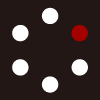
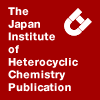
HETEROCYCLES
An International Journal for Reviews and Communications in Heterocyclic ChemistryWeb Edition ISSN: 1881-0942
Published online by The Japan Institute of Heterocyclic Chemistry
e-Journal
Full Text HTML
Received, 29th April, 2008, Accepted, 27th May, 2008, Published online, 2nd June, 2008.
DOI: 10.3987/COM-08-S(F)15
■ Preparation of a Building Block for a Pyrazole Expanded Porphyrin and Isolation of a Tripyrrolyl Ketone Decomposition Product
Alfred Hassner* and Eran Fogler
Department of Chemistry, Bar-Ilan University, Ramat-Gan 52900, Israel
Abstract
Dipyrrolylpyrazole 3, obtained from 1,3-di-(1H-pyrrol-2-yl)propane-1,3-dione (2), was prepared as a building block for pyrazole-based binuclear expanded porphyrins. An unusual transformation of 2, in solution or on chromatography, afforded 1,3,3-tri-(1H-pyrrol-2-yl)prop-2-en-1-one (4) in good yield. HRMS indicated the presence of an unstable pyrazole-based expanded porphyrin 1 and its trinuclear Mg derivative 5 on condensation of 3 with benzaldehyde.Considerable effort has been devoted in recent years to the preparation of binuclear complexes of expanded porphyrins.1-12 Given the relatively small number of binuclear complexes of expanded porphyrins prepared to date and the smaller number of well-characterized metal complexes that have been reported, it is clear that many aspects of binuclear complexes of expanded porphyrin chemistry remain to be explored.
We were interested in the synthesis of pyrazole-based binuclear expanded porphyrins of type 1 or 5 in order to investigate the influence of such a sub-unit on their chemistry. For instance, there was the possibility that introduction of a metal may cause the pyrazole sub-unit to spin to a different rotamer or to obtain additional chelation by the two pyrazole nitrogens, similar to one (Figure 1) reported by Sessler et al.5 Furthermore, binuclear metal complexes of expanded porphyrins are of potential interest as catalysts for reaction with atmospheric oxygen or CO2 and as therapeutics for photodynamic therapy or for anion sensing and transport.
The plan for the synthesis of expanded porphyrin 1 was to obtain 3,5-dipyrrolylpyrazole 3 via dipyrrolyl diketone 2, and condense it with benzaldehyde (to 1) or with 2,5-bishydroxybenzyl furan in the presence of various acids followed by oxidation, as commonly used in the synthesis of porphyrin compounds (Scheme 1).1,2,9,10,13,14
Pyrrolyl diketone 2 was obtained in 66% yield (reported 33%)15 by reaction of pyrrole with malonyl dichloride in dichloromethane (DCM) at -2 oC, following the procedure reported by Maeda et al.,15 but in
an open system protected with a CaCl2 tube instead of under argon and purifying 2 using washes only without initial attempts to purify it by chromatography. While diketone 2 was obtained from the above reaction in at least 95% purity (by NMR), attempts to further purify it by chromatography over silica or alumina led unexpectedly to 1,3,3-tripyrrolylpropenone (4) in good yield (71%). Though 2 was stable as a solid in a freezer for months, its conversion to 4 occurred in high yield (81%) even on standing for 5 months in a solution of DCM-hexane in the cold.
The formation of tripyrrolyl derivative 4 requires either reaction of the enol of 2 with a pyrrole unit (formed in a decomposition of 2 as in Scheme 1) or by self-condensation of 2 (see Scheme 2). Hence it was important to establish the absence of free pyrrole in the diketone sample before chromatography. In the isolation of diketone 2 from malonyl dichloride and pyrrole, we confirmed the complete consumption of pyrrole by tlc and the absence of characteristic pyrrole peaks in the 1H-NMR and MS of the diketone product 2, before chromatography transformed it into the tripyrrolyl derivative 4.
The structure of 4 was unequivocally determined by 1H- and 13C-NMR, HRMS, IR and UV-VIS spectra. Furthermore, the spectral data of 4 resemble those of its mono phenyl or thienyl analog prepared by double addition of pyrrole to a corresponding bromo acetylenic ketone by Trofimov et al.16,17 From 1H-NMR, 13C-NMR and H-H interactions (COSY in Figure 3) it was clear that there are three different pyrroles (“A”, “B” and “C”) in the molecule, while C-H interactions in the NMR (HMQC & HMBC) provided a final association of the carbons and hydrogens in pyrroles “A”, “B” and “C”. The C-H coupling of the carbonyl carbon with the (3-CH) hydrogen in pyrrole “C” established a 2-keto-pyrrole structure for this sub-unit. In the 1H-NMR and 2D-NMR of 4 in DMSO there is a peak at 13.5 ppm for one of the (NH) hydrogen (see Figure 3), and the others are found at 11.8 and 11.5 ppm, while in a mixture of acetone-d6 and MeOD there was an exchange of only the NH at 11.5 ppm and not of the “H-bonded hydrogens”, indicating strong intramolecular H-bonding to the C=O. In solution IR (in DCM) there were two broad peaks near 3390 and 3290 cm-1, suggesting a strong, intramolecular H-bond of the hydrogens to the ketone. Additionally, the H-bonded structure in Figure 3, as well as extensive conjugation of the ketone with the two pyrroles contributes to the fact that the C=O stretching frequency of 4 appears as a strong peak at 1590 cm-1, instead of near 1650 cm-1 expected for an unsaturated C=O. Furthermore, this IR is similar to that of an analogous compound possessing a thiophene instead of a pyrrole adjacent to the C=O.16,17 HRMS was also consistent with structure 4.
Reaction of diketone 2 with hydrazine hydrate in isopropanol under reflux produced dipyrrolyl pyrazole 3 in 75% yield after recrystallization. NMR and HRMS of 3 were consistent with its structure.18
Attempts to prepare expanded porphyrin 1 by condensation of dipyrrolylpyrazole 3 with benzaldehyde in acetic acid at 70 oC under Schlenck conditions led upon exposure to air to a purple unstable crude product that showed the required molecular ion peaks at m/z 566 (1, 22 πe) and m/z 568 (dihydro 1), but the color of which faded on standing in acetone-d6 for 20 min. The electronic absorption spectrum (280 nm and 560 nm) of the crude product is also consistent with formation of an expanded porphyrin. Attempts to oxidize dihydro 1 to 1 by DDQ or exposure to air led to decomposition, as did attempts to stabilize it by the presence of metal ions (Zn(II), Pd(II), Ni(II)). Condensation of 3 with benzaldehyde in the presence of Mg(II) acetate did furnish a crude blue solid which showed a matching HRMS in which the typical expected isotopic effect of three magnesium atoms inside the molecule was detected (Figure 4), as shown by peaks at 637.143, 638.147, 639.141, and 640.140 and consistent with a trimagnesium derivative 5. However, even this derivative was not stable and decomposed in the presence of water, light, on chromatography or attempted crystallization. The lack of stability of expanded porphyrin 1 and its metal derivative may be due to lack of planarity as well as to its anti-aromatic character (22 πe), but improved stability might be achievable by using substituted substrates.
In summary, 3,5-di-(1H-pyrrol-2-yl)-1H-pyrazole (3), obtained from 1,3-di-(1H-pyrrol-2-yl)propane-1,3-dione (2) was prepared as a potential building block for pyrazole-based expanded porphyrin 1. HRMS of unstable products resulting from condensation of 3 with benzaldehyde indicated the presence of 1 and its unstable trinuclear (trimagnesium) expanded porphyrin 5. An unusual apparent self-condensation of diketone 2 under mild conditions led to 1,3,3-tri-(1H-pyrrol-2-yl)prop-2-en-1-one (4) in good yield .
EXPERIMENTAL SECTION
1H and 13C NMR spectra of 200-MHz, 300-MHz and 600-MHz were obtained on Bruker AC-200, AM-300 and DMX-600 spectrometers, respectively. Chemical shifts are expressed as δ values in ppm downfield from Me4Si used as an internal standard. HRMS were obtained on a VG AutoSpec E spectrometer. Melting points were obtained on a Fisher-Johns melting point apparatus. Reaction progress was monitored by TLC on silica gel (Merck, Art. 5554), or neutral alumina (Merck, 60 F254). Flash chromatography was carried out on silica gel (Merck, Type 9385), basic alumina (Merck, Type 58A), acidic alumina (Merck, Type 504C) or alumina (Merck, Type 506-C-1). Unless otherwise stated, all commercially available compounds were used without further purification.
General chemical methods: Unless otherwise stated, all reactions using air- or water-sensitive reagents were conducted under a dry inert gas atmosphere (argon) with dry solvents, and all glassware was dried at
120 oC overnight and flame dried before use. THF was distilled over Na/benzophenone ketyl under dry inert gas immediately prior to use. Dichloromethane was distilled over P2O5 under dry inert gas and stored in the presence of activated 4 Å molecular sieves. Benzaldehyde was distilled immediately prior to use. Pyrrole was purified on an Al2O3 column immediately prior to use. Argon and N2 were dried over a SiO2/CoCl2 column immediately prior to use. For NMR studies CDCl3 was purified by passing through a basic alumina micro column (made from a pipette) immediately prior to use in order to eliminate HCl (or DCl) from the CDCl3. All NMR tubes were wrapped in aluminum foil, to prevent exposure to light. All samples for MS were saved at -20 oC in the dark; in the case of highly sensitive material, MS was performed immediately. All reactions were performed in the dark. All crystallizations were carried out at 4 oC or at -20 oC in the dark. Consumption of starting material was confirmed using TLC analysis.
1,3-Di-(1H-pyrrol-2-yl)propane-1,3-dione (2): Based on a reported procedure,15 a solution of pyrrole (2.01 g, 30 mmol) in dry CH2Cl2 (90 mL) protected with a drying tube was treated with malonyl chloride (2.115 g, 15 mmol) at -2 oC (in a NaCl/water/dry ice bath) and stirred for 3 h at the same temperature. After confirming the consumption of the starting pyrrole by TLC analysis, 180 mL CH2Cl2 were added and the mixture was transferred to a separatory funnel and washed twice with saturated aq. Na2CO3 and twice with saturated aq. NaCl. The solution was dried over anhydrous Na2SO4, filtered, and evaporated to dryness to yield crude 1,3-di-(1H-pyrrol-2-yl)propane-1,3-dione 2 (2.012 g, 66.4 %) as a pale yellow solid (95% pure by 1H-NMR). Crude 2 was used immediately without additional purification in the preparation of 3,5-di-(1H-pyrrol-2-yl)-1H-pyrazole 3. 1H-NMR (300MHz, DMSO-d6): (diketone 2 as a mixture of keto and enol tautomers in the ratio of 1:0.58 in DMSO-d6): keto form: 4.24 ppm (s, 2H, CH2), 6.20 (m, 2H, pyrrole-H), ), 7.07 (m, 2H, pyrrole-H), 7.11 (m, 2H, pyrrole- H), 11.86 (br s, 2H, NH),; enol form: 6.25 ppm (m, 2H, pyrrole-H), 6.66 (s, 1H, CH), 6.99 (m, 2H, pyrrole-H), 7.11 (m, 2H, pyrrole-H), 11.83 (br s, 2H, NH). These NMR data are essentially identical to the ones reported.15 13C-NMR (300MHz, acetone-d6): (diketone 2 as a mixture of keto and enol tautomers in the ratio of 1:1 in acetone-d6) 50.05, 90.65, 110.91, 110.96, 111.18, 111.23, 114.20, 114.23, 118.40, 124.60, 124.77, 126.18, 126.35, 129.72, 132.97, 176.99, 183.77. HRMS calcd. For C12H11N2O2 [MH+] 203.0821; found 203.0806.
3,5-Di-(1H-pyrrol-2-yl)-1H-pyrazole (3): To 0.226g ,1.12 mmol of 2 in 15 mL of dry THF and 15 mL of 2-propanol under argon, was added hydrazine hydrate (0.6 g , 12 mmol) and after reflux for 3 days, extraction with 1M HCl and neutralization with NaHCO3 afforded 3,5-di-(1H-pyrrol-2-yl)-1H-pyrazole 3, 0.167 g (75.5 %) after recrystallization from chloroform, mp 193 oC.18
1H-NMR (300MHz, acetone-d6): 6.14 (B, dd J=6,2 Hz, 2H), 6.47 (C, m, 2H), 6.61 (F, s, 1H), 6.84 (A, m, 2H), 10.4 (G, bs, 2H), 12.03 (H, bs, 1H). 13C-NMR (300MHz, acetone-d6): 97.01 (F), 106.85 (C), 109.58 (B), 119.49 (A)127.78 (D), 143.12 (E). HRMS calcd. For C11H10N4 [M+] 198.0905; found 198.0904.
1,3,3-Tri-(1H-pyrrol-2-yl)prop-2-en-1-one (4) (from 2 by recrystallization): Crude 1,3-di-(1H-pyrrol-2-yl)propane-1,3-dione (2) (0.050 g, 0.247 mmol devoid of pyrrole and of 4 according to 1H-NMR, TLC and ESI-MS) was dissolved in a minimal amount of refluxing CH2Cl2, two drops of hexane were added and the reaction mixture left at rt for an additional 2h. Then the mixture was kept cooled to -20 0C for 5 months and yellow crystals of pure 1,3,3-tri-(1H-pyrrol-2-yl)prop-2-en-1-one 4 (25.3 mg, 81.4 %), mp 169 oC were collected. 1H-NMR (600MHz, DMSO-d6): 6.20 (K, m, 1H), 6.25 (B, m, 1H), 6.28 (P, M, 1H), 6.49 (J, m, 1H),6.60 (O, m, 1H), 6.68 (G, s, 1H), 7.01 (L, m, 1H), 7.12 (A, m, 1H), 7.19 (Q, m, 1H), 7.21 (C, m, 1H), 11.54 (M, bs, 1H), 11.79 (E, bs, 1H), 13.5 (R, bs, 1H). 13C-NMR (600MHz, DMSO-d6): 109.11(K), 110.01 (B), 110.05 (P), 110.64 (G), 113.51 (J), 115.91 (C), 116.81(O), 121.32 (L), 122.11 (Q), 125.43 (A), 129.07 (N), 131.14 (I), 134.14 (D), 138.15 (H), 178.03 (F).
1H-NMR (600MHz, acetone-d6 and MeOD): 6.25 (P, M, 1H), 6.27 (B, m, 1H), 6.29 (K, m, 1H), 6.60 (O, m, 1H), 6.70 (J, m, 1H), 6.76 (G, s, 1H), 7.01 (Q, m, 1H), 7.14 (A, m, 1H), 7.14 (C, m, 1H), 7.17 (L, m, 1H), 10.67 (R, bs, 1H), 10.87 (E, bs, 1H). 13C-NMR (600MHz, acetone-d6 and MeOD): 110.01 (P), 111.07 (B), 111.13 (K), 111.61 (G), 113.73 (O), 116.14 (C), 118.54 (J), 121.37 (Q), 123.06 (L), 125.72 (A), 129.07 (N), 133.05 (I), 135.38 (D), 140.09 (H), 179.37 (F). UV/Vis (MeOH) λmax (ε)=437.5 nm (28,500). IR (CH2Cl2): 3390 (st, b), 3290 (s, b), 1642 (w), 1590 (s), 1501 (s), 1445 (st), 1388 (st), 1323 (w), 1100 (st), 1043 (st), 948 (w), 881 (w), 810 (w), 735 (st) cm-1. HRMS calcd. For C15H14N3O1 [MH+] 252.1137; found 252.1120.
1,3,3-Tri-(1H-pyrrol-2-yl)prop-2-en-1-one (4) (from 2 by chromatography): Crude 1,3-di-(1H-pyrrol-2-yl)propane-1,3-dione (2) (0.59 g, 2.94 mmol (in which pyrrole and 1,3,3-tri(1H-pyrrol-2-yl)prop-2-en-1-one were absent according to TLC) was dissolved in a minimal amount of (CH2Cl2 / MeOH = 97:3), a small amount of SiO2 was introduced and the mixture was evaporated to dryness and placed under high vacuum for 15 min. The above SiO2 mixture was placed on top of a SiO2 column and was chromatographed eluting once with hexane and then with (CH2Cl2/MeOH = 97:3) to yield pure 1,3,3-tri(1H-pyrrol-2-yl)prop-2-en-1-one 4 as the only product 71 % yield (0.2613 g). 1H-NMR, 13C-NMR, UV/Vis (MeOH), IR (CH2Cl2) and HRMS were identical to the spectra of 4 described above.
ACKNOWLEDGEMENT
Support of this research by the Marcus Center for Medicinal and Pharmaceutical Chemistry at Bar-Ilan University is gratefully acknowledged. We are grateful to Dr. H. E. Gottlieb and to Dr. Rachel Persky for valuable help with NMR and mass spectra respectively.
This paper is dedicated to Professor Emeritus K. Fukumoto on the occasion of his 75th birthday.
References
1. J. L. Sessler and E. Tomat, Acc. Chem. Res., 2007, 40, 371 and references therein. CrossRef
2. J. L. Sessler and D. Seidel, Angew. Chem. Int. Ed., 2003, 42, 5134. CrossRef
3. J. L. Sessler, T. D. Mody, and V. M. Lynch, J. Am. Chem. Soc., 1993, 115, 3346. CrossRef
4. J. L. Sessler, T. D. Mody, and V. M. Lynch, Inorg. Chem., 1992, 31, 529. CrossRef
5. J. L. Sessler and E. Tomat, T. D. Mody, V. M. Lynch, J. M. Veauthier, U. Mirsaidov, and J. T. Markert, Inorg. Chem., 2005, 44, 2125. CrossRef
6. J. L. Sessler, T. D. Mody, and V. M. Lynch, J. Am. Chem. Soc., 2006, 128, 4184. CrossRef
7. G. Givaja, A. J. Blake, C. Wilson, M. Schroder, and J. B. Love, Chem. Commun., 2003, 2508. CrossRef
8. J. M. Veauthier, W.-S. Cho, V. M. Lynch, and J. L. Sessler, Inorg. Chem., 2004, 43, 1220. CrossRef
9. S. Hannah, D. Seidel, J. L. Sessler, and V.M. Lynch, Inorg. Chim. Acta, 2001, 317, 211. CrossRef
10. F. H. Carre, R. J. P. Corriu, B. Geng, J. J. E. Moreau, and C. Vernhet, Organometallics, 1993, 12, 2478. CrossRef
11. M. S. Rodryguez-Morgade, B. Cabezo’n, S. Esperanza, and T. Torres, Chem. Eur. J., 2001, 7, 2407. CrossRef
12. A. Srinivasan, T. Ishizuka, A. Osuka, and H. Furuta, J. Am. Chem. Soc., 2003, 125, 878 and references therein. CrossRef
13. M. G. H. Vicente, M.T. Cancilla, C. B. Lebrilla, and K. M. Smith, Chem. Commun., 1998, 2355. CrossRef
14. M. O. Senge, M. G. H. Vicente, S. R. Parkin, H. Hope, and K. M. Z. Smith, Naturforsch., B: Chem. Sci., 1992, 23, 1189.
15. H. Maeda and Y. Kusunose, Chem. Eur. J., 2005, 11, 5661. CrossRef
16. Z. V. Stepanova, L. N. Sobenienina, A. I. Mikhaleva, I. A. Ushakov, N. N. Chipanina, V. N. Elokhina, V. K. Voronov, and B. A.Trofimov, Synthesis, 2004, 16, 2736. CrossRef
17. B. A. Trofimov, Z. V. Stepanova, L. N. Sobenienina, A. I. Mikhaleva, and I. A. Ushakov, Tetrahedron Lett., 2004, 45, 6513. CrossRef
18. The trifluoroacetic acid derivative of pyrazole 3 (63% yield) was prepared from 2 and hydrazine in refluxing acetic acid and chromatographic elution with TFA, see H. Maeda, Y. Ito, Y. Kusunose, and T. Nakanishi, Chem. Commun., 2007, 1136 CrossRef