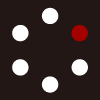
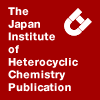
HETEROCYCLES
An International Journal for Reviews and Communications in Heterocyclic ChemistryWeb Edition ISSN: 1881-0942
Published online by The Japan Institute of Heterocyclic Chemistry
e-Journal
Full Text HTML
Received, 22nd April, 2008, Accepted, 2nd June, 2008, Published online, 5th June, 2008.
DOI: 10.3987/COM-08-S(F)13
■ Concise Assembly of the BCD Ring Part of Ginkgolide C via a Novel Cyclization Reaction
Ayaka Hibi, Kazutaka Takeda, and Masahiro Toyota*
Department of Chemistry, Graduate School of Science, University of Osaka Prefecture, Sakai, Osaka 593-8531, Japan
Abstract
Efforts to synthesize a 6-oxatricyclo[6.3.0.01.5]undecane ring system are described. A novel Pd(II)-promoted oxidative cyclization of the lactone ester constructs the tricyclic core of ginkgolide C.Ginkgo biloba is the only surviving member of a family of trees that appeared in the Jurassic period 170 million years ago and for this reason is called a “living fossil”. For approximately 5000 years, extracts of G. biloba have been used as herbal medicines to treat a variety of ailments, including coughs, asthma, and circulatory disorders. Recent clinical studies have attested to the potential benefits of ginkgolides in the delay of the onset of dementia.1
Ginkgolides vary only in the number and positions of their hydroxy groups (Figure 1). Ginkgolide A, B, C, and M were initially isolated from the root bark of G. biloba in 1932 by Furukawa.2 However, their structures were not elucidated until 1967.3,4 In 1987, ginkgolide J was isolated from the leaves of G. biloba.5
Ginkgolides are diterpenes with a cage skeleton consisting of six five-membered rings, i.e., a spiro[4.4]nonane carbocyclic ring, three lactones, and a tetrahydrofuran moiety. Furthermore, they contain an unprecedented tert-butyl group.
Herein, we describe the synthesis of the BCD tricyclic core of ginkgolide C. Scheme 1 shows our retrosynthetic analysis for 1. Namely, target molecule 1 would be synthesized by a cyclization reaction of lactone ester 2 followed by decarboxylation. Lactone 2 would be obtained from unsaturated ester 3 via halolactonization. Finally, unsaturated ester 3 would be provided from allyl alcohol 4 via a Claisen rearrangement.
We initially pursued the synthesis of cyclization precursor 2 as depicted in Scheme 2. The synthesis began with a Johnson-Claisen rearrangement6 of allyl alcohol 47 and trimethyl orthoacetate in the presence of p-anisic acid to afford methyl ester 3 in 70% yield. Hydrolysis of ester 3 with lithium hydroxide monohydrate, and subsequent iodolactonization furnished γ-butyrolactone 6 in 85% yield in two steps. Unsaturated lactone 7 was obtained in 95% yield by treating iodolactone 6 with DBU. Subsequent methoxycarbonylation of lactone 7 afforded desired lactone ester 2 in 98% yield.
The crucial cyclization was attempted under various conditions, some of which are listed in Table 1. Manganese(III)-promoted oxidative cyclization of 2 was adopted,8 and desired exo-olefin 89 was isolated in 64% yield (Entry 1). Next, palladium-promoted oxidative cyclizations of 2 were investigated. Treatment of 2 with a stoichiometric amount of Pd(OAc)2 in THF afforded 26% yield of exo-olefin 8 and 12% yield of endo-olefin 99 (Entry 2). Among the reaction conditions examined, employing DMSO as solvent was found to be the best condition as 8 was exclusively produced in 78% yield (Entry 3). To our best knowledge, there is not a report concerning palladium(II)-promoted oxidative cyclization of a lactone ester.
The relative stereochemistry was established using NOE experiments employing 109, the reduction product of 8, as described in Scheme 3. Finally, 8 was subjected to a Krapcho reaction10 to give rise to tricyclic lactone 19, the BCD tricyclic core of ginkgolide C, in 77% yield.
In conclusion, we developed a concise seven-step sequence to synthesize BCD tricyclic core 1 of ginkgolide C. A key feature of the synthesis is a new cyclization reaction. Further studies on the utility of this strategy for complex molecule synthesis as well as progress toward ginkgolide C will be reported in due course.
This paper is dedicated to Professor Emeritus Keiichiro Fukumoto in recognition of his many important contributions to the field of heterocyclic chemistry.
References
1. (a) K. Strømgaard and K. Nakanishi, Angew. Chem. Int. Ed., 2004, 43, 1640; CrossRef (b) S. H. Huang, R. K. Duke, M. Chebib, K. Sasaki, K. Wada, and G. A. R. Johnston, Eur. J. Pharmacol., 2004, 494, 131; CrossRef (c) L. Ivic, T. T. J. Sands, N. Fishkin, K. Nakanishi, A.R. Kriegstein, and K. Strømgaard, J. Biol. Chem., 2003, 278, 49279. CrossRef
2. S. Furukawa, Sci. Pap. Inst. Phys. Chem. Res. Tokyo, 1932, 19, 27.
3. (a) M. Maruyama, A. Terahara, Y. Itagaki, and K. Nakanishi, Tetrahedron Lett., 1967, 299; CrossRef (b) M. Maruyama, A. Terahara, Y. Itagaki, and K. Nakanishi, Tetrahedron Lett., 1967, 303; CrossRef (c) M. Maruyama, A. Terahara, Y. Nakadaira, M. C. Woods, and K. Nakanishi, Tetrahedron Lett., 1967, 309; CrossRef (d) M. Maruyama, A. Terahara, Y. Nakadaira, M. C. Woods, Y. Takagi, and K. Nakanishi, Tetrahedron Lett., 1967, 315; CrossRef (e) M. C. Woods, I. Miura, Y. Nakadaira, A. Terahara, M. Maruyama, and K. Nakanishi, Tetrahedron Lett., 1967, 321; CrossRef (f) K. Nakanishi, Pure Appl. Chem., 1967, 14, 89. CrossRef
4. (a) K. Okabe, K. Yamada, S. Yamamura, and S. Takada, J. Chem. Soc. C, 1967, 2201 ; CrossRef (b) N. Sakabe, S. Takada, and K. Okabe, J. Chem. Soc., Chem. Commun., 1967, 259. CrossRef
5. K. Weinges, M. Hepp, and H. Jaggy, Liebigs Ann. Chem., 1987, 521. CrossRef
6. W. S. Johnson, L. Werthemann, W. R. Bartlett, T. J. Brocksom, T. Li, D. J. Faulkner, and M. R. Petersen, J. Am. Chem. Soc., 1970, 92, 741. CrossRef
7. S. P. Moore, S. C. Coote, P. O’Brien, and J. Gilday, Org. Lett., 2006, 8, 5145. CrossRef
8. B. B. Snider, Chem. Rev., 1996, 96, 339. CrossRef
9. Data for selected new compounds: Compound 8: IR (KBr) 1781, 1732, 1264, 1056 cm-1; 1H NMR (400 MHz, CDCl3) δ 2.00-2.13 (m, 2H), 2.43 (ddd, J = 18.4, 2.1, 2.1 Hz, 1H), 2.58 (ddddd, J = 16.4, 10.8, 8.0, 2.8, 2.8 Hz, 1H), 2.66-2.74 (m, 2H), 3.76 (s, 3H), 4.95 (dd, J = 2.2, 2.2 Hz, 1H), 5.26 (dd, J = 2.2, 2.2 Hz, 1H), 5.40 (dd, J = 3.0, 1.8 Hz, 1H), 5.83 (ddd, J = 8.0, 2.4, 2.4 Hz, 1H), 6.12 (ddd, J = 5.6, 2.4, 2.4 Hz, 1H); 13C NMR (100 MHz, CDCl3) δ 31.9, 36.5, 41.6, 52.8, 61.3, 67.0, 91.1, 113.0, 127.2, 138.7, 148.4, 169.4, 172.7; LRMS (FAB) m/z 235 [(M+H)+], 154, 136; HRMS (FAB) calcd for C13H15O4 [(M+H)+] 235.0970, found 235.0981. Compound 9: IR (KBr) 1760, 1731, 1257, 1075 cm-1; 1H NMR (400 MHz, CDCl3) δ 1.83 (ddd, J = 3.8, 2.2, 2.2 Hz, 3H), 2.48 (ddd, J = 18.4, 2.4, 2.4 Hz, 1H), 2.58-2.69 (m, 2H), 2.77 (dddd, J = 18.2, 2.4, 2.4, 2.4 Hz, 1H), 3. 79 (s, 3H), 4.96 (dd, J = 2.0, 2.0 Hz, 1H), 5.72-5.74 (m, 1H), 5.84 (ddd, J = 5.6, 4.8, 2.4 Hz, 1H), 6.14 (ddd, J = 6.0, 2.4, 2.4 Hz, 1H); 13C NMR (100 MHz, CDCl3) δ 13.7, 41.3, 45.4, 52.8, 59.1, 72.8, 95.1, 127.1, 130.0, 137.2, 139.0, 169.0, 171.9; LRMS m/z 234 (M+), 131, 129, 91; HRMS calcd for C13H14O4 (M+) 234.0892, found 234.0872. Compound 10: IR (KBr) 1778, 1732, 1052 cm-1; 1H NMR (400 MHz, CDCl3) δ 1.20 (d, J = 6.8 Hz, 3H), 1.57-1.70 (m, 3H), 1.78-2.09 (m, 7H), 2.40-2.49 (m, 1H), 3.78 (s, 3H), 4.54 (dd, J = 5.2, 2.0 Hz, 1H); 13C NMR (100 MHz, CDCl3) δ 14.8, 24.4, 32.8, 33.9, 36.9, 38.6, 46.3, 51.9, 64.27, 67.7, 90.5, 168.9, 174.6; LRMS m/z 239 [(M+H)+], 178, 135, 134, 107; HRMS calcd for C13H19O4 [(M+H)+] 239.1283, found 239.1271. Compound 1: IR (KBr) 1764, 1157, 1001 cm-1; 1H NMR (400 MHz, CDCl3) δ 1.88-1.95 (m, 1H), 2.77 (ddd, J = 13.2, 6.0, 6.0 Hz, 2H), 2.45-2.50 (m, 2H), 2.56 (ddd, J = 1.6, 2.0, 17.8 Hz, 1H), 2.75 (ddd, J = 2.4, 4.8, 17.6 Hz, 1H), 3.20 (s, 1H), 5.11-5.13 (m, 2H), 5.27 (ddd, J = 2.0, 2.0, 2.0 Hz, 1H) 5.84 (dddd, J = 6.0, 4.0, 2.0, 2.0 Hz, 1H) 6.09 (dddd, J = 5.6, 5.6, 2.4, 0.8 Hz, 1H); 13C NMR (100 MHz, CDCl3) δ 33.5, 37.6, 45.2, 55.5, 57.6, 94.0, 110.3, 128.9, 137.4, 147.5, 177.2; LRMS m/z 176 (M+), 132, 117, 91; HRMS calcd for C11H12O2 (M+) 176.0837, found 176.0820.
10. A. P. Krapcho, Synthesis 1982, 805. CrossRef