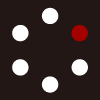
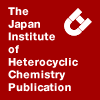
HETEROCYCLES
An International Journal for Reviews and Communications in Heterocyclic ChemistryWeb Edition ISSN: 1881-0942
Published online by The Japan Institute of Heterocyclic Chemistry
e-Journal
Full Text HTML
Received, 30th October, 2008, Accepted, 7th January, 2009, Published online, 7th January, 2009.
DOI: 10.3987/COM-08-S(D)74
■ Tandem Catalysis Strategy for Direct Glycosylation of 1-Hydroxy Sugars. Methoxyacetic Acid as an Effective Catalytic Mediator
Yasuo Yokoyama,* Takeshi Hanamoto, Shoko Suzuki, Kosuke Shimizu, Hiroshi Furuno, and Junji Inanaga*
Akita National College of Technology, Iijima-bunkyomachi 1-1, Akita, Akita 011-0923, Japan
Abstract
1-Hydroxy sugars were dehydratively coupled with a variety of alcohols including thiophenol in the presence of a catalytic amount of ytterbium(III) triflate [Yb(OTf)3] and methoxyacetic acid to give the corresponding glycosides in good to excellent yields, and in some cases, with high stereoselectivities. The reaction proceeds through selective esterification of 1-hydroxy sugars with methoxyacetic acid in the presence of free alcohols as glycosyl acceptors. The mechanism of methoxyacetic acid-mediated tandem catalysis with Yb(OTf)3 is discussed.INTRODUCTION
The glycosidic bond-forming reaction (glycosylation) is one of the most important and fundamental reactions in carbohydrate chemistry.1 In general, the reaction proceeds in two steps: activation of a glycosyl donor to form an oxycarbenium ion intermediate followed by nucleophilic attack of glycosyl acceptors (Scheme 1).
Since the classical Köenigs–Knorr synthesis,2 a vast number of methods have been developed for glycosylation, including, for example, 1-O-acylglycosides,1w,3 glycosyl halides,1,4 thioglycosides,1,5 trichloroacetoimidates,1,6 O-4'-pentenylglycosides,1w,7 and thiocarbonates1,8 as effective glycosyl donors. Although catalytic dehydrative glycosylation of 1-hydroxy sugars with free alcohols seem to be the most straightforward,9 such a methodology had not been reported until this and Mukaiyama’s groups independently demonstrated successful examples in 1993.10,11a And since then, intensive efforts by Mukaiyama’s group as well as other research groups have furthered advancement in this area.9,11
Most methods, however, required a stoichiometric or even an excess amount of activating reagents and/or the use of poisonous, explosive, or expensive catalysts or additives. On the other hand, our method using a Ln(OTf)3/methoxyacetic acid system (Ln=lanthanoid) is truly catalytic, in which methoxyacetic acid works as a catalytic mediator and water is the sole byproduct.10 In this paper, we disclose full details of this environmentally-friendly catalytic glycosylation.
RESULTS AND DISCUSSION
Previously, we found that 1-O-methoxyacetyl sugars could be an effective glycosyl donor that can be activated by a stoichiometric amount of zinc(II) ion12 or a catalytic amount of lanthanoid(III) ions13,14 (Scheme 2). Some of the results are collected in Table 1. In these reactions, the methoxyacetyl group is indispensable and seems to work as a bidentate ligand to form a metal ion-involved five membered-chelate thus weakening the C-1/oxygen glycosidic bond since almost no reaction occurred
when 1-O-acetyl analog was employed in place of 1-O-methoxyacetyl glucopyranose. The use of ytterbium(III) perfluorooctanesulfonate [Yb(OPf)3]15 and scandium(III) perfluorooctanesulfonate [Sc(OPf)3]15 was later found to be more effective (entries 10 and 11).15b
To have some theoretical support for the activation step, we carried out the semi empirical calculation study.16 As a model structure, α-acyloxytetrahydropyran was adopted and bond lengths (a–c) and dihedral angles (θ) of its derivatives were calculated for the optimized geometries by the MNDO-PM3 method.17 As shown in Table 2, the acetate and the methoxyacetate have essentially the same bond lengths and very similar dihedral angles (entry 1 vs. 3). On the other hand, when the acetyl group was activated by zinc ion, the bonds a and c became shorter (Δ0.036 Å and Δ0.066 Å, respectively) and the dihedral angle became smaller (θ=41.28˚) (entry 1 vs. 2). These changes indicate that bond a of the zinc ion-activated acetate has a somewhat double bond character. The effect is much more dramatic in the case of methoxyacetate: bonds a and c became much shorter (Δ0.072 Å and Δ0.148 Å, respectively) and bond b became longer (Δ0.095 Å) showing that the zinc ion-activated methoxyacetate group could be a good leaving group (entry 4). Such a short C–O bond length (a=1.361 Å) and the small dihedral angle (θ=26.43˚) strongly support the metal chelate-induced oxycarbenium ion character of the oxirane ring. Thus, the calculation clearly explains the experimental results, which conversely indicates the usefulness of such calculations in designing new glycosyl donors.
We further noticed that when excess 1-octanol (1.2 equiv) was used, a significant amount of octyl methoxyacetate was produced in addition to the desired glycoside (entry 6 in Table 1). This result suggests that Yb(OTf)3 catalyzes not only the glycosylation but also the esterification between 1-octanol and methoxyacetic acid, the latter of which would be liberated as glycosylation proceeds. Actually, the ester was produced almost quantitatively in a separate reaction under similar conditions. These findings prompted us to examine the possibility of the direct catalytic glycosylation of 1-hydroxy sugars with alcohols by the aid of methoxyacetic acid in an all-in-one system. A working hypothesis for the glycosylation is shown in Scheme 3.
We envisaged that the esterification of methoxyacetic acid with alcohols would occur first to give 1-O-methoxyacetyl sugar (catalysis i) and/or the corresponding methoxyacetic acid ester of glycosyl acceptor (catalysis iii), the latter of which might be transformed into the former by transesterification (catalysis iv).18 Then the desired glycosylation (catalysis ii) would take place to afford the final product releasing methoxyacetic acid to recycle. Thus, Yb(OTf)3 may catalyze all the possible reactions.
Based on this idea, the reaction of 2,3,4,6-tetra-O-benzyl-D-glucopyranose (1) with 1-octanol was
examined under various conditions (Table 3).10 As expected, the reaction proceeded smoothly in various solvents to give the glycoside 2 in good to excellent yields except in THF (entry 11) and no reaction took place when either one of the promoters was absent (entries 2 and 3). The quantity of the promoters could be reduced to 5 mol% each without serious decrease of the yield (entry 7). The ratio of Yb(OTf)3 to methoxyacetic acid did not affect yield or selectivity either. The reaction was effectively conducted in dichloromethane especially when liberated water from esterification was azeotropically removed by refluxing the solvent through a column of molecular sieves 4Å (entry 13). In all cases, the α-anomer of glycoside 2 was obtained as a major isomer, but the stereoselectivity was not high.
The optimized conditions were applied to the production of various glycosides including disaccharides, 6'-bromo-2'-naphthyl glycoside, and thioglycosides. The results are summarized in Table 4. When
partially protected sugars (3 and 5) were used as glycosyl acceptors in the glycosylation of 1, the corresponding disaccharides were obtained in good to excellent yields (entries 1 and 2). Secondary alcohols such as cyclohexanol, cholesterol, and 3β-cholestanol reacted with 1 under either condition A or B to give the desired products in excellent yields (entries 3–8). The protocol could also be successfully applied to the synthesis of O-arylglycosides and a thioglycoside (entries 9–12). In these reactions, production of α-anomers predominated except for the case of entry 2. On the other hand, highly β-selective glycosylation occurred when 2,3,5-tri-O-benzyl-D-ribofuranose (13, α/β=36:64) was used as a glycosyl donor. Especially noteworthy is the exclusive formation of β-D-gluco-D-ribofuranoside (entry 15), which indicates that the present glycosylation takes place in a non-stereospecific manner and proceeds through the formation of an oxycarbenium ion intermediate.
In order to have insight into the precise mechanism of the present glycosylation, several experiments were performed. First, the possibility of a trifluoromethanesulfonic acid (TfOH)-catalyzed process (eq. 1) was confirmed as there seemed to be an opportunity to liberate TfOH via equilibrium between methoxyacetic acid and Yb(OTf)3. As expected, TfOH was an effective catalyst for the glycosylation of compound 1 with 1-octanol (eq. 2).19 However, this route (eq. 1) may be excluded due to the following facts. First, the release of TfOH under equilibrium would be unfavorable, because of the large acidity difference between triflic acid (pKa ≈ –11) and methoxyacetic acid (pKa 2~4). Secondly, although the addition of potassium
methoxyacetate completely suppressed the catalytic activity of TfOH, Yb(OTf)3/methoxyacetic acid catalyzed glycosylation occurred in the presence of this salt (eq. 3 vs. 4).
As glycosylation step ii and esterification step iii in Scheme 3 had already been ascertained,13a,20 the transesterification step iv was tested by using 1 and octyl methoxyacetate (1.2 equiv) in the presence of Yb(OTf)3 (10 mol%) in refluxing dichloromethane for 24 h. However, no desired glycoside was produced under the conditions and the starting materials were recovered (eq. 5), which indicates that the transesterification process iv is not involved in the present glycosylation.
Next, we measured the rates of the reactions of methoxyacetic acid with various alcohols including 1-hydroxy sugar 1 and found that the consumption rate constants of 1, 4-phenylbutanol, and 4-tert-butylcyclohexanol were 1.82 x 10–3 s–1, 2.23 x 10–5 s–1, and 5.16 x 10–6 s–1, respectively. Their relative rates are compared in Figure 1. To our surprise, the consumption of 1 is more than 80 times faster than that of 4-phenylbutanol. The high reactivity of 1 may not be explained simply by its inherent nucleophilicity because the competitive acetylation of 1 and 4-phenylbutanol using acetic anhydride in the presence of 4-dimethylaminopyridine as a catalyst afforded the corresponding 1-O-acetyl sugar in only a slight predominance (1-O-acetyl sugar/4-phenylbutyl acetate=1.9:1). There must be some interaction between 1 and the catalyst to promote the esterification.
Thus, although the real mechanism of the Yb(OTf)3-catalyzed esterification step is still unclear, the actual catalytic cycle can be simplified as shown in Scheme 4: (1) methoxyacetylation of 1-hydroxy sugar (catalysis i) and (2) glycosylation of the resulting 1-O-methoxyacetyl sugar with a glycosyl acceptor (catalysis ii).
As a limitation of this protocol, O-acyl protected 1-hydroxy sugars did not react under the standard conditions. Also, the reaction of 1 or 2,3,5-tri-O-benzyl-D-ribofuranose in the absence of other alcohols did not afford the corresponding homo-coupling products such as trehalose. However, these facts in addition to the tandem catalysis process clarified in this study seem to indicate a novel and convenient access to homo and block copolymers via living polymerization of partially O-benzyl protected 1-hydroxy sugars like 2,3,4-tri-O-benzyl-D-glycopyranose. We are now exploring this possibility to develop a new area of oligosaccharide chemistry.
In conclusion, we have developed a novel and convenient method for the glycosylation of 1-hydroxy sugars with various alcohols using a catalytic amount of Yb(OTf)3 and methoxyacetic acid, in which efficient tandem catalysis takes place to produce water as the sole by-product. To the best of our knowledge, this is the first example of efficient tandem catalysis observed in glycosylation study and proved by the experiment. The kinetic study revealed that the reaction proceeds through the selective formation of 1-O-methoxyacetyl sugars followed by glycosylation, and the MNDO-PM3 calculation nicely explained the importance of methoxyacetyl group for the effective activation. Although the stereoselectivity is not always high, high chemical yields and the environmentally friendly waste-free process would be useful for convenient one-pot production of oligosaccharides.
EXPERIMENTAL
Melting points are uncorrected. 1H NMR Spectra were recorded at either 270 MHz (JEOL JNM-EX270) or 400 MHz (JEOL JNM-EX400). 13C NMR Spectra were recorded at 100 MHz JEOL JNM-EX400). Chemical shifts are given by δ relative to that of internal TMS. IR Spectra were recorded with JASCO IR-700 or PERKIN-ELMER FT-IR 1600. Mass Spectra (FABMS) were recorded with SHIMADZU/KRATOS CONCEPT 1S. Yb(OTf)3 was prepared from the corresponding oxide by refluxing them in aqueous trifluoromethanesulfonic acid, according to the literature.21 Yb(OPf)3 and Sc(OPf)3 were prepared according to the literature.15 2,3,4,6-Tera-O-benzyl-D-glucopyranose (1) and 2,3,5-tri-O-benzyl-D-ribofuranose (13) were prepared from the corresponding sugar derivatives, according to the literature.22 All solvents were purified before use: THF was distilled from sodium benzophenone or 9-fluorenone ketyl/dianion;23 toluene, C2H4Cl2, CH2Cl2, and MeCN were distilled from calcium hydride. All reactions were carried out under an atmosphere of argon or nitrogen. The reaction temperatures indicated are the bath temperatures. All reactions were monitored by TLC on 0.25 mm E. Merck silica gel plates (60F-254), where materials were detected by UV lamp and applying the 5% ethanolic solution of phosphomolybdenic acid and heat. The PM3 calculations were done with MOPAC program (ver. 6) in its ANCHOR IITM24 implementation. ANCHOR IITM was used to model the structures prior to the MOPAC geometry optimizations as well as to analyze the results of the calculations.
General procedure
Octyl 2,3,4,6-tetra-O-benzyl-D-glucopyranoside (2)
Method A: A mixture of 1 (540 mg, 1 mmol), Yb(OTf)3 (62 mg, 0.1 mmol), and 1-octanol (0.189 mL, 1.2 mmol) in dry C2H4Cl2 (20 mL) was warmed to 53 °C under argon, and to this mixture was added methoxyacetic acid (7.7 μL, 0.1 mmol). After stirring for 20 h, the reaction mixture was passed through a short column of silica gel and eluted with ether. Concentration of the eluate followed by purification by column chromatography (silica gel, hexane/AcOEt 5:1) afforded 647 mg (99%) of the corresponding glycoside as a mixture of anomers (α/β=62:38). This reaction can be also carried out in toluene, THF, or MeCN in a similar manner.
Method B: To a mixture of 1 (540 mg, 1 mmol), 1-octanol (0.189 mL, 1.2 mmol), and Yb(OTf)3 (62 mg, 0.1 mmol) in CH2Cl2 (40 mL) was added methoxyacetic acid (7.7 μL, 0.1 mmol) and this mixture was refluxed for 3 h under argon through a column of activated molecular sieves 4Å (ca. 28 g). The reaction mixture was passed through a short column of silica gel and eluted with ether. Concentration of the eluate followed by purification by column chromatography (silica gel, hexane/AcOEt 5:1) afforded 647 mg (99%) of the corresponding glycoside as a mixture of anomers (α/β=78:22). All physical data of the product fully agreed with the reported data.25,26
Methyl 6-O-(2',3',4',6'-tetra-O-benzyl-D-glucopyranosyl)-2,3,4-tri-O-benzyl-α-D-glucopyranoside (4).23
This compound was prepared from 1 and 3 by method B in 99% yield (α/β=75:25).
1,2:5,6-Di-O-isopropylidene-3-O-(2',3',4',6'-tetra-O-benzyl-D-glucopyranosyl)-α-D-glucofuranoside (6).27
This compound was prepared from 1 and 5 by the method A in 81% yield (α/β=40:60).
Cyclohexyl 2,3,4,6-tetra-O-benzyl-D-glucopyranoside (7).25,28
This compound was prepared from 1 and cyclohexanol by the method A (98% yield, α/β=68:32) or the method B (99% yield, α/β=68:32).
Cholesteryl 2,3,4,6-tetra-O-benzyl-D-glucopyranoside (8).29
This compound was prepared from 1 and cholesterol by the method A (85% yield, α/β=64:36) or the method B (99% yield, α/β = 67/33).
3β-Cholestanyl 2,3,4,6-tetra-O-benzyl-D-glucopyranoside (9).25
This compound was prepared from 1 and 3β-cholestanol by the method A (97% yield, α/β=74:26) or the method B (99% yield, α/β = 72/28).
6'-Bromo-2'-naphthyl 2,3,4,6-tetra-O-benzyl-D-glucopyranoside (10).
This compound was prepared from 1 and 6-bromo-2-naphthol by the method B in 72% yield (α/β = 82/18). The α-anomer and the β-anomer were separated by TLC on silica gel with 6:1 hexane–AcOEt (two developments).
The α-anomer: Colorless solid. Mp 91.5–92 ˚C. Rf=0.30 (hexane/AcOEt 6:1). IR (neat) ν: 2898, 1649, 1633, 1622, 1496, 1205, 1067, 910, 748, 696, 473 cm–1. 1H NMR (400 MHz, CDCl3, δ): 7.93 (d, 1H, J=1.71 Hz), 7.67 (d, 1H, J=9.03 Hz), 7.55 (d, 1H, J=8.79 Hz), 7.49 (dd, 1H, J=1.71, 8.79 Hz), 7.42–7.22 (m, 20H), 7.14–7.11 (m, 2H), 5.57 (d, 1H, J=3.17 Hz), 5.08 (1/2 AB q, 1H, J=10.74 Hz), 4.92 (1/2 AB q, 1H, J=10.74 Hz), 4.87 (1/2 AB q, 1H, J=10.74 Hz), 4.84 (1/2 AB q, 1H, J=12.21 Hz), 4.68 (1/2 AB q, 1H, J=12.21 Hz), 4.59 (1/2 AB q, 1H, J=11.96 Hz), 4.48 (1/2 AB q, 1H, J=10.74 Hz), 4.39 (1/2 AB q, 1H, J=11.96 Hz), 4.24 (t, 1H, J=9.03 Hz), 3.89–3.76 (m, 3H), 3.72 (dd, 1H, J=3.17, 10.74 Hz), 3.56 (dd, 1H, J=1.71, 10.74 Hz). 13C NMR (100 MHz, CDCl3, δ): 154.43, 138.55, 137.90, 137.74, 137.46, 132.62, 130.55, 129.48, 129.46, 128.67, 128.38, 128.34, 128.28, 128.22, 127.93, 127.85, 127.83, 127.77, 127.65, 127.64, 127.57, 120.04, 117.68, 110.58, 95.34, 81.88, 79.59, 77.21, 75.80, 75.14, 73.41, 73.33, 70.83, 68.05. [α]D15 +163.6˚ (c 1.00, CHCl3). FABMS m/z (%): 768 (7) [M+Na], 181 (93), 154 (100), 136 (82), 107 (36). Anal. Calcd for C44H41O6Br: C, 70.87; H, 5.50. Found: C, 70.66; H, 5.68.
The β-anomer: Colorless solid. Mp 143.5–144 °C. Rf=0.35 (hexane/AcOEt 6:1). IR (KBr) ν: 3031, 2868, 1589, 1499, 1353, 1208, 1066, 911, 696, 474 cm–1. 1H NMR (400 MHz, CDCl3, δ): 7.94 (s, 1H), 7.67 (d, 1H, J=8.79 Hz), 7.46 (s, 2H), 7.39–7.20 (m, 22H), 5.13 (d, 1H, J=7.08 Hz), 5.07 (1/2 AB q, 1H, J=10.74 Hz), 4.97 (1/2 AB q, 1H, J=10.74 Hz), 4.89–4.83 (m, 3H), 4.60–4.51 (m, 3H), 3.85–3.76 (m, 3H), 3.72–3.68 (m, 3H). 13C NMR (100 MHz, CDCl3, δ): 155.20, 138.26, 137.97, 137.83, 137.76, 132.66, 130.75, 129.51, 129.47, 128.84, 128.45, 128.35, 128.34, 128.27, 128.11, 127.88, 127.79, 127.73, 127.67, 127.63, 127.55, 119.90, 117.83, 111.15, 101.54, 84.61, 81.97, 77.66, 75.80, 75.17, 75.11, 75.07, 73.50, 68.90. [α]D15 –4.1˚ (c 0.83, CHCl3). FABMS m/z (%): 768 (7) [M+Na], 181 (80), 154 (100), 136 (82), 107 (36). Anal. Calcd for C44H41O6Br: C, 70.87; H, 5.50. Found: C, 70.97; H, 5.69.
Phenyl 2,3,4,6-tetra-O-benzyl-D-glucopyranoside (11).30
This compound was prepared from 1 and phenol by the method B in 98% yield (α/β=75:25).
Phenyl 2,3,4,6-tetra-O-benzyl-1-thio-D-glucopyranoside (12).31
This compound was prepared from 1 and thiophenol by the method A (97% yield, α/β=73:27) or the method B (99% yield, α/β=63:37).
Octyl 2,3,5-tri-O-benzyl-D-ribofuranoside (14).32
This compound was prepared from 13 and 1-octanol by the method B in 99% yield (α/β=8:92).
3β-Cholestanyl 2,3,5-tri-O-benzyl-D-ribofuranoside (15).33
This compound was prepared from 13 and 3β-cholestanol by the method B in 98% yield (α/β=4:96).
Methyl 6-O-(2',3',5'-tri-O-benzyl-β-D-ribofuranosyl)-2,3,4-tri-O-benzyl-α-D-glucopyranoside (16).33
This compound was prepared from 13 and 3 by the method B in 92% yield (β only).
Phenyl 2,3,5-tri-O-benzyl-1-thio-D-ribofuranoside (17).34
This compound was prepared from 13 and thiophenol by the method B in 96% yield (α/β=25:75).
Determination of the consumption rate of alcohols in the Yb(OTf)3-catalyzed esterification with methoxyacetic acid
Determination of the consumption rate of (1)
A mixture of 1 (105.2 mg, 0.2 mmol), methoxyacetic acid (18 μL, 0.23 mmol), Yb(OTf)3 (12 mg, 0.1 mmol) and diphenylmethane (32.8 mg, 0.2 mmol, internal standard) in CH2Cl2 (30 mL) were stirred under reflux. After 0, 5, 10, 15, 20, and 30 min, an aliquot of the reaction mixture was analyzed by HPLC to see the remaining amount of 1; t1/2=0.1 h.
Determination of the consumption rate of 4-phenylbutanol
A mixture of 4-phenylbutanol (60 mg, 0.4 mmol), methoxyacetic acid (36 μL, 0.46 mmol), Yb(OTf)3 (24 mg, 0.2 mmol) and diphenyl methane (32.8 mg, 0.2 mmol, internal standard) in CH2Cl2 (60 mL) were stirred under reflux. After 0, 0.5, 1, 2, 4, 6, 12, and 24 h, an aliquot of the reaction mixture was analyzed by HPLC to see the remaining amount of 4-phenylbutanol; t1/2=8.7 h.
Determination of the consumption rate of 4-tert-butylcyclohexanol
A mixture of 4-tert-butylcyclohexanol (62.5 mg, 0.4 mmol), methoxyacetic acid (36 μL, 0.46 mmol), Yb(OTf)3 (24 mg, 0.2 mmol) and diphenylmethane (32.8 mg, 0.2 mmol, internal standard) in CH2Cl2 (60 mL) were stirred under reflux. After 0, 6, 9, 14, and 25 h, an aliquot of the reaction mixture was analyzed by HPLC to see the remaining amount of 4-tert -butylcyclohexanol; t1/2=37 h.
ACKNOWLEDGEMENTS
This work was supported by a Grant-in-Aid for Scientific Research from the Ministry of Education, Culture, Sports, Science, and Technology, Japan and also by “Joint Project of Chemical Synthesis Core Research Institutions”. We are grateful to Professor Teruo Shinmyozu of this institute for access to the SUN computer (3-4/IX) and program packages (ANCHOR IITM and MOPAC ver. 6).
References
1. For some selected reviews, see: (a) H. Hojo and Y. Nakahara, Biopolymers, 2007, 88, 308; CrossRef (b) K. Toshima, Carbohydr. Res., 2006, 341, 1282; CrossRef (c) M. Jacobsson, J. Malmberg, and U. Ellervik, Carbohydr. Res., 2006, 341, 1266; CrossRef (d) E. S. H. El Ashry, L. F. Awad, and A. I. Atta, Tetrahedron, 2006, 62, 2943; CrossRef (e) P. Fugedi, Org. Chem. Sugars, 2006, 89; (f) M. P. Murrell, K. J. Yarema, and A. Levchenko, Handbook of Carbohydr. Eng., 2005, 247; (g) J. D. C. Codee, R. E. J. N. Litjens, L. J. Van den Bos, H. S. Overkleeft, and G. A. Van der Marel, Chem. Soc. Rev., 2005, 34, 769; CrossRef (h) C. Rupprath, T. Schumacher, and L. Elling, Current Med. Chem., 2005, 12, 1637; CrossRef (i) H. Pellissier, Tetrahedron, 2005, 61, 2947; CrossRef (j) B. Yu, Z. Yang, and H. Cao, Current Org. Chem., 2005, 9, 179; CrossRef (k) R. S. Haltiwanger and J. B. Lowe, Ann. Rev. Biochem., 2004, 73, 491; CrossRef (l) D. K. Ress and R. J. Linhardt, Current Org. Synth., 2004, 1, 31; CrossRef (m) Z. Gyorgydeak and I. F. Pelyvas, Glycoscience, 2001, 1, 691; (n) M. Sekine, Glycoscience, 2001, 1, 673; (o) S. Oscarson, Glycoscience, 2001, 1, 643; (p) K. Toshima, Glycoscience, 2001, 1, 583; (q) G. Boons, Glycoscience, 2001, 1, 551; (r) G. Magnusson and U. Nilsson, Glycoscience, 2001, 2, 1543; (s) S. Shoda, Glycoscience, 2001, 2, 1465; (t) J. F. Ernst and S. K.-H. Prill, Med. Mycology, 2001, 39, 67; (u) L. Panza and L. Lay, Carbohydr. Chem. Biol., 2000, 1, 195; CrossRef (v) J. Tamura, Carbohydr. Chem. Biol., 2000, 1, 177; CrossRef (w) B. Fraser-Reid, G. Anilkumar, M. R. Gilbert, S. Joshi, and R. Kraehmer, Carbohydr. Chem. Biol., 2000, 1, 135; CrossRef (x) Z. Zhang and C. Wong, Carbohydr. Chem. Biol., 2000, 1, 117; CrossRef (y) K. Toshima, Carbohydr. Res., 2000, 327, 15; CrossRef (z) P. Sinaÿ and J.-M. Mallet, Frontiers in Natural Product Res., 1996, 1, 130.
2. W. Köenigs and E. Knorr, Chem. Ber., 1901, 34, 957.
3. (a) J. C. Lopez and B. Fraser-Reid, J. Chem. Soc., Chem. Commun., 1991, 159; CrossRef (b) H. Kunz, P. Wernig, and M. Schultz, Synlett, 1990, 631. CrossRef
4. (a) R. D. Groneberg, T. Miyazaki, N. A. Stylianides, T. J. Schulze, W. Stahl, E. P. Schreiner, T. Suzuki, Y. Iwabuchi, A. L. Smith, and K. C. Nicolaou, J. Am. Chem. Soc., 1993, 115, 7593; CrossRef (b) H. Yamada and M. Nishizawa, Tetrahedron, 1992, 48, 3021; CrossRef (c) J. Junnemann, I. Lundt, and J. Theiem, Liebigs Ann. Chem., 1991, 759; CrossRef (d) M. Yamaguchi, A. Horiguchi, A. Fukuda, and T. Minami, J. Chem. Soc., Perkin Trans. 1, 1990, 1079; CrossRef (e) K. Suzuki and T. Matsumoto, Yuki Gosei Kagaku Kyokaishi, 1990, 48, 1026; (f) K. Suzuki, H. Maeta, T. Suzuki, and T. Matsumoto, Tetrahedron Lett., 1989, 30, 6879; CrossRef (g) T. Matsumoto, M. Katsuki, and K. Suzuki, Chem. Lett., 1989, 437; CrossRef (h) K. Suzuki, H. Maeta, and T. Matsumoto, Tetrahedron Lett., 1989, 30, 4853; CrossRef (i) K. Suzuki, H. Maeta, T. Matsumoto, and G. Tsuchihashi, Tetrahedron Lett., 1988, 29, 3571; CrossRef (j) M. Kreuzer and J. Thiem, Carbohydr. Res., 1986, 149, 347; CrossRef (k) S. Hashimoto, M. Hayashi, and R. Noyori, Tetrahedron Lett., 1984, 25, 1379; CrossRef (l) K. C. Nicolaou, R. E. Dolle, A. Chucholowski, and J. L. Randall, J. Chem. Soc. Chem. Commun., 1984, 1153. CrossRef
5. (a) N. K. Kochetkov, N. N. Malysheva, E. M. Klimov, and A. V. Demchenko, Tetrahedron Lett., 1992, 33, 381; CrossRef (b) N. K. Kochetkov, E. M. Klimov, N. N. Malysheva, and A. V. Demchenko, Carbohydr. Res., 1991, 212, 77; CrossRef (c) H. B. Mareyala and G. V. Reddy, Tetrahedron, 1991, 47, 9721; CrossRef (d) H. M. Zuurmond, S. C. van der Laan, G. A. van der Marel, and J. H. van Boom, Carbohydr. Res., 1991, 215, C1; CrossRef (e) M. Sasaki and K. Tachibana, Tetrahedron Lett., 1991, 32, 6873; CrossRef (f) W. R. Roush, X. Lin, and J. A. Straub, J. Org. Chem., 1991, 56, 1649; CrossRef (g) J. H. van Boom and G. H. Veeneman, Tetrahedron Lett., 1990, 31, 275; CrossRef (h) G. H. Veeneman, S. H. van Leeuwen, and J. H. van Boom, Tetrahedron Lett., 1990, 31, 1331; CrossRef (i) J. O. Kihlberg, D. A. Leigh, and D. R. Bundle, J. Org. Chem., 1990, 55, 2860; CrossRef (j) Y. Ito, T. Ogawa, M. Numata, and M. Sugimoto, Carbohydr. Res., 1990, 202, 165; CrossRef (k) Y. Ito and T. Ogawa, Tetrahedron Lett., 1988, 29, 1061; CrossRef (l) H. Lönn, J. Carbohydr. Chem., 1987, 6, 301. CrossRef
6. (a) J. Geiger, B. G. Reddy, G. A. Winterfeld, R. Weber, M. Przybylski, and R. R. Schmidt, J. Org. Chem., 2007, 72, 4367; CrossRef (b) J. Geiger, N. Barroca, and R. R. Schmidt, Synlett, 2004, 836; (c) S. Figueroa-Perez and R. R. Schmidt, Carbohydr. Res., 2000, 328, 95; CrossRef (d) F. Loussel, L. Knerr, M. Grathwohl, and R. R. Schmidt, Org. Lett., 2000, 2, 3043; CrossRef (e) R. R. Schmidt and W. Kinzy, Adv. Carbohydr. Chem. and Biochem., 1994, 50, 21; CrossRef (f) J. L. Maloisel, A. Vasella, B. M. Trost, and D. L. van Vranken, Helv. Chim. Acta, 1992, 75, 1515; CrossRef (g) J. L. Maloisel, A. Vasella, B. M. Trost, and D. L. van Vranken, J. Chem. Soc., Chem. Commun., 1991, 1099; CrossRef (h) F. J. Urban, B. S. Moore, and R. Breitenbach, Tetrahedron Lett., 1990, 31, 4421; CrossRef (i) M. Kobayashi, F. Yamazaki, Y. Ito, and T. Ogawa, Tetrahedron Lett., 1989, 30, 4547; CrossRef (j) F. Yamazaki, T. Nukada, Y. Ito, S. Sato, and T. Ogawa, Tetrahedron Lett., 1989, 30, 4417; CrossRef (k) S. Sato, S. Nunomura, T. Nakano, Y. Ito, and T. Ogawa, Tetrahedron Lett., 1988, 29, 4097; CrossRef (l) S. Sato, Y. Ito, and T. Ogawa, Tetrahedron Lett., 1988, 29, 5267; CrossRef (m) S. Nunomura and T. Ogawa, Tetrahedron Lett., 1988, 29, 5681; CrossRef (n) W. Kinzy and R. R. Schmidt, Carbohydr. Res., 1987, 164, 265; CrossRef (o) K. C. Nicolaou, T. K. Chakraborty, R. A. Daines, and Y. Ogawa, J. Chem. Soc., Chem. Commun., 1987, 686; CrossRef (p) K. C. Nicolaou, T. K. Chakraborty, Y. Ogawa, R. A. Daines, N. S. Simpkins, and G. T. Furst, J. Am. Chem. Soc., 1988, 110, 4660. CrossRef
7. (a) P. Konradsson, D. R. Mootoo, R. E. McDevitt, and B. Fraser-Reid, J. Chem. Soc., Chem. Commun., 1990, 270; CrossRef (b) A. J. Ratcliffe, P. Konradsson, and B. Fraser-Reid, J. Am. Chem. Soc., 1990, 112, 5665; CrossRef (c) J. M. Llera, J. C. Lopez, and B. Fraser-Reid, J. Org. Chem., 1990, 55, 2997; CrossRef (d) D. R. Mootoo and B. Fraser-Reid, Tetrahedron Lett., 1989, 30, 2363; CrossRef (e) D. R. Mootoo, P. Konradsson, and B. Fraser-Reid, J. Am. Chem. Soc., 1989, 111, 8540. CrossRef
8. (a) H. Lönn and K. Stenvall, Tetrahedron Lett., 1992, 33, 115; CrossRef (b) W. Birberg and H. Lönn, Tetrahedron Lett., 1991, 32, 7453; CrossRef (c) W. Birberg and H. Lönn, Tetrahedron Lett., 1991, 32, 7457; CrossRef (d) A. Marra, F. Gauffeny, and P. Sinaÿ, Tetrahedron, 1991, 47, 5149; CrossRef (e) M. J. Ford and S. V. Ley, Synlett, 1990, 255; CrossRef (f) M. J. Ford, J. G. Knight, S. V. Ley, and S. Vile, Synlett, 1990, 331; CrossRef (g) S. V. Ley, A. Armstrong, D. Diez-Martin, M. J. Ford, P. Grice, J. G. Knight, H. C. Kolb, A. Madin, and C. A. Marby, J. Chem. Soc., Perkin Trans. 1, 1991, 667; CrossRef (h) A. Marra, L. K. S. Shun, F. Gauffeny, and P. Sinaÿ, Synlett, 1990, 445. CrossRef
9. B. A. Garcia and D. Y. Gin, J. Am. Chem. Soc., 2000, 122, 4269, and references therein. CrossRef
10. J. Inanaga, Y. Yokoyama, and T. Hanamoto, J. Chem. Soc., Chem. Commun., 1993, 1090.
11. (a) N. Shimomura and T. Mukaiyama, Chem. Lett., 1993, 1941; (b) T. Mukaiyama, K. Matsubara, and M. Hora, Synthesis, 1994, 1368; CrossRef (c) N. H. Uchiro and T. Mukaiyama, Chem. Lett., 1996, 271; CrossRef (d) H. Uchiro, K. Miyazaki, and T. Mukaiyama, Chem. Lett., 1997, 403; CrossRef (e) J. Matsuo, T. Shirahata, and S. Omura, Tetrahedron Lett., 2006, 47, 267; CrossRef (f) Y. Shingu, A. Miyachi, Y. Miura, K. Kobayashi, and Y. Nishida, Carbohydr. Res., 2005, 340, 2236; CrossRef (g) M. Hirooka, Y. Mori, A. Sasaki, S. Koto, Y. Shinoda, and A. Morinaga, Bull. Chem. Soc. Jpn., 2001, 74, 1679; CrossRef (h) S. Koto, A. Kusunoki, and M. Hirooka, Bull. Chem. Soc. Jpn., 2000, 73, 967, and references therein. CrossRef
12. J. Inanaga, Y. Yokoyama, and T. Hanamoto, Chem. Exp., 1993, 8, 165.
13. (a) J. Inanaga, Y. Yokoyama, and T. Hanamoto, Tetrahedron Lett., 1993, 34, 2791; CrossRef (b) J. Inanaga, Y. Yokoyama, and T. Hanamoto, Mem. Fac. Sci., Kyushu Univ., Ser. C, Chem., 1994, 19, 125.
14. For some selected examples of lanthanoid(III) trifluoromethanesulfonate-catalyzed glycosylation, see: (a) S. Sato, Y. Naito, and K. Aoki, Carbohydr. Res., 2007, 342, 913; CrossRef (b) K. N. Jayaprakash and B. Fraser-Reid, Carbohydr. Res., 2007, 342, 490; CrossRef (c) S.-J. Hsu, H.-C. Lin, and C.-H. Lin, Carbohydr. Res., 2006, 341, 1428; CrossRef (d) M. Adinolfi, A. Iadonisi, and A. Ravida, Synlett, 2006, 583; (e) T. Yamauchi, Y. Watanabe, K. Suzuki, and T. Matsumoto, Synthesis, 2006, 2818; (f) Y. Yoshimura, H. Shimizu, H. Hinou, and S. Nishimura, Tetrahedron Lett., 2005, 46, 4701; CrossRef (g) M. Adinolfi, A. Iadonisi, A. Ravida, and M. Schiattarella, J. Org. Chem., 2005, 70, 5316; CrossRef (h) E. Kaji, E. Kurimoto, R. Saiga, A. Matsuura, K. Harada, and T. Nishino, Heterocycles, 2005, 66, 453; CrossRef (i) T. Yamauchi, M. Shigeta, T. Matsumoto, and S. Suzuki, Heterocycles, 2005, 66, 153; CrossRef (j) T. Yamanoi, Y. Oda, I. Yamazaki, M. Shinbara, K. Morimoto, and S. Matsuda, Lett. Org. Chem., 2005, 2, 242; CrossRef (k) K. N. Jayaprakash and B. Fraser-Reid, Org. Lett., 2004, 6, 4211; CrossRef (l) M. Adinolfi, A. Iadonisi, A. Ravida, and M. Schiattarella, Tetrahedron Lett., 2004, 45, 4485; CrossRef (m) K. N. Jayaprakash and B. Fraser-Reid, Synlett, 2004, 301; (n) A. Ben, T. Yamauchi, T. Matsumoto, and K. Suzuki, Synlett, 2004, 225; (o) J. S. Yadav, B. V. S. Reddy, and V. Geetha, Synth. Commun., 2003, 33, 717; CrossRef (p) M. Adinolfi, G. Barone, A. Iadonisi, and M. Schiattarella, Tetrahedron Lett., 2002, 43, 5573; CrossRef (q) J. S. Yadav, B. V. S. Reddy, and P. K. Chand, Tetrahedron Lett., 2001, 42, 4057; CrossRef (r) M. Takhi, R. A. A.-H. Abdel, and R. R. Schmidt, Tetrahedron Lett., 2001, 42, 4053; CrossRef (s) M. Adinolfi, G. Barone, A. Iadonisi, L. Mangoni, and M. Schiattarella, Tetrahedron Lett., 2001, 42, 5967; CrossRef (t) N. Hashizume and S. Kobayashi, Carbohydr. Lett., 1996, 2, 157; (u) W.-S. Kim, S. Hosono, H. Sasai, and M. Shibasaki, Heterocycles, 1996, 42, 795; CrossRef (v) S. Hosono, W.-S. Kim, H. Sasai, and M. Shibasaki, J. Org. Chem., 1995, 60, 4. CrossRef
15. (a) J. Inanaga, Y. Sugimoto, and T. Hanamoto, New J. Chem., 1995, 19, 707; (b) T. Hanamoto, Y. Sugimoto, Y. Z. Jin, and J. Inanaga, Bull. Chem. Soc. Jpn., 1997, 70, 1421. CrossRef
16. J. Inanaga, Y. Yokoyama, and T. Hanamoto, Mem. Fac. Sci., Kyushu Univ., Ser. C, Chem., 1993, 18, 29.
17. (a) G. B. Rocha, R. O. Freire, A. M. Simas, and J. J. P. Stewart, J. Comput. Chem., 2006, 27, 1101; CrossRef (b) J. J. P. Stewart, J. Mole. Model., 2004, 10, 155; CrossRef (c) J. J. P. Stewart, J. Comput. Chem., 1989, 10, 221; CrossRef (d) J. J. P. Stewart, Rev. Comput. Chem., 1990, 45; (e) J. J. P. Stewart, J. Comput.-Aided Mol. Design, 1990, 4, 1; CrossRef (f) J. J. P. Stewart, J. Comput. Chem., 1989, 10, 209. CrossRef
18. T. Hanamoto, Y. Sugimoto, Y. Yokoyama, and J. Inanaga, J. Org. Chem., 1996, 61, 4491. CrossRef
19. H. P. Wessel, J. Carbohydr. Chem., 1988, 7, 263. CrossRef
20. J. Inanaga, Y. Yokoyama, and T. Hanamoto, Nihon Kagakukaishi, 1993, 516.
21. (a) J. H. Forsberg, V. Spaziano, T. M. Balasubramanian, G. K. Liu, S. A. Kinsley, C. A. Duckworth, J. J. Poteruca, P. S. Brown, and J. L. Miller, J. Org. Chem., 1987, 52, 1017; CrossRef (b) J. H. Forsberg, T. M. Kubik, T. Moeller, and K. Gucwa, Inorg. Chem., 1971, 10, 2656; CrossRef (c) J. H. Forsberg and A. C. Wathen, Inorg. Chem., 1971, 10, 1379; CrossRef (d) J. H. Forsberg and T. Moeller, Inorg. Chem., 1969, 8, 889; CrossRef (e) J. H. Forsberg and T. Moeller, Inorg. Chem., 1969, 8, 883. CrossRef
22. 2,3,4,6-Tetra-O-benzyl-α-D-glucose (1) was synthesized from the corresponding methyl glucose. See; O. T. Schmidt, T. Auer, and H. Schmadel, Chem. Ber., 1960, 93, 556. ; 2,3,5-Tri-O-benzyl-D-ribofuranose (13) was synthesized from the corresponding methyl ribose. See; R. R. Schmidt, J. Karg, and W. Guilliard, Chem. Ber., 1977, 110, 2433 . CrossRef
23. (a) M. Kamaura and J. Inanaga, Tetrahedron Lett., 1999, 40, 7347; CrossRef (b) M. Kamaura, T. Hanamoto, Y. Kuwatani, and J. Inanaga, J. Am. Chem. Soc., 1999, 121, 6320. CrossRef
24. ANCHOR IITM is a molecular design support system for SUN computers from FUJITSU Limited and Kureha Chemical Company, 1992.
25. S. Koto, N. Morishima, and S. Zen, Bull. Chem. Soc. Jpn., 1982, 55, 1543. CrossRef
26. T. Yamanoi, S. Nagayama, H. Ishida, J. Nishikido, and T. Inazu, Synth. Commun., 2001, 31, 899. CrossRef
27. V. Dourtoglou and B. Gross, J. Carbohydr. Chem., 1983, 2, 57. CrossRef
28. H.-P. Knoben, U. Schlueter, and H. Redlich, Carbohydr. Res., 2004, 339, 2821. CrossRef
29. (a) S. Hotha and S. Kashyap, J. Am. Chem. Soc., 2006, 128, 9620; CrossRef (b) H. Vankayalapati and G. I. Tranoy, Tetrahedron: Asymmetry, 2001, 12, 1373. CrossRef
30. J. Gervay and M. J. Hadd, J. Org. Chem., 1997, 62, 6961. CrossRef
31. (a) S. K. Madhusudan, G. Agnihotri, D. S. Negi, and A. K. Misra, Carbohydr. Res., 2005, 340, 1373; CrossRef (b) A. Fuerstner, Liebigs Ann. Chem., 1993, 1211; CrossRef (c) R. J. Ferrier and R. H. Furneaux, Carbohydr. Res., 1976, 52, 63. CrossRef
32. T. Suzuki, S. Watanabe, T. Yamada, and K. Hiroi, Tetrahedron Lett., 2003, 44, 2561. CrossRef
33. S. Suda and T. Mukaiyama, Bull. Chem. Soc. Jpn., 1993, 66, 1211. CrossRef
34. T. Kametani, K. Kawamura, and T. Honda, J. Am. Chem. Soc., 1987, 109, 3010. CrossRef