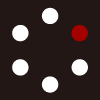
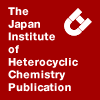
HETEROCYCLES
An International Journal for Reviews and Communications in Heterocyclic ChemistryWeb Edition ISSN: 1881-0942
Published online by The Japan Institute of Heterocyclic Chemistry
e-Journal
Full Text HTML
Received, 21st October, 2008, Accepted, 19th January, 2009, Published online, 22nd January, 2009.
DOI: 10.3987/REV-08-649
■ New Developments in the Synthesis of Saccharin Related Five- and Six-Membered Benzosultams
Zhaopeng Liu* and Yoshio Takeuchi
Department of Organic Chemistry, School of Pharmaceutical Sciences, Shandong University, No. 44, WenHua XiLu, Jinan 250012, China
Abstract
Recent developments in the novel synthesis of saccharin related five- and six-membered benzosultams are reviewed.1. INTRODUCTION
The sulfonamide unit has found many applications in medicinal chemistry. It has been incorporated in a still growing number of biologically active compounds, exhibiting antibacterial, anti-carbonic anhydrase, diuretic, hypoglycemic, antithyroid, antiviral and anticancer activity among others.1 In addition to being a carboxyl isostere, the introduction of the SO2–N moiety induces an increase of stability, for example, towards protease-catalyzed degradation in the case of sulfonamide-containing peptidomimetics.2 Recently, high interest has also been directed to the conformationally constrained cyclic counterparts, the sultams, which have served as key functional groups in the development of nonsteroidal antiinflammatory agents, agonists of 5-HT1A receptors, novel serine inhibitors, zinc enzyme carbonic anhydrase inhibitors, etc.3 Beyond their significance in the treatment of diseases, sultams have also been employed with considerable success as chiral auxiliaries or reagents in modern organic reactions.4,5 As a sister review to our previous one,4 this paper will focus on the recent developments in the synthesis of 1,2-benzisothiazoline 1,1-dioxide (saccharin) related five- and six-membered benzosultams, covering the recent literatures published from 2000. For the chemistry of isomeric 2,1-benzisothiazole 2,2-dioxides, please refer to the excellent review by K. Wojciechowski.6
2. FIVE-MEMBERED BENZOSULTAMS
2.1. Saccharin Derivatives
Due to the important biological activities of benzisothiazoline 1,1-dioxides, many efforts have been devoted to develop novel methods for the synthesis of analogues of saccharin derivatives. Aliyenne et al. reported an efficient way to prepare chiral N-substituted saccharin analogues from the readily available chiral 3-N-arylsulfonyloxazolin-2-ones.7 Compounds 1a–c and 3a–c were treated with two equivalents of LDA in anhydrous THF and HMPA at –78 °C, followed by quenching with saturated NH4Cl solution to afford the corresponding enantiomerically pure benzisothiazolinone 1,1-dioxides 2a–c and naphthoisothiazolinone 1,1-dioxides 4a–c with yields ranging between 62% and 71% (Scheme 1). The additive HMPA proved to be crucial to this reaction. Without HMPA or replacement it with TMEDA, the cyclization failed to proceed. Although ortho-lithiation could occur on C1 or C3 of the naphthalene ring, formation of the cyclization products 4a–c proved that the ortho-lithiation was regioselective and the deprotonation took place at the C1 position.
Benzenesulfonamide, both secondary and tertiary, is a powerful Directed Metalation Group (DMG) because of its ability to direct metalation to specific positions on the aryl ring. The sulfonamide Directed ortho-Metalation (DoM) methodology provides facile and exciting routes to synthesis of benzenesulfonamides and a wide variety of derived heterocycles.4,8 By using the powerful N-cumylsulfonamide directed ortho metalation-cross coupling strategies, Snieckus’s group developed a facile deprotection and expedient route to 7-substituted and 4,7-disubstituted saccharins.9
Treatment of N-cumylsulfonamide 5a with s-BuLi, 5b,c with n-BuLi in anhydrous THF in the presence of TMEDA at –78 °C, followed by reaction with iodine, gave the 2-iodo derivatives 6a–c in good yields. Suzuki cross coupling of the 2-iodo N-cumyl arylsulfonamides with aryl boronic acids led to formation of biaryl N-cumylsulfonamides 7a–d. n-BuLi/TMEDA ortho metalation of 7a–d, followed by N,N-diethylcarbamoyl chloride quench, gave the expected amide sulfonamides 8a–d. Mostly without isolation, these compounds were conveniently transformed directly by mild TFA decumylation followed by HOAc treatment into the N-unsubstituted 7-arylated saccharins 9a–d in modest to excellent yields (Scheme 2).
The n-BuLi/TMEDA ortho metalation and iodination of 8a,e gave the corresponding iodo derivatives 10a,b, respectively. Suzuki cross coupling with phenylboronic acid under the Na2CO3 conditions, afforded the tetrasubstituted aromatics 11a,b, which when subjected to the above decumylation and cyclization conditions, produced the 4,7-disubstituted saccharins 12a,b respectively, in good overall yields (Scheme 3).
2.2. Cyclic N-Sulfonylimines
3-Substituted benzo[d]isothiazole 1,1-dioxides represent an important class of compounds both as bioactive agents and synthetic intermediates for the construction of five- and six-membered benzosultams. The most direct method for the synthesis of such cyclic N-sulfonylimines involves the reaction of saccharin with organolithium or Grignard reagents.4 However, the unavailability and/or poor reactivity of the necessary hindered organometallic species limited the applications of this methodology. By adopting the DoM strategy and combining our TMSCl-NaI-MeCN reagent-mediated deprotection/cyclization10 methodology, we developed a new synthetic approach to benzo[d]isothiazole 1,1-dioxides having a secondary alkyl substituent at the 3-position (Scheme 4).11,12
A solution of N-t-butylbenzenesulfonamide in THF was treated with an excess of n-BuLi (2.5 eq) at 0 °C for 1 h, and then allowed to react with methyl 2-arylpropanoate, to give the ketone sulfonamides 13a–e in modest yields. The application of TMSCl-NaI-MeCN reagent-promoted deprotection-cyclization of sulfonamides 13a–e under reflux conditions, afforded the cyclic sulfonylimines 14a–e in good to excellent yields (62–94%).
2.3. Substituted 2,3-Dihydro-benzo[d]isothiazole 1,1-Dioxides
2.3.1. Pd-catalyzed Asymmetric Hydrogenation of Cyclic N-Sulfonylimines
Substituted benzosultams are important chiral auxiliaries that were first introduced in 1990 by Oppolzer13 and have since been successfully applied to a number of asymmetric transformations. Asymmetric hydrogenation of cyclic N-sulfonylimines is one of the most widely used synthetic approaches.
Yang et al. developed an efficient Pd-catalyzed asymmetric hydrogenation of N-tosylimines.14 Compound 15a was hydrogenated in CH2Cl2 solution at 40 °C and 75 atm, in the presence of 1.0 mol% Pd(OCOCF3)2/TangPhos complex, to give the methyl-substituted sultam with enantioselectivity of 94% ee and conversion of more than 99% (Table 1, entry 1). Wang et al. also successfully transformed the cyclic N-sulfonylimines 15a–c into the chiral sultams with Pd(OCOCF3)2/(S)-SegPhos complex with good enantioselectivities (entries 2–4).15
2.3.2. Intramolecular C−H Amination
Amidation of saturated C−H bonds catalyzed by metal complexes based on Fe, Mn, Ru, Cu, or Rh is the established methodologies for C−N bond formation.16 Fruit et al. investigated RhII-catalyzed intramolecular sulfonamidation with in situ generated phenyliodinanes derived from sufonamides.17 The amidation of 17a,b were carried out under in situ conditions with PhI(OAc)2 in the presence of MgO with 5 mol% Rh2(OAc)4 at 40 °C in CH2Cl2 for 2.5 h, to afford the cyclization products 18a,b in 65% and 45% yields, respectively (Scheme 5). Chiral RhII-catalysts were also tested for intramolecular asymmetric amidation of substrates 17a,b, although the enantioselectivity never exceeded 20%.17
Zhang’s group studied Co-catalyzed intramolecular C−H amination with azides as nitrene transfer sources.18 Using the commercially available 2,4,6-triisopropylbenzenenesulfonyl azide (19a) as a model substrate, they surveyed potential catalytic activity of various metalloporphyrins toward intramolecular C−H amination, and found that Co(II) was the most active metal ion with TPP as the supporting ligand. When the reaction was run in chlorobenzene with 2 mol% of Co(TPP) under N2 in the presence of 5 Å molecular sieves at 40 °C for 18 h, the desired benzosultam 20a was isolated in 96% yields. The Co(TPP) catalytic system was applied to a broad range of arylsulfonyl azides, having tertiary, secondary, and primary benzyl C−H bonds, containing functional groups such as bromo and nitro at different positions, thus leading to various benzosultam derivatives in excellent yields (Table 2). However, when an azide substrate containing different 2° C−H bonds such as benzylic and non-benzylic types was employed, both five- and six-membered ring formations were observed. For example, Co(TPP)-catalyzed intramolecular C−H amination of azide 19k with an n-butyl group led to the six-membered (21) and five-membered (20k) compounds (Scheme 6). The ratio of 20k to 21 was determined to be 72:28, 68:32, and 67:33 at 80 °C, 40 °C, and room temperature, respectively. The increase in the ratio of 20k to 21 at elevated temperature suggests the higher theromodynamic stability of the five-membered ring structure. Further efforts are necessary to identify suitable catalysts with high regioselctivity towards either five- or six-membered ring formation.
2.3.3. Catalyzed Intramolecular Aziridination
In recent years, metal-catalyzed intramolecular aziridination has become a new strategy for the preparation of cyclic sulfonamides. Dauban and co-workers investigated the copper- and bromine-catalyzed aziridinations.19,20 When olefinic primary sulfonamides 22a,b were treated with iodobenzene diacetate and potassium hydroxide in methanol, iminoiodiane intermediates 23a,b were formed. Iminoiodiane 23a underwent phenyltrimethylammonium tribromide (PTAB)-catalyzed intramolecular aziridination to give the desired benzosultam 24a in 70% yield. On the other hand, 23b was transformed into the cyclic sulfonamide 24b when treated with a catalytic amount of copper (I) triflate in acetonitrile in the presence of 4 Å molecular sieves (Scheme 7).19
Sulfonamides 22a,b reacted with tert-butylhypochlorite (t-BuOCl) and sodium hydroxide in water to form the corresponding N-chloramine salts, which were directly applied to PTAB-catalyzed intramolecular aziridination to form the aziridines 24a,b, respectively (Scheme 7).20
2.3.3. Catalyzed Intramolecular Aziridination
In recent years, metal-catalyzed intramolecular aziridination has become a new strategy for the preparation of cyclic sulfonamides. Dauban and co-workers investigated the copper- and bromine-catalyzed aziridinations.19,20 When olefinic primary sulfonamides 22a,b were treated with iodobenzene diacetate and potassium hydroxide in methanol, iminoiodiane intermediates 23a,b were formed. Iminoiodiane 23a underwent phenyltrimethylammonium tribromide (PTAB)-catalyzed intramolecular aziridination to give the desired benzosultam 24a in 70% yield. On the other hand, 23b was transformed into the cyclic sulfonamide 24b when treated with a catalytic amount of copper (I) triflate in acetonitrile in the presence of 4 Å molecular sieves (Scheme 7).19
Sulfonamides 22a,b reacted with tert-butylhypochlorite (t-BuOCl) and sodium hydroxide in water to form the corresponding N-chloramine salts, which were directly applied to PTAB-catalyzed intramolecular aziridination to form the aziridines 24a,b, respectively (Scheme 7).20
Rhodium (II, II) dimer has been studied for the intramolecular aziridination and amidation.21 Sulfonamides 22a–d underwent direct intramolecular aziridination catalyzed by Rh2(OAc)4 with PhI(OAc)2 and Al2O3 to afford the corresponding sultams in good to excellent yields (Scheme 8).
Chiral rhodium (II, II) dimer was applied to enantioselective intramolecular aziridinations.22 In the presence of 10 mol% [Rh2(4S-MEOX)4] and 1.5 equiv of PhIO, acyclic sulfonamides 22a–c and 22e,f were transformed into the optically enriched benzosultams in good yields with moderate enantioselectivity up to 76% ee (Scheme 9).
Following their work on dirhodium-catalyzed intramolecular aziridination of unsaturated sulfonamides,21,22 Che’s group further applied ruthenium porphyrins as catalysts for this transformation.23 As shown in Scheme 10, complex [Ru(F20-TPP)(CO)] (F20-TPP = 5,10,15,20-tetrakis(pentafluorophenyl)- porphyrinato dianion) proved to be an active catalyst for intramolecular C−N bond formation. When the reactions were conducted in dichloromethane at 40 °C for 3 h, with catalyst/substrate/PhI(OAc)2/Al2O3 molar ratio of 0.02:1:1.5:2.5, the corresponding cyclic aziridines 24a–e were obtained in good yields.
Ring opening of the aziridines can lead to 3-substituted five-membered benzosultams. For example, bicyclic sultam 24a reacted with allylmagnesium bromide to form benzo[d]isothiazole 25 (Scheme 11).19
2.3.4. TMSCl-NaI-MeCN Reagent-mediated Intramolecular C−N Bond Formation
Combining the sulfonamide directed ortho-metalation (DoM)8 strategy and TMSCl-NaI-MeCN reagent-mediated cyclization/deprotection methodology,10 we developed a facile synthesis of 3,3-disubstituted and spiro five-membered benzosultams.24
o-Lithiation of N-t-butylbenzenesulfonamide with t-BuLi followed by reaction with ketones gave carbinol sulfonamides 26a–g in modest to good yields. The sulfonamides 26a–g were subjected to TMSCl-NaI-MeCN reagent-mediated cyclization/deprotection under reflux conditions to afford benzosultams 27a–g in excellent yields (Table 3). This two-step synthesis makes 3,3-disubstituted and spiro 2,3-dihydro-benzo[d]isothiazole 1,1-dioxides readily available.
2.3.5. N-1-Alkynylated Benzosultams and Their Synthetic Utilities
Acetylenes are versatile starting materials for transition metal-mediated coupling reactions. Hirano et al. developed a practical preparation of N-(1-alkynyl)sulfonamides and studied their applications in titanium
alkoxide-mediated coupling reaction.25 In the alkynylation of benzosultam 28a (R1 = Me), the
Pd-catalysts were found to be totally ineffective, while Cu(I) was very efficient in catalyzing this transformation. The best result was obtained when 28a was treated with one equivalent of 1-bromo-1-octyne (29, R2 = n-hexyl) in the presence of 5 mol% CuI and K3PO4, with 2.5 mol% N,N’-dimethylethylenediamine in toluene at 110 °C for overnight, to afford 30a in 79% yield (Table 4, entry 1). Under the optimum conditions, a variety of benzosultams were alkynylated in good to excellent yields (Table 4). When using a chiral substrate, the enantiomeric purity of optical active sultam (S)-28c (R1 = t-Bu) was completely retained in the product 30e (96% ee, entry 5).
The amino-substituted acetylenes obtained above were demonstrated to be useful intermediates for
diastereoselective synthesis. Sultam 30b was first treated with titanium (II) alkoxide, Ti(O-i-Pr)4/2
i-PrMgBr, to generate the acetylene-titanium complex 31, which was then allowed to couple with benzaldehyde to give 32. After hydrolysis, the adduct 33a was obtained in good yield, showing virtually complete regio- and olefinic stereoselectivities with high 1,5-diastereoselectivity (ds = 96:4). The excellent level of the remote asymmetric induction (1,5-diastereoselectivity) is noteworthy. Other types of aldehydes and sultams underwent the same transformation and the results are summarized in Table 5. Figure 1 shows a proposed stereochemical course of the above reaction. The intermediate shown below, which fulfills (i) the least hindered conformation of the sultam moiety to the substituent (Me3Si or C6H13) of the titanated acetylene, (ii) the approach of the aldehyde from the less hindered side (opposite to the Me or t-Bu group on the sultam), and (iii) the less hindered orientation of the side chain (R3) of the
aldehyde, may account for the observed stereochemistry of the adducts 33a–m.
Copper-catalyzed N-alkynylation of sultam 28a with bromodiynes 34a,b, successfully afforded 35a,b, which were then cyclized with titanium reagent to give, upon hydrolysis or deuteriolysis, the desired amino-substituted exo-cyclic dienes 36a,b in excellent yields (Scheme 12). The intramolecular coupling of two acetylenic moieties offers an attractive method to prepare cyclic compounds.
The copper-catalyzed N-alkynylation of sultam 28a,c with bromoenynes also proceeded as usual, unaffected by the neighboring olefinic moiety in the same molecule, to give N-(1-alkynyl)sulfonamides 37a,b having an olefinic appendage. Although the observed diastereoselectivities of the subsequent titanium alkoxide-mediated coupling reaction fell in an unsatisfactory range (ds = 1:1), the desired cyclic products 38a,b were obtained in good yields (Scheme 13).
3. SIX-MEMBERED BENZOSULTAMS
3.1. Catalyzed Intramolecular Aziridination
As shown in Scheme 14, olefinic primary sulfonamide 39a was allowed to react with iodobenzene diacetate and potassium hydroxide in methanol to form an intermediate iminoiodiane, which underwent copper (I) triflate-catalyzed intramolecular aziridination to offer the desired benzosultam 40a in 60% yield. Ring opening of the aziridine 40a with benzylamine led to the 3-substituted six-membered benzosultam 41 in 62% yield.19
Sulfonamide 39a was treated with tert-butylhypochlorite (t-BuOCl) and sodium hydroxide in water to give the N-chloramine salt intermediate, which was subjected to an intramolecular reaction in the presence of a catalytic amount of phenyltrimethylammonium tribromide (PTAB) to produce the aziridine 40a in 46% yield.20
Sulfonamide 39a–c underwent direct intramolecular aziridination catalyzed by Rh2(OAc)2 with PhI(OAc)2 and Al2O3 to afford sultams 40a–c in good yields (Scheme 15).21
In the presence of catalyst [Ru(F20-TPP)(CO)], the reaction between 39a and PhI(OAc)2 led to the intramolecular aziridination product 40a (41%) and five-membered benzosultam 42 (25%), as shown in Scheme 16. It is reasonable that unsaturated sulfonamide 39a that has a benzylic C−H bond can also undergo intramolecular amidation to form the five-membered product 42.21
3.2. TMSCl-NaI-MeCN Reagent-mediated Deprotection/Cyclization
3,3-Disubstituted and spiro six-membered benzosultams can be efficiently prepared via the TMSCl-NaI-MeCN reagent-mediated deprotection/cyclization method which we developed.10 Some 3-monosubstituted six-membered benzosultams are available from N-acyl-o-toluenesulfonamides in two steps via ortho-methyl lithiation/cyclization processes followed by Pd/C-catalyzed hydrogenation,26 but this procedure is not suitable for the preparation of 3-arylated analogues. Recently, we developed a novel two-step method for the facile synthesis of 3-monosubstituted six-membered benzosultams, applying the sulfonamide directed ortho-methyl metalation (DoM) effect and TMSCl-NaI-MeCN reagent-mediated cyclization/deprotection strategy (Scheme 17).27,28
N-Boc-o-toluenesulfonamide underwent ortho-methyl metalation, as well as N-metalation, with two equivalents of n-BuLi in anhydrous THF under nitrogen atmosphere at –78 °C for 30 min to form dilithiosulfonamide, which reacted with aldehydes to form carbinols 43a–o in good yields. When carbinols 43a–l were treated with two equiv of TMSCl and NaI in acetonitrile under reflux conditions for 1.5 h, the cyclization proceeded smoothly to produce the benzosultams 44a–l. As can be seen from Table 6, this procedure is especially effective for preparation of 3-monoarylated benzosultams with various important functional groups that include both electron donating and withdrawing ones, such as chloro, fluoro, dimethylamino, methoxy, and cyano groups (entries a–e, j–l). For the compounds having less bulky aliphatic substituents at the 3-position, the yields are moderate (entries f and g), but for those having bulky aliphatic substituents such as cyclohexyl and tert-butyl groups (entries h and i), the yields are less than satisfactory even for an prolonged reaction time (10 h).
It is also of note, when carbinols 43j–o was treated with 4 equiv of TMSCl and NaI for each methoxy group and refluxed in acetonitrile for a prolonged time, cyclization and demethylation can take place simultaneously and the phenolic benzosultams 45j–o were readily obtained (Table 6, entries j–o).28 Thus, the TMSCl-NaI-MeCN reagent-mediated cyclization and demethylation strategy provides the most direct way to these phenolic and polyphenolic compounds that have both important phenolic and sulfonamide pharmacophores in the structure.
As shown in Scheme 18, the TMSCl/NaI/MeCN reagent-mediated novel cyclization is assumed to proceed through a sequence of consecutive processes, which involve removal of the Boc protective group, conversion of the hydroxy group to iodide, and an intramolecular nucleophilic substitution for the cyclization. When substrate 43i was treated with two equiv of TMSCl and NaI in acetonitrile at room temperature for 2 h, the N-Boc protective group was cleaved thoroughly to form the carbinol sulfonamide 46, which was converted into the iodide 47 rather slowly at room temperature. Only 25% iodide 47 was formed after 16 h. When heated, substrate 43i was converted to the iodide 47 much more quickly (in ca. 30 min). After heating for 10 h, the cyclization product 44i (25%) and the elimination product 48 (42%) were isolated, along with the unreacted iodide 47 (20%).
It is apparent that the intramolecular nucleophilc substitution (cyclization) and elimination reaction are competitive, and the sterically hindered iodide 47 with benzylic hydrogens favors the elimination, so the olefin 48 thus formed is predominant. The bulky alkyl iodides (table 6, entries h and i) are less reactive than the less bulky ones (entries f and g) and the benzyl iodides in the bimolecular nucleophilic substitution. Thus, the 3-monoalkylated benzosultams with bulky substituents such as cyclohexyl and t-Bu groups were obtained in much lower yields.
3.3. Copper (II) Carboxylate-promoted Intramolecular Carboamination of Olefins
Sherman et al. first reported copper (II) acetate reagent-promoted oxidative cyclization of arylsulfonyl-o-allylanilines.29 When sulfonamides 49a–d in DMF (0.08 M) were treated with Cu(OAc) 2 (3 equiv) and Cs2CO3 (1 equiv), and heated at 120 °C for 24 h in a pressure tube, the tetracycles 50a–d were obtained in moderate yields (Scheme 19). Substrates with electron donating groups proved most reactive, i.e., 4-methyl, methoxy, and bromoaryl sulfonamides reacted efficiently albeit the bromine was removed under the reaction conditions (50c, R = H). On the other hand 4-nitro and 4-trifluoromethyl arylsulfonamides displayed significantly lower reactivity. As for solvent, DMF proved to be better than MeCN and addition of DMSO (4 equiv) increased the yields, sometimes with significant effect.29
For meta-substituted arylsulfonamides, copper (II) acetate reagent-promoted oxidative cyclization generally led to a mixture of regioisomeric products (Scheme 19, substrates 49f–h), with a preference (ca. 2:1) for the forming of the ortho-addition (50f–i) over the para-addition (51f–i) products. The reaction of meta-substituted nitrosulfonamide 49i afforded the ortho-adduct as a sole cyclization product, albeit in low yield. This surprising ortho preference (sterically more hindered site) indicated that C−C bond formation might occur via addition of a carbon radical to the aromatic ring. Further studies support the following proposed mechanism shown in Scheme 20.30 Copper (II) carboxylate-promoted intramolecular carboamination of olefins involves nitrogen-copper (II) bond formation followed by aminocupration (intramolecular migratory insertion), generating an unstable organocopper (II) species that homolyzes to the carbon radical and Cu (I), and subsequent addition of the primary carbon radical to an aromatic ring.
In the optimization of the best reaction conditions, the more organic soluble copper (II) neodecanoate [Cu (ND)2] was found to be suitable to promote the intramolecular carboamination. A variety of unactivated olefins, including arylsufonamides derived from aliphilic amines and substrates with different olefin substitution patterns, were applied for Cu(ND)2-mediated oxidative cyclization. The results are summarized in Table 7.30
All of the substrates examined in this reaction give exclusively the exo cyclization adducts except for the 1,1-disubstitued olefin 49j, which gave a ca. 1:1 mixture of the exo carboamination adduct 50j and the endo oxidative amination adduct 52j (Table 7, entry 2). Sufonamides 49k–m, derived from aliphilic amines (entries 3–5), underwent the cyclization efficiently. The formation of hydroamination minor products was also observed with some substrates and the carboamination to hydroamination ratio is presumably a function of the relative rates of radical addition to the aromatic ring vs hydrogen atom abstraction from the reaction medium (Scheme 20). The carboamination adducts derived from styrenyl
substrates 49n,p were obtained in modest yields upon heating at 200 °C (entries 6 and 8). Microwave heating was proved to be effective and reduced the reaction time (entries 4 and 7). Further to expand the scope of the carboamination reactions, several α-substituted γ-alkenyl sulfonamides 49q–v were examined for the preparation of 2,5-disubstituted pyrrolidines (Table 8).
In copper (II)-promoted oxidative cyclization of enantiomerically enriched sulfonamides 49q–v, cis-2,5-disubstituted pyrrolidines 50 were formed with very high diastereoselectivity (>20:1) and in 31–51% yields, using either oil bath heating (sealed tube, 170–210 °C, 72 h) or microwave irradiation (210 °C, 3 h). The net hydroamination adducts 52 were formed in these reactions as well (15–28%) with high 2,5-cis selectivity in all cases examined. The products emerged as a ca. 2:1 mixture of carboamination and hydroamination adducts 50 and 52, respectively.
3.4. Pd/C-mediated C−C Coupling Followed by Iodocyclization
A two-step synthesis of iodo benzothiazines, that involved (i) Pd/C-mediated coupling of o-halo-benzenesulfonamides with terminal alkynes and (ii) iodocyclization, was developed.31 In optimizing reaction conditions for the Sonogashira coupling of o-halo-benzenesulfonamides with terminal alkynes, it was observed that the use of 10% Pd/C-PPh3-CuI as a catalyst system in the presence of Et3N in acetonitrile produced the best result. Under the optimum conditions, 2-alkynyl-N-benzenesulfonamides 54 were prepared (Table 9).
Generally, o-halo-benzenesulfonamides exhibited high reactivity towards Pd/C-mediated Sonogashira coupling when all the reactions were carried out using a 1:4:2 ratio of Pd/C-PPh3-CuI at 80 °C for 8–24 h. Both bromo (53a) and iodo (53b–d) derivatives reacted well with terminal alkynes bearing various substituents such as alkyl, hydroalkyl, chloroalkyl, cyanoalkyl, aryl, and heterocyclic groups. Target compounds were obtained in good to excellent yields (85–97%) in all the cases, irrespective of the nature of the substituents present in the halides as well as alkynes. This allowed the preparation of a variety of functionalized o-(1-alkynyl)benzenesulfonamides 54a–o.
o-(1-Alkynyl)benzenesulfonamides 54a–o underwent smooth iodocyclization under the conditions with 2.5 equiv of iodine and 3 equiv of K2CO3 in acetonitrile at room temperature to afford 4-iodo-2H-benzo[e][1,2]thiazine-1,1-dioxides 55a–o in good yields (62–87%, Table 10). Substituents such as methyl, ethyl, and methoxy groups on the benzene ring, and alkyl, chloroalkyl, cyanoalkyl, heterocyclyl alkyl, and aryl groups at the acetylenic end were well tolerated. In general, simple alkyl and aryl substituents on the triple bond of compounds 54 cyclized more rapidly (e.g. 6–12 h) than others (12–24 h), where various groups such as hydroxyl, chloro or cyano were present at the terminal position of the alkyl side chain (R2). The present iodocyclization of 54 showed very high selectivity for six-membered ring formed as a result of ‘6-endo-dig’ ring closure. No isomeric five-membered ring products were detected under the conditions employed, nor the product involving the simple addition of iodine to the triple bond of 54.
A plausible mechanism for the formation of 4-iodo-2H-benzo[e][1,2]thiazine 1,1-dioxides 55 via iodocyclization is shown in Scheme 21.
The reaction proceeds via (i) activation of the triple bond of 54 by coordination to I+ followed by (ii) intramolecular nucleophilic attack by nitrogen of the sulfonamide group in an ‘endo-dig’ fashion. Thus, the iodocyclization seems to be facilitated by several factors. (1) The alkyl group enhances the nucleophilicity of the nitrogen of the sulfonamide moiety. (2) The electron withdrawing effect of the sulfonamide moiety makes the vinylic cation susceptible for nucleophilic attack leading to the formation
of six-membered ring. (3) The presence of a base facilitates the efficient N−H bond cleavage as cyclization proceeds.
The presence of the iodo group at the C-4 position of the benzothiazine ring allows further structural elaboration through conversion of the iodide functionality into other substituents. Compound 55k was exposed to Sonogashira, Heck, and Suzuki coupling conditions in the presence of a terminal alkyne, olefin or aryl boronic acid individually, the corresponding products 56a, 56b, and 56c formed via C−C bond forming reactions were isolated in 82–92% yields (Scheme 22).
3.5. Abramovitch’s Ring Expansion of 3-Bromoalkyl-1,2-benzisothiazole 1,1-Dioxides
Abramovitch’s ring-expansion procedure is an efficient way for the preparation of 3,3-disubstituted 2H-benzo[e][1,2]thiazine 1,1,4-triones.32 Cyclic N-sulfonylimines 14a,e were brominated in benzene to give bromides 57a,e quantitatively, which underwent Abramovitch’s ring expansion mediated by 20% KOH (aq.) to form benzosultams 58a,e in excellent yields. Optical resolution of the racemic benzosultams 58a,e was performed by derivatization with (–)-menthoxyacetyl choride followed by the separation of the diastereomers (+)-59aa (more polar, 40%) and (–)-59ab (less polar, 46%), and (+)-59ea (more polar, 39%) and (–)-59eb (less polar, 35%) respectively, using column chromatography on silica gel. Removal of the chiral auxiliaries of 59 was achieved with LiOH in aqueous THF to furnish the corresponding enantiomerically pure (+)- and (–)-58a and (+)- and (–)-58e, respectively (Scheme 23).12,33 These optically pure benzosultams are useful as chiral auxiliaries for asymmetric synthesis and templates for novel chiral N–F agents.
4. CONCLUSION
In conclusion, much progress has been made in the construction of saccharin related five- and six-membered benzosultams in recent years, especially via the sulfonamide directed ortho-metalation (DoM)/coupling strategy and cyclization mediated by TMSCl-NaI-MeCN or other reagents, metal-catalyzed intramolecular C−H amination, aziridination, olefin carboaminations, and Pd/C-mediated coupling/iodocyclizations. Further researches will be directed to the development of the asymmetric versions of these novel reactions for the preparation of optically active benzosultams. Considering the diverse biological profiles of sulfonamide containing compounds, functionalized benzosultams are expected to find many application in life science related areas.
ACKNOWLEDGEMENTS
This work was supported by the Natural Science Foundation of Shandong Province (Y2007C125) and the Natural Science Foundation of China (30873134). Dr. Z. Liu likes to dedicate this paper to his MS supervisor, Professor Lijun Xu, who passed away on Jun. 25, 2008.
References
1. C. T. Supuran, A. Innocenti, A. Mastrolorenzo, and A. Scozzafava, Mini-Reviews in Med. Chem., 2004, 4, 189.
2. D. B. A. de Bont, K. M. Sliedret-Bol, L. J. F. Hofmeyer, and R. M. J. Liskamp, Bioorg. Med. Chem., 1999, 7, 1043. CrossRef
3. P. Dauban and R. H. Dodd, Org. Lett., 2000, 2, 2327, and references cited therein. CrossRef
4. Z. Liu and Y. Takeuchi, Heterocycles, 2002, 56, 693. CrossRef
5. A. W. M. Lee, W. H. Chan, S.-J. Zhang, and H.-K. Zhang, Curr. Org. Chem., 2007, 11, 213. CrossRef
6. K. Wojciechowski, Heterocycles, 2002, 57, 1717. CrossRef
7. A. O. Aliyenne, J. E. Khiari, J. Kraïem, Y. Kacem, and B. B. Hassine, Tetrahedron Lett., 2006, 47, 6405. CrossRef
8. See a review: O. B. Familoni, Synlett, 2002, 1181. CrossRef
9. J. Blanchet, T. Macklin, P. Ang, C. Metallinos, and V. Snieckus, J. Org. Chem., 2007, 72, 3199. CrossRef
10. Z. Liu, N. Shibata, and Y. Takeuchi, J. Org. Chem., 2000, 65, 7583. CrossRef
11. Z. Liu, T. Toyoshi, and Y. Takeuchi, Synth. Commun., 2004, 34, 471. CrossRef
12. Z.-P. Liu, Heterocycles, 2007, 71, 1843. CrossRef
13. W. Oppolzer, M. Wills, C. Starkemann, and G. Bernardinelli, Tetrahedron Lett., 1990, 31, 4117; CrossRef W. Oppolzer, M. Wills, M. J. Kelly, M. Signer, and J. Blagg, Tetrahedron Lett., 1990, 31, 5015; CrossRef W. Oppolzer, I. Rodriguez, C. Starkemann, and E. Walther, Tetrahedron Lett., 1990, 31, 5019. CrossRef
14. Q. Yang, G. Shang, W. Gao, J. Deng, and X. Zhang, Angew. Chem. Int. Ed., 2006, 45, 3832. CrossRef
15. Y.-Q. Wang, S.-M. Lu, and Y.-G. Zhou, J. Org. Chem., 2007, 72, 3729. CrossRef
16. P. Müller and C. Fruit, Chem. Rev., 2003, 103, 2905. CrossRef
17. C. Fruit and P. Müller, Helv. Chim. Acta, 2004, 87, 1607. CrossRef
18. J. V. Ruppel, R. M. Kamble, and X. P. Zhang, Org. Lett., 2007, 9, 4889. CrossRef
19. P. Dauban and R. H. Dodd, Org. Lett., 2000, 2, 2327. CrossRef
20. P. Dauban and R. H. Dodd, Tetrahedron Lett., 2001, 42, 1037. CrossRef
21. J.-L. Liang, S.-X. Yuan, P. W. H. Chan, and C.-M. Che, Org. Lett., 2002, 4, 4507. CrossRef
22. J.-L. Liang, S.-X. Yuan, P. W. H. Chan, and C.-M. Che, Tetrahedron Lett., 2003, 44, 5917. CrossRef
23. J.-L. Liang, S.-X. Yuan, J.-S. Huang, and C.-M. Che, J. Org. Chem., 2004, 69, 3610. CrossRef
24. Z. Liu, N. Shibata, and Y. Takeuchi, J. Chem. Soc., Perkin Trans. 1, 2002, 302. CrossRef
25. S. Hirano, R. Tanaka, H. Urabe, and F. Sato, Org. Lett., 2004, 6, 727; CrossRef S. Hirano, Y. Fukudome, R. Tanaka, H. Urabe, and F. Sato, Tetrahedron, 2006, 62, 3896. CrossRef
26. Y. Takeuchi, Z. Liu, A. Satoh, T. Shiragami, and N. Shibata, Chem. Pharm. Bull., 1999, 47, 1730.
27. F. Fang, R. Wang, Z.-P. Liu, and A.-G. Ji, Heterocycles, 2007, 71, 2377. CrossRef
28. R. Wang, F. Fang, L.-Q. Tang, and Z.-P. Liu, Lett. in Org. Chem., 2008, 5, 461. CrossRef
29. E. S. Sherman, S. R. Chemler, T. B. Tan, and O. Gerlits, Org. Lett., 2004, 6, 1573. CrossRef
30. E. S. Sherman, P. H. Fuller, D. Kasi, and S. R. Chemler, J. Org. Chem., 2007, 72, 3896. CrossRef
31. D. K. Barange, V. R. Batchu, D. Gorja, V. R. Pattabiraman, L. K. Tatini, J. M. Babu, and M. Pal, Tetrahedron, 2007, 63, 1775. CrossRef
32. R. A. Abramovitch, K. M. More, I. Shinkai, and P. C. Srinivasan, J. Chem. Soc., Chem. Commun., 1976, 771. CrossRef
33. H. M. Sun, Z. P. Liu, and L. Q. Tang, Chin. Chem. Lett., 2008, 19, 907. CrossRef