde, 6-(MOMoxy)-3-methyl- 2,5-dihydro-1-benzoxepin-7-carbaldehyde (1y). Also, the oxepin-7-carbaldehyde 1y might be convertible to maracin G (1u) via Wittig condensation and following furan-ring formation and deprotection.3
4 ACYLATIONS OF 3-METHYL-2,5-DIHYDRO-1-BENZOXEPINS
We already reported the acylations of 2-isopropenyl-2,3-dihydrobenzofurans,18 also labile to the acids, and found that Vilsmeier condition were successful for their formylation and an acylation mixture of acetic acid-trifluoroacetic anhydride were also successful for their C-acetylation. The seven-membered 3-methyl-2,5-dihydro-1-benzoxepins (1a-e) were supposed to be labile to the acidic condition, and the acylations of 3-methyl-2,5-dihydro-1-benzoxepins (1a-e) were studied in the similar condition; (formylation) dry N,N-dimethylformamide-phosphoryl chloride at 90 oC for 1 h; (C-acetylation) acetic acid-trifluoroacetic ahydride at room temperature for 6 hr, and the results of formylation and C-acetylation were summarized in Scheme 10a and 10b, respectively.19
4-1 VILSMEIER FORMYLATION
In Vilsmeier formylations, 6-methoxy derivative 1b, only showed the formylation at position 9 to give 6-metyhoxy-3-methyl-2,5-dihydro-1-benzoxepin-9-carbaldehyde (1b-9F) in 51% yield, and the structure was confirmed both with the ortho coupling (J = 8.8 Hz) of aromatic protons and with +7% NOE effect of a 7-H signal on irradiation at a 6-MeO signal. Other derivative, none-methoxy 1a, 7-methoxy 1c, 8-methoxy 1d, and 9-methoxy 1e, showed the recovery of the starting materials. Thus, the 3-methyl-2,5-dihydro-1-benzoxepins showed the poorer reactivities than the corresponding 2-isopropenyl-2,3-dihydrobenzofurans.
4-2 C-ACETYLATIONS
In the C-acetylations, three methoxy derivatives 1b,d,e showed the C-acetylation, but in poor yields. The acetylation of 8-methoxy derivative 1d with 3 eq. AcOH-(CF3CO)2O for 2 hr showed the 7-acetylation, ortho to the methoxy-substituent and para to the ring oxygen, to give 1d-7A in 29%. The acetylation of 9-methoxy derivative 1e in a similar condition (3 eq. acylating mixture for 2 hr) showed both acetylation, para to the methoxy-substituent or to the ring oxygen, to give a mixture of 6-acetylated 1e-6A (9.8%) and 7-acetylated 1e-7A (3.2%), but the yields were very low.
Interestingly, the acetylation of 6-methoxy derivative 1b with 3 eq. acylating mixture for 30 min gave a mixture of two acetylated products, 7-acetyl-6-methoxy-3-methyl-2,5-dihydro-1-benzoxepin (1b-7A), 7-acetyl-2-isopropenyl-2,3-dihydrobenzofuran (1b-9A*) in 1.6% and 10.4% yields, respectively. In addition, the similar acetylation of 1b with an equimoler acylating mixture for 30 min, gave a mixture of 1b-7A, 9-acetyl-6-methoxy-3-methyl-2,5-dihydro-1-benzoxepin (1b-9A), and 1b-9A* in 1.6%, 5.1%, and 1.3% yields, respectively. These showed the both acetylation, ortho/para to the methoxy-substituent and to the ring oxygen, to give a mixture of 7-acetylated 1b-7A and 9-acetylated 1b-9A, and only the latter isomer might cause the ring contraction to give 7-acetylbenzofuran 1b-9A*.
This ring contraction, selective for 1b-9A, might be explained as following, the protonation on the ring oxygen of 1b-9A caused the ring cleavage to give the transition state T, stabilized the hydrogen bonding with the acetyl, and then caused recyclization to give five-membered 1b-9A*.
Thus, trifluoroacetic acid, derived from trifluoroacetic anhydride in C-acetylations, is a stronger acid than phosphoryl chloride, used in formylation. The acetylation of three methoxy- substituted derivatives (1b,d,e) shows better reactivities than the formylation. But, the stronger acidic conditions sometimes caused the decomposition or the isomerization (ring contraction), and the lower reaction temperature (rt) leads to the poorer yields.
References
1. M. Stefinovic and V. Snieckus, J. Org. Chem., 1998, 63, 2808. CrossRef
2. a) S. Yamaguchi, K. Furihata, M. Miyazawa, and Y. Hirai, Tetrahedron Lett., 2000, 41, 4787; CrossRef b) S. Yamaguchi, K. Furihata, M. Miyazawa, and Y. Hirai, J. Org. Chem., 2006, 43, 657.
3. S. Yamaguchi, X. Wuu, M. Miyazawa, and Y. Hirai, , the details will be reported soon.
4. a) F. M. Dean and D. A. H. Taylor, J. Chem. Soc. (C), 1966, 114; CrossRef b) P. H. McCabe, R. McCrindle, and R. D. H. Murray, J. Chem. Soc. (C), 1967, 145; CrossRef c) I. T. Eshiett and D. A. H. Taylor, J. Chem. Soc. (C), 1968, 481. CrossRef
5. a) Y. Asakawa, M. Toyota, and T. Takemoto, Phytochemistry, 1978, 17, 2005; CrossRef b) Y. Asakawa, E. Kusube, T. Takemoto, and C. Suire, Phytochemistry, 1978, 17, 2115; CrossRef c) Y. Asakawa, R. Takeda, M. Toyota, and T. Takemoto, Phytochemistry, 1981, 20, 858; CrossRef d) Y. Asakawa, K. Takikawa, M. Toyota, and T. Takemoto, Phytochemistry, 1982, 21, 2481; CrossRef e) Y. Asakawa, T. Hashimoto, K. Takikawa, M. Tori, and S. Ogawa, Phytochemistry, 1991, 30, 235; CrossRef f) Y. Asakawa, K. Kondo, and M. Tori, Phytochemistry, 1991, 30, 325. CrossRef
6. M. Takasugi, S. Nagao, T. Masamune, A. Shirata, and K. Takahashi, Tetrahedron Lett., 1979, 48, 4675. CrossRef
7. M. Breuer, G. Leeder, P. Proksch, and H. Budzikiewicz, Phytochemistry, 1986, 25, 495. CrossRef
8. S. McCormick, K. Robson, and B. Bohm, Phytochemistry, 1986, 25, 1723. CrossRef
9. K. J. Baird and M. F. Grundon, J. Chem. Soc., Perkin Trans. 1, 1980, 1820. CrossRef
10. A. Chakrabarti and D. P. Chakraborty, Tetrahedron Lett., 1988, 29, 6625. CrossRef
11. F. Dallacker and K. Reperich, Chem. Zeit., 1991, 115, 306.
12. Private Report in our Laboratory.
13. a) S. Yamaguchi, A. Saitoh, and Y. Kawase, Bull. Chem. Soc. Jpn., 1986, 59, 3983; CrossRef b) S. Yamaguchi, M. Takai, I. Hanazome, Y. Okada, and Y. Kawase, Bull. Chem. Soc. Jpn., 1987, 60, 3603. CrossRef
14. S. Yamaguchi, A. Arisawa, N. Katoh, C. Hatanaka, H. Yokoyama, and Y. Hirai, Bull. Chem. Soc. Jpn., 1997, 70, 2215. CrossRef
15. a) S. Yamaguchi, Y. Sugioka, M. Ishida, H. Yokoyama, and Y. Hirai, J. Heterocycl. Chem., 1997, 34, , 1329; CrossRef b) S. Yamaguchi, N. Tsuchida, K. Umeda, H. Yokoyama, M. Miyazawa, and Y. Hirai, J. Heterocycl. Chem., 2006, 43, 657. CrossRef
16. S. Yamaguchi, Y. Sugioka, Y. Kitagawa, Y. Matsumoto, H. Yokoyama, and Y. Hirai, Bull. Chem. Soc. Jpn., 1997, 70, 2777. CrossRef
17. A similar mild oxidation of the aldehyde 1x with Ag2O caused the cleavage of the seven-membered oxepin ring .
18. S. Yamaguchi, A. Miyata, M. Ueno, T. Hase, K. Yamamoto, and Y. Kawase, Bull. Chem. Soc. Jpn., 1984, 57, 617. CrossRef
19. S. Yamaguchi, Y. Hirasaki, and M. Omote, J. Heterocycl. Chem., 2009, 40, in press.