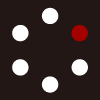
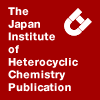
HETEROCYCLES
An International Journal for Reviews and Communications in Heterocyclic ChemistryWeb Edition ISSN: 1881-0942
Published online by The Japan Institute of Heterocyclic Chemistry
e-Journal
Full Text HTML
Received, 10th April, 2008, Accepted, 15th May, 2008, Published online, 19th May, 2008.
DOI: 10.3987/COM-08-S(F)11
■ Enantioselective Synthesis of a 3,5,5-Trialkylated Tetronic Acid Derivative
Ken-ichi Takao,* Yuki Kojima, Tomo Miyashita, Kentaro Yashiro, Tatsuya Yamada, and Kin-ichi Tadano*
Department of Applied Chemistry, Faculty of Science and Technology, Keio University, Hiyoshi, Kohoku-ku Yokohama 223-8522, Japan
Abstract
A convenient and enantioselective method for the synthesis of 3,5,5-trialkylated tetronic acid has been developed. The Dieckmann-type condensation of enantioenriched α-acyloxy-α,α-dialkylated acetic acid ester (11) proceeded in the presence of lithium hexamethyldisilazide, providing the expected tetronic acid derivative (12) after quenching with methoxymethyl chloride. The product (12) was converted into a doubly prenylated tetronic acid derivative (13), which constitutes a substructure of perforatumone, a newly isolated polycyclic polyprenylated acylphloroglucinol-type natural product.3,5,5-Trialkylated tetronic acids depicted by the general structures (1 and 2) (Figure 1) are abundantly found in nature, and many of them exhibit a wide range of biological properties such as antimicrobial, antitumor, anticoagulant, antiepileptic, antifungal, insecticidal, analgesic, and anti-inflammatory activities.1 Densely functionalized polyprenylated tetronic acids have recently been isolated from plants, especially those found in the family of Guttiferae. The research group of the Kao Corporation isolated two polyprenylated tetronic acids, erectumins A and B (3), from Hypericum erectum (Guttiferae) as a vasodilator and a skin medicine, respectively, for external use.2 A more structurally functionalized tetronic acid-based compound, perforatumone (4), was recently isolated from the aerial part of Hypericum perforatum (St. John’s wort) by Harrison and co-workers.3 This natural product (4) is regarded to be a structural variant of polycyclic polyprenylated acylphloroglucinols (PPAPs), a representative of which is the antidepressant hyperforin.4 The structure of 4 was characterized by its rare 7-oxabicyclo[4.2.1]nonane-8,9-dione skeleton. In recent years, a number of research groups have focused on the chemical synthesis of PPAPs because of their synthetically formidable structures and wide variety of biological activities.5 To date, only one synthetic attempt on 4 has been reported.6 We have been involved in the enantioselective total synthesis of 4, and our initial efforts in this area have focused on the practical synthesis of 3,5,5-trialkylated tetronic acids as advanced synthetic intermediates toward 4. Herein, we describe the enantioselective synthesis of an O-protected 3,5,5-trisubstituted tetronic acid derivative such as 13, featured by the Sharpless asymmetric epoxidation protocol to introduce the C-5 chirality and also by Dieckmann-type condensation to form the tetronic acid skeleton.
Although a number of synthetic approaches toward functionalized tetronic acids have been reported,7 we envisioned that the tetronic acid equivalent of 4, simplified as structural formula (A) in Figure 2, would be synthesized via the Dieckmann-type condensation8 of α-acyloxy-α,α-dialkylated acetic acid ester (B) and the subsequent exchange of the allyl groups at C3 and C5 for prenyl groups by cross-metathesis. This ester (B) for the attempted Dieckmann-type reaction, in turn, could be prepared from an enantioenriched α,α-differentially substituted chiral epoxide (C) through the epoxy-ring opening with a vinyl nucleophile, oxidation of one hydroxymethyl group into a methoxycarbonyl group, and acylation of the resulting tertiary alcohol with 4-pentenoic acid.
The synthesis of 13 began with the Sharpless asymmetric epoxidation of known allylic alcohol (5)9 (Scheme 1). Treatment of 5 with tert-butyl hydroperoxide in the presence of catalytic amounts of (–)-diisopropyl tartrate (DIPT) (10 mol %) and titanium tetraisopropoxide (8 mol %) provided α,α- disubstituted epoxide (6)10,11 in high yield and excellent enantiomeric excess (98% ee).12 The hydroxy group in 6 was protected as tetrahydropyranyl (THP) ether, providing 7. The epoxy-ring opening of 7 with vinylmagnesium bromide in the presence of copper iodide occurred regioselectively to furnish homoallylic alcohol (8) as a single product.13 To minimize halohydrin formation and to maintain a high-yielding reaction, it was essential to use freshly prepared Grignard reagent. Removal of the tert-butyldimethysilyl (TBS) group in 8 provided diol (9), which was converted into hydroxy-ester (10) by two-step oxidation and the subsequent esterification of the resulting α,α-disubstituted glycolic acid. Acylation of the tertiary hydroxy group in 10 was well achieved by using sodium bis(trimethylsilyl)amide (NaHMDS) (1.2 equiv) and 4-pentenoyl chloride (1.5 equiv) to provide acyloxy-ester (11) in 70% yield and 28% of 10 was recovered. When tert-butyllithium or lithium bis(trimethylsilyl)amide (LiHMDS) was used as the base, the yield of 11 diminished significantly (47% or 39%). To produce the desired tetronic acid derivative, the Dieckmann-type condensation of 11 was executed by treatment with LiHMDS (1.5 equiv).8 Trapping the intermediary enolate with methoxymethyl chloride (MOMCl) led to the corresponding MOM enol ether (12) in a good yield of 88%.14 The two allyl groups in 12 were simultaneously exchanged with both prenyl groups by the cross-metathesis of 12 with 2-methyl-2-butene15 using the second-generation Grubbs catalyst16 to afford 13 in almost quantitative yield.17
In conclusion, we have developed a novel method for the enantioselective synthesis of a 3,5,5-trialkylated tetronic acid derivative such as 13. The present approach realizes access to the tetronic acid moiety of perforatumone (4). Further studies toward the total synthesis of 4 are in progress in this laboratory.
ACKNOWLEDGEMENTS
K. Takao thanks the Amano Institute of Technology for financial support.
‡ This paper is dedicated to Professor Emeritus Keiichiro Fukumoto, with respect and admiration, on the occasion of his 75th birthday.
References
1. F. Effenberger and J. Syed, Tetrahedron: Asymmetry, 1998, 9, 817 and references cited therein. CrossRef
2. Y. Jokura, T. Kamiya, and Y. Nishizawa, Jpn. Kokai Tokkyo Koho, 1992, JP 04211676 (Chem. Abstr., 1992, 118, 45729).
3. J. Wu, X.-F. Cheng, L. J. Harrison, S.-H. Goh, and K.-Y. Sim, Tetrahedron Lett., 2004, 45, 9657. CrossRef
4. N. S. Bystrov, B. K. Chernov, V. N. Dobrynin, and M. N. Kolosov, Tetrahedron Lett., 1975, 16, 2791. CrossRef
5. R. Ciochina and R. B. Grossman, Chem. Rev., 2006, 106, 3963. CrossRef
6. K. C. Nicolaou, G. E. A. Carenzi, and V. Jeso, Angew. Chem. Int. Ed., 2005, 44, 3895. CrossRef
7. A. L. Zografos and D. Georgiadis, Synthesis, 2006, 3157. CrossRef
8. For precedents to the utility of Dieckmann-type condensation for the construction of the tetronic acid skeleton, see: (a) R. E. Ireland and W. J. Thompson, J. Org. Chem., 1979, 44, 3041; CrossRef (b) W. R. Roush and R. J. Sciotti, J. Am. Chem. Soc., 1998, 120, 7411. CrossRef
9. (a) S. Brass, H.-D. Gerber, S. Dörr, and W. E. Diederich, Tetrahedron, 2006, 62, 1777; CrossRef (b) S. J. Danishefsky and N. Mantlo, J. Am. Chem. Soc., 1988, 110, 8129. CrossRef
10. For the synthesis of the enantiomer of 6 by enzymatic reactions, see: (a) Y.-B. Seu, T.-K. Lim, C.-J. Kim, and S.-C. Kang, Tetrahedron: Asymmetry, 1995, 6, 3009; CrossRef (b) T. Itoh, H. Ohara, Y. Takagi, N. Kanda, and K. Uneyama, Tetrahedron Lett., 1993, 34, 4215. CrossRef
11. All new compounds were fully characterized by spectroscopic means [1H NMR (300 MHz in CDCl3), 13C NMR (68 MHz in CDCl3), and IR] and gave satisfactory HRMS (EI). Yields refer to homogeneous samples purified by chromatography on silica gel.
12. The ee value of 6 was determined by chiral HPLC analysis of the benzoyl ester derived from 6 (column, Daicel Chiralpak AD-H, 2-propanol: hexane = 1:400, flow rate = 0.5 mL/min; tR = 29.9 min for benzoate of 6, 25.8 min for the enantiomer).
13. Treatment of 6 with vinylmagnesium bromide and copper iodide produced a significant amount of the corresponding bromohydrin, formed by the ring opening with a bromide ion. The desired homoallylic alcohol was rather insufficiently obtained. This issue was avoided by the protection of the primary hydroxy group.
14. Synthesis of 12: To a cooled (-78 °C) stirred solution of 11 (1.25 g, 3.83 mmol) in THF (80 mL) was added dropwise LiHMDS (1.0 M solution in THF, 5.7 mL, 5.7 mmol). The mixture was stirred at -78 °C for 2 h, warmed to room temperature, and stirred for an additional 2.5 h. Then MOMCl (0.73 mL, 9.6 mmol) was added at 0 °C. The mixture was stirred for 2 h, diluted with brine (50 mL), and extracted with Et2O (30 mL x 3). The combined extracts were dried and concentrated in vacuo. The residue was purified by column chromatography on silica gel (EtOAc: hexane, 1:20) to provide 1.14 g (88%) of 12 (as a mixture of diastereomers regarding the asymmetric carbon in the THP group), and 114 mg (8%) of 11 was recovered. Compound 12 was obtained as white crystals: mp 61-63 °C TLC Rf 0.48 (EtOAc: hexane, 2:3); [α]D21 + 30.4 (c 0.985, CHCl3); IR 2945, 1755, 1665 cm-1; 1H NMR δ 1.42-1.80 (m, 6H), 2.39-2.71 (m, 2H), 3.13-3.18 (m, 2H), 3.46-3.56 (m, 1H), 3.50 (s, 3H x 1/2), 3.51 (s, 3H x 1/2), 3.52 (d, 1H x 1/2, J = 11.0 Hz), 3.67 (d, 1H x 1/2, J = 11.0 Hz), 3.74-3.85 (m, 1H), 3.89 (d, 1H x 1/2, J = 11.0 Hz), 3.97 (d, 1H x 1/2, J = 11.0 Hz), 4.59-4.69 (m, 1H), 5.01-5.30 (m, 6H), 5.61-5.77 (m, 1H), 5.85-5.99 (m, 1H); 13C NMR δ 18.3 x 1/2, 19.2 x 1/2, 25.2, 26.7, 30.1 x 1/2, 30.2 x 1/2, 39.6 x 1/2, 37.0 x 1/2, 57.0, 61.2 x 1/2, 62.3 x 1/2, 67.8 x 1/2, 68.4 x 1/2, 84.6 x 1/2, 84.7 x 1/2, 95.4, 97.6 x 1/2, 99.9 x 1/2, 102.0, 115.2, 119.8 x 1/2, 119.9 x 1/2, 129.9 x 1/2, 130.0 x 1/2, 135.8, 170.7, 173.3 x 1/2, 173.4 x 1/2; HRMS calcd for C18H26O6 (M+) m/z 338.1729, found 338.1731.
15. (a) A. K. Chatterjee, D. P. Sanders, and R. H. Grubbs, Org. Lett., 2002, 4, 1939; CrossRef (b) S. J. Spessard and B. M. Stoltz, Org. Lett., 2002, 4, 1943; CrossRef (c) D. R. Siegel and S. J. Danishefsky, J. Am. Chem. Soc., 2006, 128, 1048. CrossRef
16. M. Scholl, S. Ding, C. W. Lee, and R. H. Grubbs, Org. Lett., 1999, 1, 953. CrossRef
17. Synthesis of 13: To a stirred solution of 12 (1.18 g, 3.50 mmol) in 2-methyl-2-butene (18 mL) was added a solution of the second-generation Grubbs catalyst (122 mg, 0.144 mmol) in CH2Cl2 (6 mL). The mixture was stirred for 22 h and concentrated in vacuo. The residue was purified by column chromatography on silica gel (EtOAc: hexane, 1:20) to provide 1.36 g (99%) of 13 as a colorless oil (a mixture of diastereomers regarding the asymmetric carbon in the THP group): TLC Rf 0.51 (EtOAc: hexane, 1:2); [α]D21 + 1.8 (c 1.04, CHCl3); IR 2940, 1755, 1665 cm-1; 1H NMR δ 1.60 (s, 6H), 1.67 (s, 6H), 1.40-1.80 (m, 6H), 2.29-2.64 (m, 2H), 3.07 (d, 2H, J = 6.0 Hz), 3.46-3.55 (m, 1H), 3.49 (s, 3H x 1/2), 3.51 (s, 3H x 1/2), 3.53 (d, 1H x 1/2, J = 10.8 Hz), 3.67 (d, 1H x 1/2, J = 10.8 Hz), 3.74-3.91 (m, 1H), 3.87 (d, 1H x 1/2, J = 10.8 Hz), 3.94 (d, 1H x 1/2, J = 10.8 Hz), 4.59-4.68 (m, 1H), 4.95-5.04 (m, 2H), 5.14-5.24 (m, 2H); 13C NMR δ 17.7, 17.9, 18.3 x 1/2, 19.1 x 1/2, 21.9, 25.3, 25.5, 25.8, 30.2, 31.0 x 1/2, 31.1 x 1/2, 56.8 x 1/2, 56.9 x 1/2, 61.2 x 1/2, 62.0 x 1/2, 68.0 x 1/2, 68.4 x 1/2, 85.2 x 1/2, 85.4 x 1/2, 95.4, 97.5 x 1/2, 99.3 x 1/2, 104.6 x 1/2, 104.7 x 1/2, 115.5 x 1/2, 115.6 x 1/2, 122.3 x 1/2, 122.4 x 1/2, 132.1 x 1/2, 132.2 x 1/2, 136.1 x 1/2, 136.2 x 1/2, 169.5, 173.7 x 1/2, 173.8 x 1/2; HRMS calcd for C22H34O6 (M+) m/z 394.2355, found 394.2350.