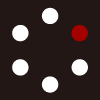
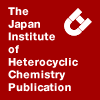
HETEROCYCLES
An International Journal for Reviews and Communications in Heterocyclic ChemistryWeb Edition ISSN: 1881-0942
Published online by The Japan Institute of Heterocyclic Chemistry
e-Journal
Full Text HTML
Received, 30th October, 2008, Accepted, 15th December, 2008, Published online, 16th December, 2008.
DOI: 10.3987/COM-08-S(D)76
■ Synthesis of Macrocyclic Lactams from 2-(ω-Aminoalkyl)-2-benzoylamino-3-phenyl-N,N-dimethylpropanamides via Direct Amide Cyclization
Stephan P. Fritschi, Anthony Linden, and Heinz Heimgartner*
Institute of Organic Chemistry, University of Zürich, Winterthurerstrasse 190, CH-8057 Zürich, Switzerland
Abstract
The alkylation of 4-benzyl-2-phenyl-1,3-oxazol-5(4H)-one (11) with ω-azidoalkyl iodides (12) by deprotonation with LDA in THF/HMPT at –78 °C yielded mixtures of the 4,4-disubstituted 1,3-oxazol-5(4H)-ones (13) and the O-alkylated 1,3-oxazoles (14) in 65–50%, with 13 as the major product. The reaction of the latter with dimethylamine in acetonitrile at room temperature led to ω-azido-2-benzamido-2-benzylalkane amides (15), which were reduced to give the corresponding ω-amino derivatives (16). On treatment with HCl gas or BF3 in boiling toluene, the macrocyclic 2-benzamido lactams (18) were formed in up to 27% yield via the intermediate formation of 1,3-oxazol-5(4H)-ones (17). The structures of the 14- and 15-membered lactams have been established by X-ray crystallography.INTRODUCTION
In the last couple of years, we have investigated extensively the use of 2,2-disubstituted 2H-azirin-3-amines (1) as synthons for α,α-disubstituted α-amino acids in peptide synthesis.2–16 The basic concept is the so-called ‘azirine/oxazolone method’,17–22 in which both the selective hydrolysis of the terminal amide group of 2, obtained via extension of the peptide chain by the reaction with the 2H-azirin-3-amine (1), to give 4, and the coupling with the next amino acid or peptide segment to yield 5 occur via 1,3-oxazol-5(4H)-ones (azlactones, 3) as intermediates (Scheme 1, see also refs.23,24). In a very recent study, Brückner and coworkers pointed to the high tendency of peptides containing α-aminoisobutyric acid (Aib) to undergo acid-catalyzed cleavage of the peptide chain via the formation of 1,3-oxazol-5(4H)-ones.25
Furthermore, it has been shown that the cyclization of peptide-like compounds of type (6) by treatment with dry HCl gas in toluene at 100 °C proceeds smoothly (‘direct amide cyclization’), and cyclic depsipeptides (7) are formed in astonishingly high yield (Scheme 2).9,26–30 Again, the ‘activated intermediates’ are 1,3-oxazol-5(4H)-ones, which are formed via acid-catalyzed cyclization of the C-terminal acyl-Aib-NMe2 unit.
Under different reaction conditions, i.e., by the treatment of penta to octapeptides containing α,α-disubstituted glycines and deprotected H2N– and –COOH termini with peptide coupling reagents, the synthesis of the corresponding cyclopeptides could be achieved.3,12–14,31 Also in these cases, 1,3-oxazol-5(4H)-ones are likely intermediates in the cyclization reaction.
The above ring closing reactions to cyclodepsipeptides and cyclopeptides can be characterized by the sequence 8 → 9 (Scheme 3), in which the nucleophilic group in the side chain at C(2) of the 1,3-oxazol-5(4H)-one (8, R3 = HNu~~) attacks C(5) of 8, followed by ring opening. The intermolecular version of this reaction, e.g., the ring opening of 1,3-oxazol-5(4H)-ones via hydrolysis and methanolysis, is well known32–36 (for some relevant examples, see refs.37–41). The preferred reaction mechanism is the nucleophilic attack at C(5), but, depending on steric and electronic effects of the substitutens, the nucleophilic attack of H2O can also occur at C(2), leading to the same product.42,43 Similarly, the reactions of 1,3-oxazol-5(4H)-ones with N-nucleophiles lead to α-(N-acylamino) alkanamides or peptides via attack at C(5).43–45
Some years ago, we demonstrated that 1,3-oxazol-5(4H)-ones with an OH group in the side chain at C(4), i.e., 8, R1 = HO~~, under acidic conditions (HCl, toluene, 110 °C) form 2-acylaminolactones via the nucleophilic addition to the C(5)=O group of the azlactone, followed by ring opening31,46 (8 → 10, Scheme 3).
In the present paper, the results of lactam formations of the type 8 → 10 (HNu = NH2) under acidic conditions (‘direct amide cyclization’) are reported.
RESULTS AND DISCUSSION
We intended to prepare 1,3-oxazol-5(4H)-ones of type (13) with an NH2 group in the side chain as starting materials for the cyclization of type 8 → 10 via the alkylation of the anion of 4-benzyl-2-phenyl-1,3-oxazol-5(4H)-one (11)47,48 (Scheme 4). Whereas benzylations and allylations of azlactones are known to proceed smoothly by using Hünig’s base,41,49,50 alkylations occur more sluggishly. These reactions could be improved by variation of the reaction conditions, e.g., the base and the solvent.38,45,46,51,52 For this reason, alkyl iodides are the most promising reagents. The required alkyl iodides (12) with N3 as a masked amino group were prepared in a straightforward sequence starting with ω-bromoalkanols, which were transformed into the corresponding ω-azidoalkanols, respectively. These products were treated with 2-fluoro-N-methylpyridinium tosylate (FMPT) and NaI53 to give the desired ω-azidoalkyliodides (12a–c) in good yields (see experimental part).
The alkylations of 11 were carried out in a mixture of THF/HMPT by deprotonation with LDA at ca. –78 °C and reaction with 12a–c at ca. 15 °C. In all cases, mixtures of the 4-alkylated oxazolones (13) and the O-alkylated oxazoles (14) were obtained with 13 as the major product (Table 1). All attempts to separate the two products were in vain.
Furthermore, preliminary experiments (H2, Pd/C; LiAlH4; ZnCl2 or SnCl2 in MeOH) showed that it was not possible to reduce the azido group of 13 without destroying the azlactone structure. Therefore, the mixtures 13/14 were treated with dimethylamine in acetonitrile to yield the azido amides (15), which subsequently were reduced in a Staudinger reaction54–56 with triethylphosphine57 and water to give the corresponding amines (16) in almost quantitative yields.58
The ω-aminoalkyl-substituted diamides (16) were exposed to the conditions of the ‘direct amide cyclization’,46 i.e., dry HCl gas was bubbled through a solution of 16 in boiling dry toluene for 1.5–2 h, and the mixture was kept boiling for 15 h. Then, toluene and excess HCl were evaporated and the residue was separated by ‘flash column chromatography’59 (SiO2, Et2O/hexane) to give the lactams 18a–c in 27, 23, and 11% yield, respectively (Scheme 5). In an analogous experiment with 16c, in which toluene had not been dried before its use, the corresponding carboxylic acid (19c) was obtained in 51% yield, indicating the intermediate formation of an oxazolonium ion of type (17).
The spectroscopic data (MS, NMR, IR) of 18a–c were in accordance with the lactam structures. Indicative were two IR absorptions at 1675–1670 and 1650–1645 cm–1; the amide bands in the precursors (16) appeared at 1660 and 1625 cm–1. The 1H-NMR spectra showed the lactam NH (CONH–CH2) at 5.80–5.30 ppm as a multiplet or broadened signal, whereas the NH of the benzamide group absorbed as a singlet at 7.64–7.61 ppm. The corresponding absorptions in 16 were at ca. 1.95 (NH2) and 7.99 ppm (PhCONH). On the other hand, the differences of the 13C-NMR absorptions for the amide and lactam C=O groups of 18 were not significant.
For this reason, the structures of 18a and 18b were established by X-ray crystal-structure determinations (Figure 1). Suitable crystals were grown from Et2O/hexane/CH2Cl2 and CH2Cl2/hexane, respectively, whereas no good crystals of 18c could be obtained.
Since the space groups of 18a and 18b are centrosymmetric, the compounds in the crystal are racemic. The macrocyclic ring of 18a is severely disordered. The disorder is of the type common to such rings where the molecule can adopt a large number of slightly different conformations. The net effect is that the electron density of each atom is spread out over a wide region and it is very difficult to define a model, which closely fits the electron density. Two sets of positions were defined for seven consecutive CH2 groups. Furthermore, the crystals were very weakly diffracting, and only a small percentage of the reflections in the region with 2θ > 40° had a measurable intensity. It was also necessary to record the data at room temperature, because the crystals were always damaged when the temperature was reduced. These factors, together with the disorder, have resulted in a poor agreement between the model and the observed data. This is reflected in the high R-factor and large estimated standard deviations for the atomic parameters. The model is the best one obtainable under the circumstances, but the nature of the disorder makes it extremely difficult to adequately account for the electron density distribution in the disordered region of the molecule. The refined model is therefore only an approximation to the large number of actual molecular conformations adopted in the crystal lattice. In the case of 18b, the macrocyclic ring is disordered over two conformations. Two sets of positions were defined for five consecutive CH2 groups.
The N-H group of the 15- and 14-membered rings (in 18a and 18b, resp.) acts as a donor for intermolecular hydrogen bonds with the corresponding acceptor atom being the carbonyl O-atom of the side chain of a neighboring molecule. These interactions link the molecules into extended chains which run parallel to the [010] direction and can be described by a graph set motif61 of C(7). The NH of the benzamide group is not involved in any intermolecular hydrogen bonds.
A possible explanation for the low yields of the cyclization reaction in comparison with analogous lactone formations46 is the unfavorable strongly acidic reaction condition, which led to the preferred protonation of the amino group, and not only to that of the amide group in 16 or the intermediate oxazolone to give the activated 17.62 Therefore, we tried to substitute the Brønsted acid by a Lewis acid, e.g., boron trifluoride (BF3).
Treatment of a solution of 16a and 16c in toluene with BF3.Et2O at 100 °C and chromatographic workup led to the corresponding lactams (18a) and (18c), respectively, in only 7–8% yield. In addition, a labile product, for which we propose the structure 20a,c, was obtained in 10 and 23% yield, respectively (Scheme 6). In the case of 20c, it was possible to collect the spectroscopic data (see experimental part), which supported the structure, but the compound was not stable at room temperature and decomposed slowly to give at least three different products. The isolated homologous product (20a) rearranged within a few minutes into the azlactone (17a), which had also been isolated in 30% yield from the original reaction mixture. Furthermore, the linear ω-benzamido α-amino acid (21a) was obtained in 35% yield.
In an additional experiment, a solution of 16a and BF3.Et2O in toluene was heated to reflux for 2 h. Then, 6 equivalents of methanol were added and the mixture was kept boiling for another 21 h. Chromatographic workup gave the ester (22a) in 80% yield, and a second product in ca. 6% yield, which rearranged quickly to give 22a.
A likely reaction mechanism for the formation of 21a and 22a is shown in Scheme 6. Whereas, in the intermediate azlactones (17), the nucleophilic addition of the NH2 group onto the C(5)=O group leads to the lactams (18), the alternative addition onto C(2)42,43 yields the bicyclic product (20). Hydrolysis and methanolysis of 20a then give the linear α-amino-ω-benzamido alkanoic acid (21) and ester (22), respectively.
CONCLUSIONS
The α,α-disubstituted α-acylamino acid amides of type (16), which bear an amino group in the ω-position, are conveniently accessible via alkylation of 4-benzyl-2-phenyl-1,3-oxazol-5(4H)-one (11) with ω-azidoalkyl iodides (12), ring opening with dimethylamine, and reduction of the azido group. Treatment of 16 with dry HCl gas in boiling toluene leads to the corresponding α-benzamido lactams (18), albeit in low yield. The analogous BF3-catalyzed cyclization yields a mixture of different products with the lactams (18) in very low yield. We propose that the cyclization reactions occur via the intermediate formation of 1,3-oxazol-5(4H)-ones (azlactones, 17), i.e., the so-called ‘direct amide cyclization’. An indication for the appearance of azlactones as intermediates is the formation of the products (19) – (22), and in the BF3-catalyzed reaction with 16a, the corresponding azlactone 17a could be isolated.
EXPERIMENTAL
General remarks. TLC: silica gel 60 F254 aluminium sheets (0.25 mm, Merck). Flash column chromatography59 (FCC): silica gel 60 (40–60 μm, Merck). Melting points: Mettler FP-5 apparatus, uncorrected. IR spectra: Perkin-Elmer 297 or Perkin-Elmer 781 IR spectrophotometer, in CHCl3 or KBr; in cm–1. 1H-NMR (300 MHz) and 13C-NMR (50 MHz) spectra: Varian XL-200, Bruker AC-300, and Bruker ARX-300 instrument, in DMSO-d6 or CDCl3; chemical shifts in ppm, coupling constants J in Hz; multiplicity of C atoms from DEPT spectra. EI-MS: MAT-112 instrument, 70 eV; CI-MS: MAT-90 instrument, isobutane or NH3 as carrier gas; ESI-MS: Finnigan TSQ-700 instrument.
Starting materials. 4-Benzyl-2-phenyl-1,3-oxazol-5(4H)-one (11) was prepared from 2-benzamido-3-phenylpropanoic acid according to ref.47 All other reagents and solvents were commercially available (Fluka, Aldrich).
Synthesis of ω-azidoalkan-1-ols. General procedure. A solution of a ω-bromoalkan-1-ol (1 equiv.), NaN3 (2 equiv.), and Bu4NHSO4 (0.1 equiv.) in THF/H2O (2:1) was stirred for 60 h at 25–34 °C. Then, the mixture was poured into H2O and extracted with Et2O (3x). The organic phase was dried over MgSO4, filtered, and evaporated. The crude products were directly used for the next reaction.
12-Azidododecan-1-ol. From 12-bromododecan-1-ol (3.03 g, 11.4 mmol) in THF (13 mL) and H2O (7 mL), NaN3 (1.49 g, 22.9 mmol), and Bu4NHSO4 (0.78 g, 2.3 mmol). Yield: 2.52 g (97%). Colorless oil. IR (CHCl3): 3620w, 3450w (br), 3000w, 2930s, 2860s, 2100s, 1470m, 1460m, 1390w, 1350w, 1290m, 1255m (br), 1050m (br), 1015w, 890w (br). 1H-NMR (CDCl3): 3.64 (t-like, J ≈ 5.2, CH2O); 3.25 (t, J = 6.9, CH2N3); 1.65–1.50 (m, 3 CH2); 1.45–1.25 (m, 7 CH2). 13C-NMR (CDCl3): 62.8 (t, CH2O); 51.4 (t, CH2N3); 32.7, 29.5 (2t, 2 CH2); 29.4, 29.3 (2t, 4 CH2); 29.0, 28.7, 26.6, 25.6 (4t, 4 CH2). CI-MS (NH3): 245 (28, [M+18]+), 228 (7, [M+1]+), 200 (100, [M+1–N2]+).
11-Azidoundecan-1-ol. From 11-bromoundecan-1-ol (3.98 g, 15.8 mmol) in THF (20 mL) and H2O (10 mL), NaN3 (1.23 g, 19.0 mmol), and Bu4NHSO4 (0.97 g, 2.9 mmol). Yield: 3.37 g (quant.). Colorless oil. IR (CHCl3): 3620m, 3460w (br), 3030w, 3010m, 2930s, 2860s, 2100s, 1465m, 1455m, 1390w, 1350m, 1285m, 1250m (br), 1110w, 1050m (br), 1025m, 890w (br). 1H-NMR (CDCl3): 3.65 (t, J = 6.6, CH2O); 3.26 (t, J = 6.9, CH2N3); 1.65–1.50 (m, 2 CH2); 1.40–1.25 (m, 7 CH2). 13C-NMR (CDCl3): 62.5 (t, CH2O); 51.3 (t, CH2N3); 32.5, 29.4 (2t, 2 CH2); 29.2 (t, 3 CH2); 28.9, 28.6, 26.5, 25.6 (4t, 4 CH2). CI-MS (NH3): 214 (6, [M+1]+), 186 (100, [M+1–N2]+). Anal. Calcd for C11H23N3O: C, 61.93; H, 10.87; N, 19.70. Found: C, 61.69; H, 10.66; N, 19.86.
10-Azidodecan-1-ol. From 10-bromodecan-1-ol (1.90 g, 8.0 mmol) in THF (10 mL) and H2O (5 mL), NaN3 (0.63 g, 9.6 mmol), and Bu4NHSO4 (0.49 g, 1.4 mmol). Yield: 1.58 g (99%). Colorless oil. IR (CHCl3): 3620w, 3440w (br), 3030w, 2995w, 2920s, 2850s, 2095s, 1465s, 1455w, 1390w, 1350w, 1300m, 1255m (br), 1045m (br), 1015w, 915w. 1H-NMR (CDCl3): 3.65 (t, J = 6.6, CH2O); 3.26 (t, J = 6.9, CH2N3); 1.70–1.50 (m, 2 CH2); 1.50–1.20 (m, 6 CH2). 13C-NMR (CDCl3): 62.8 (t, CH2O); 51.4 (t, CH2N3); 32.6, 29.4 (2t, 2 CH2); 29.3 (t, 2 CH2); 29.0, 28.7, 26.6, 25.6 (4t, 4 CH2). CI-MS (NH3): 217 (100, [M+18]+), 200 (9, [M+1]+), 172 (48, [M+1–N2]+). Anal. Calcd for C10H21N3O: C, 60.27; H, 10.62; N, 21.08. Found: C, 60.37; H, 10.45; N, 20.98.
Synthesis of ω-azidoalkyl iodides (12). General procedure. To a solution of an ω-azidoalkan-1-ol (1 equiv.) and triethylamine (TEA, 1.5 equiv.) in CHCl3 was added 2-fluoro-N-methylpyridinium tosylate (FMPT, 1.5–2.0 equiv.) and the mixture stirred for 2 h at 24 °C. After evaporation of the solvent, the residue was dissolved in acetone, NaI (2 equiv.) was added, and the mixture was heated to reflux for 18 h. The precipitate was filtered, the filtrate evaporated, and Et2O added. Filtration, evaporation, and FCC (SiO2, Et2O/hexane 1:9 to 2:1) gave the product and up to 50% recovered starting material.
12-Azidododecyl iodide (12a). From 12-azidododecan-1-ol (2.30 g, 10.1 mmol) in CHCl3 (55 mL), FMPT (4.29 g, 15.1 mmol), and TEA (1.54 g, 15.2 mmol), and then NaI (3.03 g, 20.2 mmol) in acetone (55 mL). Yield: 1.68 g (49%) of 12a. Colorless oil. IR (CHCl3): 3000w, 2930s, 2860s, 2100s, 1465w, 1460w, 1370w, 1350w, 1290w, 1255w (br), 1170w (br). 1H-NMR (CDCl3): 3.19 (t, J = 6.9, CH2N3); 3.12 (t, J = 7.1, CH2I); 1.75 (p, J ≈ 7.1, CH2); 1.65–1.45 (m, CH2); 1.40–1.15 (m, 8 CH2). 13C-NMR (CDCl3): 51.4 (t, CH2N3); 33.5, 30.4 (2t, 2 CH2); 29.4 (t, 3 CH2); 29.3, 29.1, 28.8, 28.4, 26.6 (5t, 5 CH2); 7.1 (t, CH2I). CI-MS (NH3): 310 (14, [M+1–N2]+), 182 (19), 151 (100). Anal. Calcd for C12H24IN3: C, 42.74; H, 7.17; I, 37.63; N, 12.46. Found: C, 42.53; H, 7.02; I, 37.41, N, 12.38.
11-Azidoundecyl iodide (12b). From 11-azidoundecan-1-ol (2.21 g, 10.4 mmol) in CHCl3 (55 mL), FMPT (4.29 g, 15.1 mmol), and TEA (1.60 g, 15.8 mmol), and then NaI (3.03 g, 20.2 mmol) in acetone (50 mL). Yield: 1.78 g (53%) of 12b. Colorless oil. IR (CHCl3): 3000w, 2930s, 2860m, 2100s, 1465w, 1455w, 1370w, 1350w, 1255w (br), 1170w. 1H-NMR (CDCl3): 3.26 (t, J = 7.0, CH2N3); 3.19 (t, J = 7.0, CH2I); 1.82 (p, J ≈ 7.2, CH2); 1.60 (p, J ≈ 7.3, CH2); 1.45–1.25 (m, 7 CH2). 13C-NMR (CDCl3): 51.4 (t, CH2N3); 33.5, 30.4 (2t, 2 CH2); 29.30 (t, 2 CH2); 29.27, 29.0, 28.8, 28.4, 26.6 (5t, 5 CH2); 7.1 (t, CH2I). CI-MS (NH3): 324 (14, [M+1]+), 296 (100, [M+1–N2]+). Anal. Calcd for C11H22IN3: C, 40.88; H, 6.86; I, 13.00; N, 39.26. Found: C, 40.86; H, 6.61; I, 12.82; N, 39.05.
10-Azidodecyl iodide (12c). From 10-azidodecan-1-ol (1.45 g, 7.3 mmol) in CHCl3 (35 mL), FMPT (4.22 g, 14.6 mmol), and TEA (1.52 g, 10.9 mmol), and then NaI (2.18 g, 14.6 mmol) in acetone (50 mL). Yield: 1.11 g (49%). Colorless oil. IR (CHCl3): 3000m, 2930s, 2860s, 2100s, 1465m, 1455m, 1430w, 1370w, 1350m, 1295m, 1285m, 1260m (br), 1175m, 1130w, 1100w, 890w. 1H-NMR (CDCl3): 3.26 (t, J = 6.9, CH2N3); 3.19 (t, J = 7.0, CH2I); 1.90–1.75 (m, CH2); 1.70–1.55 (m, CH2); 1.40–1.25 (m, 6 CH2). 13C-NMR (CDCl3): 62.8 (t, CH2O); 51.4 (t, CH2N3); 33.5, 30.4, 29.3, 29.2, 28.4, 27.8, 26.6 (8t, 8 CH2); 7.1 (t, CH2I). CI-MS (NH3): 327 (12, [M+18]+), 282 (20, [M+1–N2]+), 154 (100). Anal. Calcd for C10H20IN3: C, 38.85; H, 6.52; I, 41.04; N, 13.59. Found: C, 39.08; H, 6.37; I, 40.71; N, 13.35.
Alkylations of 1,3-oxazol-5(4H)-one (11) with ω-azidoalkyl iodides (12). General procedure. To a solution of LDA in a mixture of THF and HMPT between –110 and –60 °C was added a solution of 11 in THF. After addition of 12, the temperature was increased to 15–20 °C and the mixture stirred for 5–16 h. Then, the mixture was extracted with cold water (3x) and Et2O, and the organic phase was dried over MgSO4. Filtration, evaporation, and FCC (SiO2, Et2O/hexane) gave mixtures of 13 and 14.
4-(12-Azidododecyl)-4-benzyl-2-phenyl-1,3-oxazol-5(4H)-one (13a) and 5-[(12-Azidododecyl)oxy]- 4-benzyl-2-phenyl-1,3-oxazole (14a). From 11 (1.00 g, 3.99 mmol) in THF (7 mL), LDA (4.4 mmol) in THF (7 mL) and HMPT (4 mL), and 12a (1.50 g, 4.40 mmol) in THF (7 mL). FCC gave 0.44 g (24%) of 13a and 0.57 g (26%) of a ca. 6:11 mixture of 13a and 14a as colorless oils. Data of 13a: IR (CHCl3): 3060w, 3030w, 3010w, 2930s, 2860m, 2100s, 1815s, 1655s, 1605w, 1580w, 1495w, 1465w, 1450m, 1320m, 1290m, 1050m, 970m, 700s. 1H-NMR (CDCl3): 7.90–7.80 (m, 2 arom. H); 7.55–7.50 (m, 1 arom. H); 7.45–7.40 (m, 2 arom. H); 7.20–7.10 (m, 5 arom. H); 3.30–3.15 (m, CH2N3); 3.22, 3.14 (AB, J = 13.4, PhCH2); 2.05–1.95 (m, CH2); 1.65–1.50 (m, 2 CH2); 1.40–1.10 (m, 8 CH2). 13C-NMR (CDCl3): 179.6 (s, CO); 159.6 (s, CN); 134.3 (s, 1 arom. C); 132.3 (d, 1 arom. CH); 130.0, 128.5, 127.9, 127.6 (4d, 2 arom. CH each); 127.0 (d, 1 arom. CH); 125.7 (s, 1 arom. C); 74.7 (s, C(4)); 51.3 (t, CH2N3); 43.7 (t, PhCH2); 37.3 (t, CH2); 29.3 (t, 5 CH2); 29.1, 29.0, 28.7, 26.6, 23.9 (5t, 5 CH2). CI-MS (NH3): 461 (100, [M+1]+), 433 (26, [M+1–N2]+).
Data of 14a (from the mixture): 1H-NMR (CDCl3): 7.95–7.85 (m, 2 arom. H); 7.30–7.25 (m, 8 arom. H); 4.11 (t, J = 6.6, CH2O); 3.83 (s, PhCH2); 1.80–1.65 (m, CH2).
4-(11-Azidoundecyl)-4-benzyl-2-phenyl-1,3-oxazol-5(4H)-one (13b) and 5-[(11-Azidoundecyl)oxy]- 4-benzyl-2-phenyl-1,3-oxazole (14b). From 11 (0.99 g, 3.94 mmol) in THF (7 mL), LDA (4.1 mmol) in THF (7 mL) and HMPT (4 mL), and 12b (1.40 g, 4.33 mmol) in THF (7 mL). Yield: 1.14 g (65%) of an oily 19:1 mixture of 13b and 14b. Data of this mixture: IR (CHCl3): 3060w, 3030w, 3010w, 2930s, 2860s, 2100s, 1815s, 1655s, 1600w, 1580w, 1495m, 1465w, 1450m, 1320m, 1290s, 1255m, 1045m, 1025m, 970m, 895m, 700s. EI-MS: 446 (4, M+.), 418 (5), 355 (12), 285 (100), 251 (32). Anal. Calcd for C27H34N4O: C, 72.62; H, 7.67; N, 12.55. Found: C, 72.40; H, 7.81; N, 12.81. Data of 13b (from the mixture): 1H-NMR (CDCl3): 7.90–7.80 (m, 2 arom. H); 7.60–7.50 (m, 1 arom. H); 7.45–7.35 (m, 2 arom. H); 7.20–7.10 (m, 5 arom. H); 3.24 (t, J = 7.0, CH2N3); 3.22, 3.14 (AB, J = 13.3, PhCH2); 2.05–1.95 (m, CH2); 1.65–1.50 (m, CH2); 1.45–1.10 (m, 8 CH2). 13C-NMR (CDCl3): 179.6 (s, CO); 159.6 (s, CN); 134.3 (s, 1 arom. C); 132.3 (d, 1 arom. CH); 130.0, 128.5, 127.9, 127.6 (4d, 2 arom. CH each); 127.0 (d, 1 arom. CH); 125.7 (s, 1 arom. C); 74.7 (s, C(4)); 51.3 (t, CH2N3); 43.7 (t, PhCH2); 37.3 (t, CH2); 29.2 (t, 4 CH2); 29.1, 29.0, 28.7, 26.6, 23.9 (5t, 5 CH2).
Data of 14b (from the mixture): 1H-NMR (CDCl3): 7.95–7.90 (m, 2 arom. H); 7.30–7.25 (m, 8 arom. H); 4.11 (t, J = 6.6, CH2O); 3.84 (s, PhCH2); 2.75–2.65 (m, CH2); 2.50–2.35 (m, CH2). 13C-NMR (CDCl3): 128.0, 127.7, 127.0 (3d, 2 arom. CH each); 38.4 (t, CH2).
4-(10-Azidodecyl)-4-benzyl-2-phenyl-1,3-oxazol-5(4H)-one (13c) and 5-[(10-Azidodecyl)oxy]- 4-benzyl-2-phenyl-1,3-oxazole (14c). From 11 (1.00 g, 3.98 mmol) in THF (8 mL) and HMPT (4 mL), with LDA (4.3 mmol), and 12c (1.40 g, 4.53 mmol) in THF (7 mL). Yield: 1.06 g (61%) of an oily 10:1 mixture of 13c and 14c. Data of this mixture: IR (CHCl3): 3060w, 3030w, 3000w, 2990w, 2930s, 2860m, 2100s, 1815s, 1655s, 1600w, 1580w, 1500w, 1470w, 1450m, 1440w, 1320m, 1290m, 1250m, 1045m, 1025m, 980m, 895w, 700s. CI-MS (NH3): 433 (100, [M+1]+), 405 (7), 250 (15). Anal. Calcd for C26H32N4O: C, 72.19; H, 7.46; N, 12.95. Found: C, 72.38; H, 7.32; N, 12.95. Data of 13c (from the mixture): 1H-NMR (CDCl3): 7.90–7.80 (m, 2 arom. H); 7.55–7.45 (m, 1 arom. H); 7.45–7.30 (m, 2 arom. H); 7.15–7.10 (m, 5 arom. H); 3.24 (t, J = 6.9, CH2N3); 3.21, 3.14 (AB, J = 13.5, PhCH2); 2.05–1.90 (m, CH2); 1.60–1.50 (m, CH2); 1.25–1.20 (m, 7 CH2). 13C-NMR (CDCl3): 179.5 (s, CO); 159.6 (s, CN); 134.3 (s, 1 arom. C); 132.2 (d, 1 arom. CH); 129.9, 128.5, 127.9, 127.5 (4d, 2 arom. CH each); 126.9 (d, 1 arom. CH); 125.6 (s, 1 arom. C); 74.6 (s, C(4)); 51.2 (t, CH2N3); 43.6 (t, PhCH2); 37.2 (t, CH2); 29.2 (t, CH2); 29.1 (t, 2 CH2), 29.0, 28.8, 28.6, 26.5, 23.8 (5t, 5 CH2).
Data of 14c (from the mixture): 1H-NMR (CDCl3): 7.95–7.90 (m, 2 arom. H); 7.45–7.35 (m, 3 arom. H); 7.35–7.30 (m, 3 arom. H); 7.30–7.05 (m, 2 arom. H); 4.11 (t, J = 6.6, CH2O); 3.84 (s, PhCH2); 2.45–2.35 (m, CH2); 1.75–1.60 (m, 1 H); 1.60–1.45 (m, CH2); 1.45–1.05 (m, 11 H). 13C-NMR (CDCl3): 128.2, 128.1, 127.7, 125.2 (4d, 2 arom. CH each); 74.5 (t, CH2).
Synthesis of N-[ω-azido-1-benzyl-1-(N,N-dimethylcarbamoyl)alkyl]benzamides (15). General procedure. To a solution of the mixture of 13 and 14 in acetonitrile was added dropwise condensed dimethylamine and the mixture stirred at rt for 2–5 h. The product was purified by FCC (Et2O/hexane 3:2).
N-[13-Azido-1-benzyl-1-(N,N-dimethylcarbamoyl)tridecyl]benzamide (15a). From 13a/14a (0.50 g, 1.1 mmol) in acetonitrile (5 mL). FCC gave 0.45 g (96%) of 15a as a colorless thick oil. IR (CHCl3): 3360w, 3060w, 3030w, 3000m, 2930s, 2860m, 2100s, 1655s, 1625s, 1605m, 1580w, 1505s, 1480s, 1460m, 1455m, 1400m, 1350w, 1255m, 1150w, 1105w, 1080w, 1055w, 1030w, 880w, 700m. 1H-NMR (CDCl3): 8.00 (br. s, NH); 7.75–7.65 (m, 2 arom. H); 7.50–7.45 (m, 1 arom. H); 7.45–7.35 (m, 2 arom. H); 7.20–7.15 (m, 3 arom. H); 7.05–6.95 (m, 2 arom. H); 4.13, 3.22 (AB, J = 14.1, PhCH2); 3.50–2.90 (br. s, Me2N); 3.25 (t, J = 7.0, CH2N3); 3.15–2.90 (m, 1 H); 2.25–1.90 (m, 1 H); 1.65–1.50 (m, 2 CH2); 1.45–1.20 (m, 8 CH2). 13C-NMR (CDCl3): 171.1, 165.7 (2s, 2 CO); 136.5, 135.7 (2s, 2 arom. C); 131.0 (d, 1 arom. CH); 129.4, 129.0, 128.3, 126.7 (4d, 2 arom. CH each); 126.6 (d, 1 arom. CH); 66.2 (s, Cq); 51.3 (t, CH2N3); 38.8 (t, PhCH2); 38.5 (q, Me2N); 33.7 (t, CH2); 29.4 (t, 6 CH2); 29.0, 28.7, 26.6, 24.3 (4t, 4 CH2). CI-MS (NH3): 506 (100, [M+1]+), 478 (85, [M+1–N2]+), 461 (70). Anal. Calcd for C30H43N5O2: C, 71.25; H, 8.57; N, 13.85. Found: C, 71.08; H, 8.31; N, 13.68.
N-[12-Azido-1-benzyl-1-(N,N-dimethylcarbamoyl)dodecyl]benzamide (15b). From 13b/14b (0.20 g, 0.44 mmol) in acetonitrile (2 mL). FCC gave 0.19 g (94%) of 15b as a colorless thick oil. IR (CHCl3): 3660w, 3240w, 3100w, 3080w, 3060w, 3020w, 3000w, 2930s, 2860s, 2100s, 1655m, 1625s, 1605w, 1580w, 1505s, 1480s, 1470m, 1455m, 1395m, 1350w, 1300w, 1255m, 1210w, 1150w, 1110w, 1080w, 1055w, 1030w, 1000w, 880w, 700m. 1H-NMR (CDCl3): 8.01 (br. s, NH); 7.75–7.70 (m, 2 arom. H); 7.50–7.45 (m, 1 arom. H); 7.45–7.35 (m, 2 arom. H); 7.20–7.15 (m, 3 arom. H); 7.05–7.00 (m, 2 arom. H); 4.14, 3.23 (AB, J = 14.2, PhCH2); 3.60–2.80 (br. s, Me2N); 3.25 (t, J = 6.9, CH2N3); 3.10–2.95 (m, 1 H); 2.05–1.90 (m, 1 H); 1.50–1.20 (m, 9 CH2). 13C-NMR (CDCl3): 171.1, 165.6 (2s, 2 CO); 136.5, 135.7 (2s, 2 arom. C); 130.9 (d, 1 arom. CH); 129.4, 128.3, 127.9, 126.7 (4d, 2 arom. CH each); 126.6 (d, 1 arom. CH); 66.1 (s, Cq); 51.3 (t, CH2N3); 38.7 (t, PhCH2); 38.4 (q, Me2N); 33.6, 29.3 (2t, 2 CH2); 29.2 (t, 4 CH2); 28.9, 28.6, 26.5, 24.2 (4t, 4 CH2). CI-MS (NH3): 492 (8, [M+1]+), 464 (8, [M+1–N2]+), 447 (100), 419 (5). Anal. Calcd for C29H41N5O2: C, 70.84; H, 8.41; N, 14.24. Found: C, 71.01; H, 8.20; N, 14.43.
N-[11-Azido-1-benzyl-1-(N,N-dimethylcarbamoyl)undecyl]benzamide (15c). From 13c/14c (0.84 g, 1.94 mmol) in acetonitrile (10 mL). FCC gave 0.76 g (91%) of 15c as a colorless thick oil. IR (CHCl3): 3660w, 3355m, 3060w, 3030w, 3000m, 2930s, 2860m, 2100s, 1655s, 1625s, 1605m, 1580m, 1505s, 1480s, 1455m, 1400s, 1350w, 1300w, 1255m, 1180w, 1115m, 1080w, 1055w, 1030w, 1000w, 880w, 700m. 1H-NMR (CDCl3): 8.00 (br. s, NH); 7.75–7.70 (m, 2 arom. H); 7.50–7.40 (m, 1 arom. H); 7.40–7.30 (m, 2 arom. H); 7.20–7.10 (m, 3 arom. H); 7.00–6.90 (m, 2 arom. H); 4.12, 3.22 (AB, J = 14.0, PhCH2); 3.50–2.85 (br. s, Me2N); 3.24 (t, J = 7.0, CH2N3); 3.10–2.90 (m, 1 H); 2.00–1.85 (m, 1 H); 1.60–1.50 (m, CH2); 1.50–1.20 (m, 7 CH2). 13C-NMR (CDCl3): 170.9, 165.6 (2s, 2 CO); 136.4, 135.5 (2s, 2 arom. C); 130.9 (d, 1 arom. CH); 129.4, 128.2, 127.8, 126.6 (4d, 2 arom. CH each); 126.4 (d, 1 arom. CH); 65.8 (s, Cq); 51.1 (t, CH2N3); 38.5 (t, PhCH2); 38.3 (q, Me2N); 33.4, 29.2 (2t, 2 CH2); 29.1 (t, 3 CH2); 28.8, 28.5, 26.4, 24.1 (4t, 4 CH2). CI-MS (NH3): 450 (8, [M+1–N2]+), 433 (100), 405 (5). Anal. Calcd for C28H39N5O2: C, 70.41; H, 8.23; N, 14.66. Found: C, 70.63; H, 8.20; N, 14.44.
Synthesis of N-[ω-amino-1-benzyl-1-(N,N-dimethylcarbamoyl)alkyl]benzamides (16). General procedure. To a solution of 15 (1 equiv.) in THF under N2 atmosphere at rt was added Et3P (1.4 equiv.) and the mixture stirred at rt for 2.5 h. The spontaneous elimination of N2 ceased after ca. 20 min. After addition of water (2.8 equiv.) and stirring for 2 h, the mixture was poured into CHCl3/MeOH/NH3 (85:14:1), filtered through SiO2, and evaporated. The product contained traces of Et3PO, which could not be removed.
N-[13-Amino-1-benzyl-1-(N,N-dimethylcarbamoyl)tridecyl]benzamide (16a). From 15a (0.35 g, 0.69 mmol), Et3P (0.14 mL, 0.97 mmol), and H2O (0.035 g, 1.94 mmol). Yield: 0.33 g (95%) of 16a as a colorless thick oil. IR (CHCl3): 3620w (br), 3360w (br), 3060w, 3030w, 3000m, 2930s, 2860m, 1660m, 1625s, 1605m, 1580w, 1505s, 1480s, 1455m, 1400m, 1250w, 1220w, 1180w, 1150w, 1110m, 1080w, 1050w, 1030w, 880w, 700m. 1H-NMR (CDCl3): 7.99 (br. s, NH); 7.75–7.65 (m, 2 arom. H); 7.50–7.45 (m, 1 arom. H); 7.45–7.35 (m, 2 arom. H); 7.20–7.15 (m, 3 arom. H); 7.05–6.95 (m, 2 arom. H); 4.12, 3.22 (AB, J = 14.2, PhCH2); 3.60–2.80 (br. s, Me2N); 3.05–2.95 (m, 1 H); 2.67 (t, J = 7.0, CH2N); 2.00–1.90 (m, 1 H); 1.35–1.15 (m, 10 CH2). 13C-NMR (CDCl3): 170.8, 165.6 (2s, 2 CO); 136.3, 135.2 (2s, 2 arom. C); 130.8 (d, 1 arom. CH); 129.4, 128.1, 127.7, 126.5 (4d, 2 arom. CH each); 126.3 (d, 1 arom. CH); 65.4 (s, Cq); 41.1 (t, CH2N); 38.2 (t, PhCH2); 38.1 (q, Me2N); 33.2 (t, CH2); 29.1 (t, 5 CH2); 29.0 (t, 2 CH2); 26.4, 23.9, 18.6 (3t, 3 CH2). ESI-MS (MeOH): 480 (100, [M+1]+).
N-[12-Amino-1-benzyl-1-(N,N-dimethylcarbamoyl)dodecyl]benzamide (16b). From 15b (0.24 g, 0.49 mmol), Et3P (0.11 mL, 0.75 mmol), and H2O (0.025 g, 1.40 mmol). Yield: 0.23 g (quant.) of 16b as a colorless thick oil. IR (CHCl3): 3360m (br), 3070w, 3030w, 3000m, 2930s, 2860m, 1660s, 1625s, 1605m, 1580m, 1505s, 1480s, 1455m, 1400m, 1255w, 1180w, 1150w, 1110w, 1080w, 1050w, 1030w, 880w, 705m. 1H-NMR (CDCl3): 7.99 (br. s, NH); 7.75–7.70 (m, 2 arom. H); 7.50–7.35 (m, 2 arom. H); 7.20–7.15 (m, 3 arom. H); 7.05–6.95 (m, 3 arom. H); 4.12, 3.22 (AB, J = 14.1, PhCH2); 3.19 (br. s, Me2N); 3.05–2.90 (m, 1 H); 2.70 (t, J = 6.4, CH2N); 2.05–1.80 (m, 1 H); 1.95 (br. s, NH2); 1.50–1.40 (m, CH2); 1.40–1.00 (m, 8 CH2). 13C-NMR (CDCl3): 171.1, 165.7 (2s, 2 CO); 136.5, 135.6 (2s, 2 arom. C); 131.0 (d, 1 arom. CH); 129.5, 128.3, 128.0, 126.7 (4d, 2 arom. CH each); 126.6 (d, 1 arom. CH); 66.1 (s, Cq); 41.7 (t, CH2N); 38.7 (t, PhCH2); 38.5 (q, Me2N); 33.7, 32.7 (2t, 2 CH2); 29.4 (t, 2 CH2); 29.34 (t, 2 CH2); 29.30 (t, 2 CH2); 26.7, 24.2 (2t, 2 CH2). CI-MS (i-butane): 466 (100, [M+1]+).
N-[11-Amino-1-benzyl-1-(N,N-dimethylcarbamoyl)undecyl]benzamide (16c). From 15c (0.57 g, 1.19 mmol), Et3P (0.27 mL, 1.83 mmol), and H2O (0.13 g, 7.22 mmol). Yield: 0.52 g (97%) of 16c as a colorless thick oil. IR (CHCl3): 3360m, 3060w, 3000m, 2930s, 2860m, 1660s, 1625s, 1610m, 1580m, 1510s, 1480s, 1460m, 1400m, 1255w, 1215w, 1150m, 1110m, 1080w, 1055w, 1030w, 880w, 700m. 1H-NMR (CDCl3): 7.99 (br. s, NH); 7.70–7.65 (m, 2 arom. H); 7.50–7.45 (m, 1 arom. H); 7.45–7.35 (m, 2 arom. H); 7.20–7.15 (m, 3 arom. H); 7.05–6.95 (m, 2 arom. H); 4.12, 3.22 (AB, J = 14.2, PhCH2); 3.19 (br. s, Me2N); 3.10–2.95 (m, 1 H); 2.65 (t, J = 6.9, CH2N); 2.05–1.90 (m, 1 H); 1.60–1.10 (m, 8 CH2). 13C-NMR (CDCl3): 171.0, 165.6 (2s, 2 CO); 136.5, 135.6 (2s, 2 arom. C); 130.9 (d, 1 arom. CH); 129.4, 128.3, 127.9, 126.6 (4d, 2 arom. CH each); 126.5 (d, 1 arom. CH); 66.1 (s, Cq); 42.0 (t, CH2N); 38.7 (t, PhCH2); 38.4 (q, Me2N); 33.6 (t, CH2); 29.3 (t, 3 CH2); 29.2 (t, 3 CH2); 26.7, 24.2 (2t, 2 CH2). CI-MS (NH3): 452 (100, [M+1]+).
HCl catalyzed cyclization reactions with 16. General procedure. Through a 1.9 mM solution of 16 in boiling toluene, dry HCl gas was bubbled for 1.5–2 h, and the boiling mixture stirred for 15 h. Evaporation of the solvent and FCC (Et2O/hexane 1:1) gave the lactam (18).
N-(3-Benzyl-2-oxo-1-azacyclopentadec-3-yl)benzamide (18a). From 16a (0.20 g, 0.42 mmol) in toluene (373 mL). Yield: 0.048 g (27%) of 18a. Colorless solid, mp 257.7–265.8 °C (decomp.). Crystals suitable for an X-ray crystal-structure determination were obtained from Et2O/hexane/CH2Cl2. IR (CHCl3): 3620w (br), 3450w, 3380w, 3100w, 3060w, 3030w, 3010w, 2940s, 2880w, 2860m, 1675m, 1650s, 1605w, 1580w, 1510w, 1485s, 1465m, 1455m, 1265m, 1230w, 1215w, 1205w, 1125m (br), 1050w, 1030w, 880w, 715m, 705m. 1H-NMR (CDCl3): 7.75–7.70 (m, 2 arom. H); 7.63 (s, NH); 7.50–7.45 (m, 1 arom. H); 7.45–7.35 (m, 2 arom. H); 7.20–7.15 (m, 3 arom. H); 7.10–7.00 (m, 2 arom. H); 5.80–5.30 (m, CH2NH); 3.85, 3.10 (AB, J = 13.8, PhCH2); 3.85–3.70 (m, 1 H of CH2N); 3.20–2.95 (m, 1 H of CH2N + 1 H); 1.80–1.00 (m, 21 H). 13C-NMR (CDCl3): 172.5, 166.5 (2s, 2 CO); 136.1, 135.4 (2s, 2 arom. C); 131.3 (d, 1 arom. CH); 129.8, 128.5, 128.2 (3d, 2 arom. CH each); 126.9 (d, 3 arom. CH); 67.4 (s, Cq); 65.3 (t, CH2N); 41.9 (t, PhCH2); 39.7, 36.4, 29.7, 27.2, 27.0, 26.7, 26.4, 26.2, 25.8, 24.9, 23.2 (11t, 11 CH2). ESI-MS (MeOH): 480 (100, [M+2 Na]+), 466 (5, [M+MeOH]+), 435 (8, [M+1]+).
N-(3-Benzyl-2-oxo-1-azacyclotetradec-3-yl)benzamide (18b). From 16b (0.07 g, 0.15 mmol) in toluene (100 mL). Yield: 0.019 g (30%) of pure 18b (and 0.025 g of a mixture of 18b and Me2NH2Cl). Colorless solid, mp 267.0–268.0 °C (decomp.). Crystals suitable for an X-ray crystal-structure determination were obtained from hexane/CH2Cl2. IR (CHCl3): 3450w, 3370w, 3000w, 2995w, 2935s, 2860m, 1670m, 1650s, 1605w, 1580w, 1510s, 1485s, 1460m, 1445w, 1365w, 1355w, 1130w, 1030w, 910w, 880w, 700m. 1H-NMR (CDCl3): 7.75–7.70 (m, 2 arom. H); 7.64 (br. s, NH); 7.50–7.35 (m, 3 arom. H); 7.25–7.15 (m, 3 arom. H); 7.10–7.05 (m, 2 arom. H); 5.91 (br t, CH2NH); 3.90, 3.09 (AB, J = 13.8, PhCH2); 3.75–3.65 (m, 1 H of CH2N); 3.15–2.95 (m, 1 H of CH2N + 1 H); 1.80–1.05 (m, 19 H). 13C-NMR (CDCl3): 172.4, 166.3 (2s, 2 CO); 136.0, 135.4 (2s, 2 arom. C); 131.2 (d, 1 arom. CH); 129.7, 128.4, 128.0 (3d, 2 arom. CH each); 126.8 (d, 3 arom. CH); 65.2 (s, Cq); 61.8 (t, CH2N); 39.3 (t, PhCH2); 36.2, 27.8, 26.0, 25.9, 25.7, 25.0, 23.2, 23.0, 22.9, 21.2 (10t, 10 CH2). CI-MS (i-butane): 421 (100, [M+1]+).
N-(3-Benzyl-2-oxo-1-azacyclotridec-3-yl)benzamide (18c). From 16c (0.33 g, 0.80 mmol) in toluene (300 mL). Yield: 0.034 g (11%) of 18c. Colorless solid, mp 250.0–251.0 °C (decomp.). IR (CHCl3): 3440w, 3360w, 3050w, 2995w, 2920s, 2850m, 1670m, 1645s, 1600w, 1575w, 1510s, 1480s, 1460m, 1440m, 1340w, 1320w, 1290w, 1260w, 1140w, 1115m, 880w, 700m. 1H-NMR (CDCl3): 7.75–7.70 (m, 2 arom. H); 7.61 (br. s, NH); 7.50–7.45 (m, 1 arom. H); 7.45–7.35 (m, 2 arom. H); 7.20–7.10 (m, 3 arom. H); 7.10–7.05 (m, 2 arom. H); 5.79 (br d, CH2NH); 3.90, 3.10 (AB, J = 13.9, PhCH2); 3.80–3.70 (m, 1 H of CH2N); 3.20–2.95 (m, 1 H of CH2N + 1 H); 1.85–1.70 (m, 1H); 1.70–1.15 (m, 16 H). CI-MS (i-butane): 407 (100, [M+1]+).
12-Amino-2-benzamido-2-benzyldodecanoic acid (19c). An analogous experiment with 16c in non-dried toluene gave 51% of 19c. Colorless solid, mp 144.0–145.0 °C (decomp.). IR (KBr): 3680–2340s (br), 3420m, 3350m, 3050m, 2920s, 2850s, 1660s, 1640s, 1630s, 1600s, 1570s, 1510s, 1475s, 1455s, 1440s, 1385s, 1325m, 1310m, 1180w, 1115w, 1070w, 1030w, 1000w, 875w, 700s. 1H-NMR (CDCl3): 7.74 (s, NH); 7.65–7.55 (m, 2 arom. H); 7.45–7.30 (m, 3 arom. H); 7.15–7.05 (m, 5 arom. H); 3.71, 3.10 (AB, J = 13.1, PhCH2); 3.60–3.00 (m, NH2); 2.76 (t-like, J ≈ 7.6, CH2N); 2.65–2.60 (m, 1 H); 1.95–1.80 (m, 1H); 1.75–1.05 (m, 8 CH2). 13C-NMR (CD3OD): 178.5, 168.5 (2s, 2 CO); 139.5, 137.1 (2s, 2 arom. C); 132.3 (d, 1 arom. CH); 130.9, 129.6, 128.7, 127.5 (4d, 2 arom. CH each); 127.1 (d, 1 arom. CH); 68.5 (s, Cq); 42.1 (t, CH2N); 40.6 (t, PhCH2); 36.9, 30.5, 30.3 (3t, 3 CH2); 30.1 (t, 2 CH2); 30.0, 28.4, 27.3, 25.6 (4t, 4 CH2). ESI-MS (MeOH): 463 (18, [M+K]+), 447 (100, [M+Na]+), 425 (35, [M+1]+).
BF3 catalyzed reactions with 16a. To a solution of 16a (0.15 g, 0.31 mmol) in toluene (60 mL) at 100 °C, BF3.Et2O (0.47 mmol) in CH2Cl2 was added and the mixture stirred for 19 h. Evaporation of the solvent and FCC (Et2O/hexane 1:1 and then CHCl3/MeOH/NH3 85:14:1) gave lactam (18a) (0.009 g, 7%), an unstable product (20a) (0.013 g, 10%), azlactone (17a) (0.040 g, 30%), and the acid (21a) (0.049 g, 35%). The unstable 20a converted into 17a.
4-(12-Aminododecyl-4-benzyl-1,3-oxazol-5(4H)-one (17a). Colorless oil. IR (CHCl3): 3650w, 3580w, 3360w, 3260w, 3020m, 2920s, 2850m, 1810m, 1655s, 1600w, 1580w, 1510m, 1490m, 1480m, 1450m, 1320m, 1290m, 1240w, 1130m (br), 1065s, 1040s, 1020s, 970m, 930m, 910m, 700s. 1H-NMR (CDCl3): 7.85–7.80 (m, 2 arom. H); 7.60–7.50 (m, 1 arom. H); 7.50–7.35 (m, 2 arom. H); 7.15–7.05 (m, 5 arom. H); 3.90–3.80 (m, NH2); 3.21, 3.14 (AB, J = 13.4, PhCH2); 3.00–2.85 (m, 2 H); 2.05–1.95 (m, 2 H); 1.75–1.55 (m, 5 H); 1.40–1.10 (m, 15 H). CI-MS (NH3): 435 (100, [M+1]+).
2-Amino-14-benzamido-2-benzyltetradecanoic acid (21a). Colorless solid, mp 121.7–122.2 °C. IR (CHCl3): 3700–3210m, 3210–2200s, 3110m, 3050m, 3020m, 2920s, 2840s, 1650m, 1640s, 1630s, 1575s, 1510s, 1480s, 1465s, 1450m, 1440m, 1385s, 1325w, 1310w, 1180w, 1115w, 1075w, 1025w, 1000w, 875w, 700m. 1H-NMR (CDCl3): 7.66 (s, NH); 7.55–7.50 (m, 2 arom. H); 7.40–7.35 (m, 1 arom. H); 7.35–7.25 (m, 2 arom. H); 7.10–7.00 (m, 5 arom. H); 3.70, 3.09 (AB, J = 13.1, PhCH2); 3.30–3.25 (m, 1 H); 2.80–2.75 (m, 2 H); 2.55–2.50 (m, 1 H); 1.90–1.50 (m, 2H); 1.40–1.00 (m, 18 H). CI-MS (NH3): 453 (100, [M+1]+), 435 (11, [M–H2O]+), 409 (100, [M–CO2]+).
Methyl 2-amino-14-benzamido-2-benzyltetradecanoate (22a). In an analogous experiment, a solution of 16a (0.15 g, 0.31 mmol) in toluene (62 mL) and BF3.Et2O (0.47 mmol) in CH2Cl2 at 90 °C was stirred for 2 h. Then, MeOH (0.06 g, 1.88 mmol) was added and the mixture heated to reflux for 21 h. Evaporation of the solvent and FCC (CHCl3/MeOH/NH3 85:14:1) gave 0.116 g (80%) of 22a. Colorless thick oil. IR (CHCl3): 3400m (br), 3050w, 2980m, 2920s, 2850s, 1730m, 1660s, 1600w, 1580w, 1515s, 1485s, 1450m, 1370w, 1350m, 1240m, 1180m, 1170–1090s, 1070s, 1025s, 880w, 700m. 1H-NMR (CDCl3): 7.70–7.65 (m, 2 arom. H); 7.55–7.45 (m, 1 arom. H); 7.45–7.35 (m, 2 arom. H); 7.20–7.15 (m, 3 arom. H); 7.05–6.95 (m, 2 arom. H); 6.96 (s, NH); 4.10–3.70 (m, NH2); 3.91, 3.16 (AB, J = 13.5, PhCH2); 3.82 (s, MeO); 2.92 (t, J = 7.7, CH2N); 2.85–2.70 (m, 1 H); 2.00–1.85 (m, 1 H); 1.65–1.55 (m, CH2); 1.40–1.10 (m, 9 CH2). 13C-NMR (CDCl3): 173.9, 166.7 (2s, 2 CO); 136.3, 135.1 (2s, 2 arom. C); 131.4 (d, 1 arom. CH); 129.5, 128.5, 128.1, 126.6 (4d, 2 arom. CH each); 126.7 (d, 1 arom. CH); 66.4 (s, Cq); 52.6 (q, MeO); 40.64, 40.60, 40.4, 35.1 (4t, 4 CH2); 29.4 (t, 3 CH2); 29.2 (t, 2 CH2); 29.0, 28.9, 26.3, 24.2 (4t, 4 CH2). CI-MS (NH3): 481 (5, [M+NH4]+), 467 (100, [M+1]+).
BF3 catalyzed reaction with 16c. To a solution of 16c (0.137 g, 0.30 mmol) in toluene (150 mL) at 100 °C, BF3.Et2O (0.30 mmol) in CH2Cl2 was added and the mixture stirred for 13 h. Then, additional BF3.Et2O (0.30 mmol) was added, the mixture stirred at 100 °C for 7.5 h, diisopropyl(ethyl)amine (2 x 30 mmol) added, and the boiling mixture stirred for 13 h. Evaporation of the solvent and FCC (Et2O/hexane 1:1 and then CHCl3/MeOH/NH3 85:14:1) gave the lactam (18c) (0.010 g, 8%) and a less stable product (20c) (0.028 g, 23%).
13-Benzyl-1-phenyl-15-oxa-2,16-diazabicyclo[12.2.1]octadecan-14-one (20c). Colorless oil, which slowly decomposed at rt. IR (CHCl3): 3660w, 3580w, 3350w, 3300w (br), 3010w, 2920s, 2850m, 1810s, 1650s, 1575w, 1490w, 1460w, 1450m, 1390w, 1320m, 1290m, 1225w, 1205w, 1130s (br), 1040m, 970s (br), 890w, 695s. 1H-NMR (CDCl3): 7.85–7.80 (m, 2 arom. H); 7.60–7.45 (m, 1 arom. H); 7.45–7.35 (m, 2 arom. H); 7.20–7.10 (m, 5 arom. H); 7.10–6.60 (m, NH); 4.15–4.10 (m, NH); 3.20, 3.12 (AB, J = 13.3, PhCH2); 3.00–2.85 (m, CH2); 2.05–1.95 (m, CH2); 1.70–1.55 (m, CH2); 1.30–1.10 (m, 7 CH2). 13C-NMR (CD3OD): 179.9 (s, CO); 134.4 (s, 1 arom. C); 132.5 (d, 1 arom. CH); 130.1, 128.7, 128.1, 127.7 (4d, 2 arom. CH each); 127.1 (d, 1 arom. CH); 125.7 (s, 1 arom. C); 94.2 (s, NCN); 74.9 (s, NCq); 43.8 (t, CH2N); 41.5 (t, PhCH2); 40.3, 37.4 (2t, 2 CH2); 29.3 (t, 3 CH2); 27.4, 26.3, 24.0, 19.0 (4t, 4 CH2). CI-MS (NH3): 407 (100, [M+1]+).
X-Ray Crystal-Structure Determination of 18a and 18b (Figure 1).63 All measurements were made on a Rigaku AFC5R diffractometer using graphite-monochromated MoKα radiation (λ = 0.71073 Å) and a 12kW rotating anode generator. The intensities were corrected for Lorentz and polarization effects, but not for absorption. Equivalent reflections were merged. Data collection and refinement parameters are given below, and views of the molecules are shown in Figure 1. The structures of 18a and 18b were solved by direct methods using SHELXS86,64 respectively, which revealed the positions of all non-hydrogen atoms. In both cases, the macrocyclic ring is disordered and two sets of positions were defined for five and seven consecutive CH2 groups, respectively. The site occupation factor of the major conformation of these groups refined to 0.662(4) and 0.667(5), respectively. Similarity restraints were applied to the chemically equivalent bond lengths and angles involving all disordered C-atoms, while neighboring atoms within and between each disordered conformation were restrained to have similar atomic displacement parameters. The non-hydrogen atoms were refined anisotropically. The amide H-atoms were placed in the positions indicated by difference electron density maps and their positions were allowed to refine together with individual isotropic displacement parameters. All remaining H-atoms were placed in geometrically calculated positions and refined by using a riding model where each H-atom was assigned a fixed isotropic displacement parameter with a value equal to 1.2Ueq of its parent C-atom. The refinement of each structure was carried out on F2 by using full-matrix least-squares procedures, which minimized the function Σw(Fo2–Fc2)2. Corrections for secondary extinction were not applied. Neutral atom scattering factors for non-H-atoms were taken from ref.65, and the scattering factors for H-atoms were taken from ref.66 Anomalous dispersion effects were included in Fc;67 the values for ƒ’ and ƒ” were those of ref.68 The values of the mass attenuation coefficients are those of ref.69 All calculations were performed using the SHELXL97 program.70 Crystal data for 18a: Crystallized from Et2O/hexane/CH2Cl2, C28H38N2O2, M = 434.60, colorless, prism, crystal dimensions 0.18 × 0.23 × 0.40 mm, monoclinic, space group C2/c, Z = 8, reflections for cell determination 23, 2θ range for cell determination 21–37°, a = 26.026(5) Å, b = 10.833(3) Å, c = 21.330(4) Å, β = 119.83(1)°, V = 5217(2) Å3, DX = 1.107 g⋅cm–3, μ(MoKα) = 0.069 mm–1, T = 295 K, ω scans, 2θmax = 55°, total reflections measured 6444, symmetry independent reflections 5989, reflections with I > 2σ(I) 2327, reflections used in refinement 5989, parameters refined 370, restraints 223, final R (on F; I > 2σ(I) reflections) = 0.0751, wR = 0.2682 (w = [σ2(Fo2) + (0.1293P)2]–1 where P = (Fo2 + 2Fc2)/3, goodness of fit 1.006, final Δmax/σ = 0.002, Δρ (max; min) = 0.33; –0.33 e Å–3.
Crystal data for 18b: Crystallized from CH2Cl2/hexane, C27H36N2O2, M = 420.58, colorless, plate, crystal dimensions 0.10 × 0.35 × 0.47 mm, monoclinic, space group C2/c, Z = 8, reflections for cell determination 23, 2θ range for cell determination 24–45°, a = 24.951(3) Å, b = 10.683(4) Å, c = 20.996(2) Å, β = 118.377(7)°, V = 4924(2) Å3, DX = 1.135 g⋅cm–3, μ(MoK<συβ>α</συβ>) = 0.071 mm–1, T = 173(1) K, ω/2θ scans, 2θmax = 55°, total reflections measured 6134, symmetry independent reflections 5692, reflections with I > 2σ(I) 3655, reflections used in refinement 5692, parameters refined 335, restraints 117, final R (on F; I > 2σ(I) reflections) = 0.0485, wR = 0.1419 (w = [σ2(Fo2) + (0.0637P)2 + 1.4002P]–1 where P = (Fo2 + 2Fc2)/3, goodness of fit 1.021, final Δmax/σ = 0.001, Δρ (max; min) = 0.35; –0.21 e Å–3.
ACKNOWLEDGEMENTS
We thank the analytical services of our institute for analyses and spectra. Financial support by the Swiss National Science Foundation and F. Hoffmann-La Roche AG, Basel, is gratefully acknowledged.
References
1. Part of the Ph.D. thesis of S.P.F., University of Zürich, 1995; present address: Carbogen Amcis AG, Schachenallee 29, CH-5001 Aarau, Switzerland.
2. A. Budzowski, A. Linden, and H. Heimgartner, Helv. Chim. Acta, 2008, 91, 1471. CrossRef
3. T. Jeremic, A. Linden, and H. Heimgartner, J. Pept. Sci., 2008, 14, 1051. CrossRef
4. K. A. Brun, A. Linden, and H. Heimgartner, Helv. Chim. Acta, 2008, 91, 526. CrossRef
5. J. L. Räber, K. A. Brun, and H. Heimgartner, Heterocycles, 2007, 74, 397. CrossRef
6. W. Altherr, A. Linden, and H. Heimgartner, Chem. Biodivers., 2000, 4, 1144. CrossRef
7. S. Stamm and H. Heimgartner, Helv. Chim. Acta, 2006, 89, 1841. CrossRef
8. S. Stamm and H. Heimgartner, Tetrahedron, 2006, 62, 9671. CrossRef
9. P. Köttgen, A. Linden, and H. Heimgartner, Helv. Chim. Acta, 2006, 89, 731. CrossRef
10. S. Stamm, A. Linden, and H. Heimgartner, Helv. Chim. Acta, 2006, 89, 1. CrossRef
11. N. Pradeille, O. Zerbe, K. Moehle, A. Linden, and H. Heimgartner, Chem. Biodivers., 2005, 2, 1127. CrossRef
12. T. Jeremic, A. Linden, K. Moehle, and H. Heimgartner, Tetrahedron, 2005, 61, 1871. CrossRef
13. T. Jeremic, A. Linden, and H. Heimgartner, Helv. Chim. Acta, 2004, 87, 3056. CrossRef
14. T. Jeremic, A. Linden, and H. Heimgartner, Chem. Biodivers., 2004, 1, 1730. CrossRef
15. S. Stamm and H. Heimgartner, Eur. J. Org. Chem., 2004, 3820. CrossRef
16. R. T. N. Luykx, A. Linden, and H. Heimgartner, Helv. Chim. Acta, 2003, 86, 4093. CrossRef
17. H. Heimgartner, Angew. Chem., Int. Ed. Engl., 1991, 30, 238. CrossRef
18. P. Wipf and H. Heimgartner, Helv. Chim. Acta, 1990, 73, 13. CrossRef
19. M. Sahebi, P. Wipf, and H. Heimgartner, Tetrahedron, 1989, 45, 2999. CrossRef
20. P. Wipf and H. Heimgartner, Helv. Chim. Acta, 1987, 70, 354. CrossRef
21. D. Obrecht and H. Heimgartner, Helv. Chim. Acta, 1987, 70, 102. CrossRef
22. P. Wipf and H. Heimgartner, Helv. Chim. Acta, 1986, 69, 1153. CrossRef
23. D. Obrecht and H. Heimgartner, Helv. Chim. Acta, 1981, 64, 482. CrossRef
24. D. Obrecht and H. Heimgartner, Chimia, 1982, 36, 7.
25. C. Theis, T. Degenkolb, and H. Brückner, Chem. Biodivers., 2008, 5, 2337. CrossRef
26. K. N. Koch, A. Linden, and H. Heimgartner, Tetrahedron, 2001, 57, 2311. CrossRef
27. K. N. Koch, A. Linden, and H. Heimgartner, Helv. Chim. Acta, 2000, 83, 1881. CrossRef
28. K. N. Koch, A. Linden, and H. Heimgartner, Helv. Chim. Acta, 2000, 83, 233. CrossRef
29. B. Iliev, A. Linden, R. Kunz, and H. Heimgartner, Tetrahedron, 2006, 62, 1079. CrossRef
30. B. Iliev, A. Linden, and H. Heimgartner, Helv. Chim. Acta, 2003, 86, 3215. CrossRef
31. H. Heimgartner, F. S. Arnhold, S. P. Fritschi, K. N. Koch, J. E. F. Magirius, and A. Linden, J. Heterocycl. Chem., 1999, 36, 1539. CrossRef
32. R. Filler, Adv. Heterocycl. Chem., 1965, 4, 75. CrossRef
33. W. Steglich, Fortschr. Chem. Forsch., 1969, 12, 77. CrossRef
34. R. Filler and Y. S. Rao, Adv. Heterocycl. Chem., 1977, 21, 175. CrossRef
35. A. K. Mukerjee and P. Kumar, Heterocycles, 1981, 16, 1995. CrossRef
36. A. K. Mukerjee, Heterocycles, 1987, 26, 1077. CrossRef
37. U. Wittmann, F. Tranel, R. Froehlich, and G. Haufe, Synthesis, 2006, 2085.
38. B. M. Trost and C. Lee, J. Am. Chem. Soc., 2001, 123, 12191. CrossRef
39. N. Devi, M. D. Threadgill, and S. J. B. Tendler, Synth. Commun., 1988, 18, 1545. CrossRef
40. D. Obrecht, B. Scholl, and H. Heimgartner, Helv. Chim. Acta, 1985, 68, 465. CrossRef
41. R. Lohmar and W. Steglich, Chem. Ber., 1980, 113, 3706. CrossRef
42. W. Steglich, V. Austel, and A. Prox, Angew. Chem., Int. Ed. Engl., 1968, 7, 726. CrossRef
43. M. Sedlak, R. Keder, P. Skala, and J. Hanusek, J. Phys. Org. Chem., 2005, 18, 743. CrossRef
44. M. Altamura, R.-M. Catalioto, D. Giannotti, A. Giolitti, S. Guliani, A. Guidi, N. J. S. Harmat, A. Lecci, S. Meini, R. Nannicini, F. Pasqui, M. Tramontana, A. Triolo, and C. A. Maggi, J. Med. Chem., 2007, 50, 4793. CrossRef
45. D. Obrecht, C. Spiegler, P. Schönholzer, K. Müller, H. Heimgartner, and F. Stierli, Helv. Chim. Acta, 1992, 75, 1666. CrossRef
46. D. Obrecht and H. Heimgartner, Tetrahedron Lett., 1984, 25, 1717. CrossRef
47. F. M. F. Chen, K. Kuroda, and N. L. Benoiton, Synthesis, 1979, 230. CrossRef
48. D. F. DeTar, R. Silverstein, and F. F. Rogers Jr., J. Am. Chem. Soc., 1966, 88, 1024. CrossRef
49. S. Götze, B. Kübel, and W. Steglich, Chem. Ber., 1976, 109, 2331. CrossRef
50. B. Kübel, P. Gruber, R. Hurnhaus, and W. Steglich, Chem. Ber., 1979, 112, 128. CrossRef
51. D. Obrecht, U. Bohdal, R. Ruffieux, and K. Müller, Helv. Chim. Acta, 1994, 77, 1423. CrossRef
52. M. L. Gelmi, D. Pocar, and L. M. Rossi, Synthesis, 1984, 763. CrossRef
53. K. Hojo, S. Kobayashi, K. Soai, S. Ikedea, and T. Mukaiyama, Chem. Lett., 1977, 635. CrossRef
54. H. Staudinger and J. Meier, Helv. Chim. Acta, 1919, 2, 635. CrossRef
55. N. Knouzi, M. Vaultier, and R. Carrié, Bull. Soc. Chim. Fr., 1985, 815.
56. W. Q. Tian and Y. A. Wang, J. Org. Chem., 2004, 69, 4299. CrossRef
57. No reaction occurred by treatment of 15b with Ph3P under the conditions of ref.55, whereas with Bu3P a slow evolution of gas was observed.
58. In the case of 15b, small amounts (<5%) of the corresponding lactam 18b were obtained, when the reaction mixture was dissolved in toluene and heated under reflux (neutral conditions).
59. W. C. Still, M. Kahn, and A. Mitra, J. Org. Chem., 1978, 43, 2923. CrossRef
60. C. K. Johnson, ORTEPII, Report ORNL-5138; Oak Ridge National Laboratory, Oak Ridge, Tennessee, 1976.
61. J. Bernstein, R. E. Davis, L. Shimoni, and N.-L. Chang, Angew. Chem., Int. Ed. Engl., 1995, 34, 1555. CrossRef
62. We believe that in the corresponding 4-(ω-hydroxyalkyl)-1,3-oxazol-5(4H)-ones the oxazoline N-atom is the preferred site for protonation.
63. CCDC-706638 and 706639 contain the supplementary crystallographic data for this paper. These data can be obtained free of charge from the Cambridge Crystallographic Data Centre via www.ccdc.cam.ac.uk/data_request/cif.
64. G. M. Sheldrick, SHELXS86, Acta Crystallogr., Sect. A, 1990, 46, 467. CrossRef
65. E. N. Maslen, A. G. Fox, and M. A. O’Keefe, in ‘International Tables for Crystallography’, ed. by A. J. C. Wilson, Kluwer Academic Publishers, Dordrecht, 1992, , Vol. C, Table 6.1.1.1, p. 477.
66. R. F. Stewart, E. R. Davidson, and W. T. Simpson, J. Chem. Phys., 1965, 42, 3175. CrossRef
67. J. A. Ibers and W. C. Hamilton, Acta Crystallogr., 1964, 17, 781. CrossRef
68. D. C. Creagh and W. J. McAuley, in ‘International Tables for Crystallography’, ed. by A. J. Wilson, Kluwer Academic Publishers, Dordrecht, 1992, Vol. C, Table 4.2.6.8, pp. 219–222.
69. D. C. Creagh and J. H. Hubbell, in ‘International Tables for Crystallography’, ed. by A. J. C. Wilson, Kluwer Academic Publishers, Dordrecht, 1992, , Vol. C, Table 4.2.4.3, pp. 200–206.
70. G. M. Sheldrick, SHELXL79, Program for the Refinement of Crystal Structures, University of Göttingen, Germany, 1997.