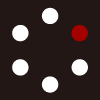
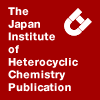
HETEROCYCLES
An International Journal for Reviews and Communications in Heterocyclic ChemistryWeb Edition ISSN: 1881-0942
Published online by The Japan Institute of Heterocyclic Chemistry
e-Journal
Full Text HTML
Received, 4th November, 2008, Accepted, 26th January, 2009, Published online, 26th January, 2009.
DOI: 10.3987/COM-08-S(D)80
■ Synthesis and Spectroscopic Comparison of the Eight Methyl-2,3’-bipyridyls and Identification of a Hoplonemertine Alkaloid as 3-Methyl-2,3’-bipyridyl
William R. Kem,* James Rocca, H. Martin Garraffo, Thomas F. Spande, John W. Daly, and Ferenc Sóti
Department of Pharmacology and Therapeutics, College of Medicine, University of Florida, 1600 SW Archer Rd., Gainesville, FL 32610-0267, U.S.A.
Abstract
The pyridyl ring is frequently found in natural products and drugs. While bipyridyls have served as useful scaffolds for development of industrial and pesticidal chemicals, their biological properties are still not well understood. Only 2,3’-bipyridyl, of the six isomeric bipyridyls, has been reported as a natural product in tobacco plants and in a hoplonemertine marine worm, Amphiporus angulatus (Aa), which uses its pyridyl alkaloid-rich venom to paralyze its crustacean prey and chemically defend itself against predators. Here we report for the first time the synthesis and spectroscopic properties of all eight possible methyl 2,3’-bipyridyl isomers and use this data to identify a trace alkaloidal constituent of Aa as 3-methyl-2,3’-bipyridyl. This is only the second reported instance of a methyl-bipyridyl being found as a natural product, the first being the tobacco alkaloid 5-methyl-2,3’-bipyridyl.INTRODUCTION
Nemertines, a phylum of free-living marine worms, use a variety of heterocyclic, peptide and protein toxins to defend themselves against predators.1 One major group, the hoplonemertines also utilize their heterocyclic toxins to paralyze their prey and repel potential predators. The worm everts its proboscis, exposing a mineralized stylet (See dagger-like structure on the right side of drawing in the graphical abstract) that, by puncturing the skin of the intended victim several times, facilitates the entry of venom alklaoids. Anabaseine, 2-(3-pyridyl)-3,4,5,6-tetrahydropyridine, was the first nemertine alkaloid identified, although nemertine alkaloids were discovered eighty years ago.2 It possesses a pharmacological profile that is different from nicotine in that it preferentially stimulates muscle and α7 subtype nicotinic acetylcholine receptors, whereas the tobacco compound preferentially stimulates heteromeric neuronal nicotinic receptors.3 A synthetic derivative of anabaseine, 3-(2,4-dimethoxybenzylidene)-anabaseine, selectively stimulates α7-type nicotinic receptors and has been found to improve cognition in clinical tests on normal and schizophrenic humans.4
The chevron (the triangular mark on the head can be seen in the drawing, graphical abstract) hoplonemertine Amphiporus angulatus is a relatively large hoplonemertine that preys upon small shrimp-like crustaceans (amphipods), which it paralyzes with its venom. The nemertine’s skin also contains the alkaloids, which serve as a chemical defence against predators. In our initial report, a methyl-bipyridyl was detected in trace amounts along with much greater amounts of the crustacean-toxic 2,3’-bipyridyl hereafter referred to as BP, a tetrapyridyl named nemertelline and several other alkaloids (Figure 1).5 Identification of the compound was initially unsuccessful because its LC and GC retention times were very similar to BP, which is present in much greater amounts in this species. Due to the high volatility of microgram amounts of the HPLC purified natural product, it was difficult to remove the various HPLC solvents associated with it under reduced pressure (even at 30 oC) without drastic losses. Assuming that the compound was a methylated 2,3’-bipyridyl, we then resorted to the use of several online microanalytical techniques such as LC-MS, GC-MS and GC-FTIR-MS to determine the chemical properties of our presumed methyl-BP natural product relative to those of the eight possible methyl BPs that we synthesized. Finally, we obtained a proton NMR spectrum with a few micrograms of the HPLC-purified, air-dried compound, which allowed the unequivocal identification of the compound as 3-methyl-BP.
RESULTS AND DISCUSSION
Methyl-BP Synthesis: General Comments
There are 30 possible methyl-bipyridyls, including eight methyl-BPs. Because other bipyridyl hoplonemertine alkaloids contain 2,3’ bonds (excepting the two non-terminal pyridyl rings of nemertelline, which have a 4,3’ connectivity)6 the Aa natural product was likely a methyl-BP. While NMR and mass spectral data for four methyl-BPs have been reported, spectral data for the other four isomers was lacking or unsatisfactory and they had not been prepared in an unequivocal manner. To assist in the future identification of microgram amounts of these compounds all eight isomers were prepared to allow comparison of their chemical and biological properties.
Several methods were available for the synthesis of these isomers. The most efficient methods would utilize either Suzuki or Stille type cross-coupling reactions or aromatization of the appropriate methyl-anabaseines, which we had already prepared for pharmacological studies. The palladium-catalyzed Suzuki cross-coupling reaction was used for synthesis of the 4-, 5- and 6-methyl-2,3’-bipyridyls.7 Aromatization of the piperidine ring of anabasine8 and the tetrahydropyridyl rings of both anabaseine9 and anatabine10 have been described. We chose the dehydrogenation method of Kimpe et al.9 as the most suited to our purposes, since we had the methyl-anabaseine starting materials for most of the methyl bipyridyls and because this method consists of only two simple steps, each with a good yield. The 4-, 5-, 2'-, 4'-, 5'-, and 6'-methyl-BPs were prepared by this method as described in the next section. The methyl-anabaseine precursors were generally prepared by Claisen condensation of the appropriate nicotinic acid ester with the appropriate piperidone;11 their syntheses will be described elsewhere, along with their pharmacological properties.
3-Me-BP was prepared from BP-3-carboxylic acid, previously prepared from 1,10-phenanthroline by the Skraup method,12 by reduction to 3-hydroxymethyl-BP and subsequent catalytic hydrogenolysis, as described below. 6-Me-BP was prepared by a palladium-catalyzed cross-coupling reaction according to Bloom.13 Synthetic BP was prepared by oxidation of natural anabasine, as previously described.14 All of the synthetic bipyridyls, purified by SG column chromatography as their free bases, were homogeneous by SG TLC and NMR analysis. Like BP, they were oils at rt.
LC, GC and Spectroscopic Properties of the Aa Natural Product and Synthetic Me-BPs
The Aa natural product had the molecular size (170) and elemental composition expected for a Me-BP. Our best estimate of its abundance in Aa (17% of the BP peak area) is based on the LC-CI/MS measurement of the total ion currents of the two peaks in the crude BP-containing Aa fraction, as shown in Figure 3. Thus, relative to BP, present at a level of approximately 120 µg/g fresh weight of Aa, the methyl-bipyridyl is a relatively inconspicuous but significant (estimate: 20 µg/g fresh weight of tissue) constituent of Aa.
Direct probe introduction EI-MS at UF and GC-MS at NIH of the initial silica gel low pressure chromatography BP containing fraction of Aa revealed that the trace constituent displayed a molecular ion of m/z170 (found, 170.0838; theor., 170.0843, C11H10N2) and prominent fragments at m/z 169 (M-1; found, 169.0765, theor., 169.0765, C11H9N2), 168 (M-2), 143 (M-27; found, 143.0755, theor., 143.0734, C10H9N2), 142 (M-28; found, 142.0620, theor., 142.0656, C10H8N2), and 115 (M-65); the full list of fragments is provided in Table 2 for comparison with the eight synthetic methyl-BPs. The mass spectra indicated that there were major fragmentations resulting in (M-1) and (M-28), as expected for a methyl-bipyridyl.
The mass spectral and other properties of the eight synthetic methyl-BPs and the Aa natural product can be compared in Tables 2 and 3. Besides serving as a basis for identification of the natural product as 3-methyl-BP, this compendium of properties should be useful in future structure determinations of small quantities of environmental methyl-bipyridyls.
Although mass spectrometric identification of isomers is often difficult due to the similarity of their spectra, analysis of peak intensities for the methyl-BPs was quite informative and useful in identifying the Aa trace constituent. We made some generalizations and then applied them to the analysis of the natural product spectrum.
The molecular ions (M+.) of the 2’-, 4’- and 3-methyl-BPs were much less abundant relative to the M+.-1 ions (only 15-20% of the intensity of M+.-H.), as might be expected to occur as a result of diminished stability due to steric hindrance of coplanarity between the two pyridyl rings. Second, the M+.-15 (m/z 155) fragments were only abundant (>20%) for the 5’ and 6’-methyl-BPs. Third, the M-26 (m/z 144) fragments were only abundant in the spectra of the 4-, 5- and 6-methyl-BPs. Finally, the ratio M-28 (m/z 142) / M-32 (m/z168) was almost 2 for all four 2-pyridyl ring isomers (3-, 4-, 5- and 6-methyl-BPs) but was
essentially 1 for the 2’- and 4’-methyl-BPs; the ratio for the remaining 5’- and 6’-isomers was practically 3. Thus, the EI mass spectrum of a methyl-BP should permit tentative identification of any methyl isomer of this particular bipyridyl. The Aa trace constituent displayed a relatively low abundance of the m/z 170 molecular ion relative to the m/z 169 base peak ion, consistent with a 2’, 4’- or 3-methyl-BP structure. The M-28 (m/z 142) peak: M-32 (m/z 168) peak ratio was ~2, as expected for a methyl-BP containing a 2-pyridyl, but not a 2’- or 4’-methyl-BP. Based on the EI-MS data comparison with the eight possible Me-BPs (assuming it is a 2,3’-bipyridyl), the trace constituent can only be 3-Me-BP.
For three 3-Me-bipyridyls (i.e. 3-Me-2,2’-bipyridyl, 3-Me-BP and 3-Me-2,4’-bipyridyl), the analysis by GC-MS shows small but definite differences in m/z peak intensities (Figure 4) and in Rts (see Figure 4 legend). The first isomer has a shorter Rt than the other two. Also the first isomer shows a bigger M+ and smaller m/z 142 (M+-28) than the other two. The last isomer lacks the ion m/z 84. The natural compound appears identical to the second isomer, 3-Me-BP. However, for the same isomers, a striking difference is observed in the infrared spectra (see Figures 3 and 4). The second isomer, 3-Me-BP, is identical with the natural compound clearly in three physical properties: GC-Rt (in two columns in two instruments), MS and FTIR spectra.
An initial 500 MHz NMR spectrum (not shown) was obtained by J. Rocca (UF) from a microgram sample of the trace natural product still dissolved in the hexane-isopropanol-DEA eluate obtained from the normal phase HPLC analytical column. It showed the seven resolved aromatic proton signals and spin coupling constants (Table 3) characteristic of 3-methyl-2,3’-bipyridyl but the proton chemical shifts were affected by the complex solvent mixture (hexane, isopropanol and diethylamine). The region upfield from these aromatic protons displayed many solvent peaks in addition to the methyl protons characteristic of 3-methyl-BP. After the solvents were evaporated by exposure to air, proton signals of the natural product (Figure 7) were demonstrated to be identical with those of synthetic 3-methyl-BP (see comparison in Table 3).
One reason for our special interest in identifying the Aa methyl-bipyridyl was that Aa also possesses a still unidentified methyl-nemertelline, a tetrahydro-nemertelline and a hydroxy-nemertelline, in addition to nemertelline, which is the most abundant alkaloid of this species.5 A reasonable hypothesis regarding the biosynthesis of these tetrapyridyls is that they result from the reaction of a partially unsaturated bipyridyl with another, similar pyridyl precursor, followed by aromatization reactions. The methyl-bipyridyl and methyl-nemertelline likely originate from the same biosynthetic pathway, a precursor of the former compound serving as a starting compound for biosynthesis of the methyl-nemertelline. Thus, assignment of the methyl substitutions in these two Aa compounds would be a test of this hypothesis. The precursor compounds (each contributing two of the four eventual pyridyl rings) would be different dihydropyridines. Leete17 provided strong evidence that the two rings of the tobacco alkaloid anatabine are biosynthesized by coupling of twp dihydropyridyl ring precursors. Although anatabine is not found in the Aa extract, we have isolated and unequivocally identified its δ-3,4 isomer, which we refer to as isoanatabine, from this nemertine (Soti et al., in preparation). It is very interesting that tobacco plants contain 5-methyl-BP18 and anatabine,17 whereas A. angulatus contains 3-Me-BP and isoanatabine. One can surmise that the double bond placement determines the site of methylation of the tetrahydropyridine ring in both worm and plant.
We previously reported some biological activities of the various bipyridyls and Me-BPs, including BP.19,20 Besides their most conspicuous activity, causing a rapid convulsive paralysis of crayfish and other crustaceans, BP and the eight methyl-BPs also inhibit the surface attachment (settlement) of barnacle larvae, an activity which might have practical application in development of new, antifouling paint additivies that are not injurious to the marine environment. Naturally occurring 3-methyl-BP displays these dual biological activities. While the neurotoxic paralytic effects are thought to be due to interaction with nicotinic acetylcholine receptors within the crustacean central nervous system, the antisettlement activity of these pyridines on barnacle larvae may be due to their interaction with chemoreceptor neuron terminals located at the body surface that specifically respond to pyridine-containing heterocycles.20,21 Further studies on these compounds are needed to identify the receptors they target.
EXPERIMENTAL
Animal Collection and Initial Isolation of Pyridyl Alkaloids by Solvent Fractionation Amphiporus angulatus was collected at low tides along the rocky shoreline of Passamaquoddy Bay, which is bordered by northern Maine USA and New Brunswick Canada coasts [Adults often attain a 10 cm length and 1 g fresh weight]. Live worms were placed in approximately 5 volumes of methanol-1% acetic acid for rapid euthanization; this solvent also extracted most of the pyridine alkaloids. After evaporation of the solvent and successive extractions under acidic and basic conditions with CH2Cl2 as previously described,2 an orange-colored oil (approx. 10 mg/ g fresh weight of animal) containing a variety of pyridyl alkaloids and other compounds, was obtained. Several hundred Aa worms were collected over a span of more than two decades and worked up at different times for alkaloid isolation. Initial attempts at isolation of the trace constituent using preparative thin-layer and low pressure (Lobar) SG column chromatography yielded samples contaminated with 2,3’-bipyridyl and other pyridyl alkaloids that will be reported elsewhere.
HPLC Purification Normal phase HPLC (Beckman Gold Nouveau system, UF lab) used analytical or semipreparative 25 cm length ODS silica gel columns equilibrated with Solvent A: 98.5% hexane (Fisher Optima grade), 1% isopropanol (Fisher HPLC grade) and 0.5% diethylamine (Aldrich analytical grade). After 10 min. elution with Solvent A, a linearly increasing proportion of solvent B consisting of 50% hexane, 49.5% isopropanol and 0.5% diethylamine was applied. Flow rates were 1.0 mL/min (analytical column) and 3.0 mL/min (semiprep column). The most lipophilic compounds in the Aa alkaline CH2Cl2 extract, including BP and MBP, preferentially dissolved in Solvent A and were injected in volumes of 1 mL or less into a 2 mL injector loop. UV absorbing peaks were measured with a diode array detector (UV range 210-320 nm) and collected with an Isco Foxy Jr fraction collector using PeakTrak software. Whereas the UF lab used normal phase HPLC to separate the Aa natural product from the much more abundant 2,3’-bipyridyl (BP), the NIH lab resolved the two natural compounds in protonated form using a reversed-phase C18 analytical column, as described in the next section.
GC-MS, HPLC-MS and GC-FTIR Measurements (Daly lab at NIH) GC-MS analyses were performed on a Finnigan GCQ instrument equipped with a 25 m x 0.25 mm i.d. Rtx-5 Amine column using a temperature program from 100 °C (1 min) to 280 °C at a rate of 10 °C/min. GC-MS and -FTIR spectral analyses were performed with a Hewlett-Packard (HP) model 5890 gas chromatograph with a 25 m x 0.32 mm i.d. HP-5 column using the above temperature program, interfaced with an HP model 5971 Mass Selective Detector and an HP model 5965B FTIR instrument with a narrow range (4000-750 cm-1) detector and an HP ChemStation (DOS-based) to generate the chromatograms. HPLC-MS analyses were performed using an HP model 1100 liquid chromatograph interfaced with a Finnigan LCQ MS operating in the atmospheric pressure chemical ionization (APCI) mode. The HP Zorbax HPLC column (SB-C18, 25 cm x 4.6 mm i.d., 5 microns particle size) used a 0.5 mL/min flow rate and a solvent gradient from 9 parts of 0.1% HOAc in H2O and 1 part 0.1% HOAc in MeCN to a 1:1 mixture over 40 min. Samples of 10 uL were injected.
UV and NMR Spectroscopic Measurements UV spectra of the air-dried Aa natural product and rotary-evaporated synthetic methyl-bipyridyls were obtained in HPLC-grade ethanol at rt using a Beckman DU600 spectrophotometer. Absorbance data (λmax), determined for the free bases, are presented in Table 1. The proton NMR spectrum of the natural product was acquired with a Bruker Avance500 spectrometer equipped with a 2.5 mm TXI probe. All other NMR spectra were obtained on a Varian 300MHz instrument in CDCl3.
Synthesis of Methyl-BPs from the Corresponding Methyl-Anabaseines A mixture of the appropriate methyl-anabaseine free base (35 mg, 0.2 mmol) and N-chlorosuccinimide (67 mg, 5 mmol) in CCl4 (5 mL) was stirred overnight at rt. Aqueous NaHCO3 (0.3 g/ 3 mL) was added, and the aqueous solution was separated and then extracted with CCl4 (2x1 mL). The combined organic phase was dried (MgSO4) and evaporated in vacuo, giving the methyl-3,3-dichloroanabaseines in 68-98 % yield. These synthetic intermediates were pure enough by TLC and 1H-NMR for the next step. Each methyl-3,3- dichloroanabaseine was dissolved in a solution of sodium methoxide in dry methanol (0.5 mL of a 2 M solution, 1.0 mmol) and stirred at rt overnight. After removal of solvent by rotary evaporation, water (2 mL) was added and the aqueous solution was extracted with CH2Cl2 (5 x 1 mL). The combined CH2Cl2 extracts were dried (MgSO4), decolorized with activated carbon and evaporated under vacuum (2 mm Hg, 45 °C), yielding the pure methyl-BPs in 64-84 % yield. The GC and LC retention times and UV absorbance data are in Table 1, the EI-MS data for the natural product and the synthetic methyl-BPs are presented in Table 2, NMR data for these compounds are in Table 3 and finally, the NMR data for the dichloro synthetic intermediates are presented in Supplementary Table 4.
3-Methyl-BP Synthesis For synthesis of the 3-Hydroxymethyl-BP precursor, ethyl chloroformate (0.14 mL, 1.46 mmol) was added dropwise over 30 min to a continually stirred, ice-cold solution of BP-3-carboxylic acid (0.28 g, 1.40 mmol) in dry THF (10 mL) and triethylamine (0.20 mL, 1.43 mmol). The precipitated triethylamine hydrochloride was then filtered and washed several times with THF (3x2 mL). The THF washes were combined, cooled to 10 °C , and while stirring under an argon atmosphere, NaBH4 (0.185 g, 4.9 mmol) was added and then methanol (0.90 mL, 22.2 mmol) dropwise over a 1 h. during which time the temperature increased to 20 °C.15 After the material was stirred for an additional hr. at rt, 1 N HCl was carefully added, the solvent concentrated under vacuum to 5 mL and extracted with CH2Cl2 (3 x 5 mL). Na2CO3 (0.85 g) was added to the aqueous phase, which was then extracted with CH2Cl2. The combined fractions were dried (MgSO4) and evaporated under vacuum (2 mm, 45 °C) giving a pure product (0.22 g, 84 %). 1H-NMR (CDCl3), d, ppm, 8.71(1H, dd, J = 2.1, 0.6 Hz, C2'-H), 8.55 (1H, dd, J = 4.8, 1.5 Hz, C6-H), 8.52 (1H, dd, J = 4.8, 1.8 Hz, C6'-H), 7.95 (1H, dd, J = 7.8, 1.5 Hz, C4-H), 7.91 (1H, ddd, J = 7.8, 2.1, 1.8 Hz, C4'-H), 7.35 (1H, ddd, J = 7.8, 4.8, 0.6 Hz, C5'-H), 7.31 (1H, dd, J = 7.8, 4.8 Hz, C5-H), 4.58 (2H, s, CH2-O).
3-Methyl-BP: A 3-hydroxymethyl-BP (42.3 mg, 0.227 mmol) solution containing methanol (5 mL) and 4N HCl in dry dioxane (0.57 mL, 2.3 mmol) was hydrogenated in the presence of palladium/C (10 %, 40 mg) at rt and ambient pressure for 3 h. The catalyst was removed by filtration, washed with methanol (3 x 1 mL), and the combined methanolic solutions were evaporated under vacuum. The residue was dissolved in water (2 mL), Na2CO3 was added (0.1 g) and the solution was extracted with CH2Cl2 (3x2 mL). The combined CH2Cl2 solutions were dried (MgSO4) and rotary-evaporated at 2 mm and 45 °C, giving pure 3-methyl-BP (29.4 mg, 76 %) as a colorless oil.
ACKNOWLEDGEMENTS
We thank Eric Kem and Robert Bosien for their assistance in collecting the Amphiporus angulatus at numerous low tides, often in harsh weather and dim light. We are also grateful to Roy King and James Duncan (both UF) for obtaining the initial direct probe introduction EI mass spectra and high resolution mass determinations of the methyl-bipyridyl natural product. Barbara Seymour provided the nemertine drawing for the graphical abstract and Anne Rouchaud assisted in the preparation of the figures. This research was supported by Florida SeaGrants R/RL-MB-9, MB-16 and MB-20 to the Kem lab and NIDDK/NIH intramural funds to the Daly lab.
References
1. a) W. R. Kem, Toxicon, 1971, 9, 23; CrossRef b) W. R. Kem, “Neurotoxicology Handbook: Natural Toxins of Animal Origin: Nemertine Neurotoxins,” Vol. 2, ed. by A. Harvey, Humana Press, N. J. Totowa, 2001, pp. 573-593.
2. a) W. R. Kem, B. C. Abbott, and R. M. Coates, Toxicon, 1971, 9, 15; CrossRef b) X. M Bacq, Bull. Acad. R. Belg. Cl. Sci.(ser. 5), 1936, 22, 1072.
3. W. R. Kem, V. M. Mahnir, R. L. Papke, and C. Lingle, J. Pharmacol. Exper. Therap., 1997, 283, 979.
4. a) H. Kitagawa, T. Takenouchi, R. Azuma, K. A. Wesnes, W. G. Kramer, D. E. Clody, and A. L. Burnett, Neuropsychopharmacology, 2003, 28, 542; CrossRef b) A. Olincy, J. G. Harris, L. L. Johnson, V. Pender, S. Kongs, D. Allensworth, J. Ellis, G. O. Zerbe, S. Leonard, K. E. Stevens, S. O. Stevens, L. Martin, L. E. Adler, F. Soti, W. R. Kem, and R. Freedman, Arch. Gen. Psychiat., 2006, 63, 630; CrossRef c) R. Freedman, A. Olincy, R. W. Buchanan, J. G. Harris, J. M. Gold, L. Johnson, D. Allensworth, A. Guzman-Bonilla, B. Clement, M. P. Ball, J. Kutnick, V. Pender, L. F. Marin, K. E. Stevens, B. D. Wagner, G. O. Zerbe, F. Soti, and W. R. Kem, Amer. J. Psychiat., 2008, 165, 1040. CrossRef
5. W. R. Kem, K. N. Scott, and J. H. Duncan, Experientia, 1976, 32, 684. CrossRef
6. M. P. Cruskie, Jr., J. A. Zoltewicz, and K. A. Abboud, J. Org. Chem., 1995, 60, 7491. CrossRef
7. a) R. D. Bowden, Brit. Pat,, 1969, , 1,268,192 (Chem. Abstr., 1972, 76, 153605); b) R. D. Bowden, Pyridines and bipyridines, Ger. Offen, 1969, , 1,913,732 (Chem. Abstr., 1970, 72, 132535); c) R. L. Frank and J. V. Crawford, Bull. Soc. Chim. Fr., 1958, 419; d) J. K. Stille, Angew. Chem., Int. Ed. Eng., 1986, 25, 508; CrossRef e) A. Suzuki, Pure Appl. Chem., 1984, 66, 213; CrossRef f) M. Terashima and M. Ishikura, Adv. Het. Chem., 1989, 46, 143; CrossRef g) Y. Yamamoto and A. Yanagi, Chem. Pharm. Bull., 1982, 30, 1731; h) H. Sakuma, M. Kusama, K. Yamaguchi, T. Matsuki, and S. Sugawara, Beitr. Tabakforsch. Int., 1984, 22, 199, (Chem. Abstr., 1984, 101, 207812); i) M. Ishikura, M. Kamada, and M. Terashima, Synthesis, 1984; k) L. M. Bloom, Ph. D. Thesis., University of Florida, 1990.
8. a) A. Orekhov and G. Menschikov, Ber. Dtsch. Chem. Ges., 1931, 64, 266; CrossRef b) C. R. Smith, J. Am. Chem. Soc., 1935, 57, 959; CrossRef c) E. Leete, J. Am. Chem. Soc., 1969, 91, 1697. CrossRef
9. a) J. W. Wheeler, O. Olubajo, C. B. Storm, and R. M. Duffield, Science, 1981, 211, 1051; CrossRef b) N. De Kimpe, M. Keppens, and G. Fonck, Chem. Commun., 1996, 635. CrossRef
10. a) E. Spath and F. Kesztler, Ber. Dtsch. Chem. Ges., 1937, 70, 239; CrossRef b) E. Wada, T. Kisaki, and M. Ihida, Arch. Biochem., 1959, 80, 258. CrossRef
11. E. Spath and L. Mamoli, Ber. Dtsch. Chem. Ges., 1936, 69, 1082. CrossRef
12. a) Z. H. Skraup and G. Vortmann, Monatsh. Chem., 1882, 3, 572; b) C. R. Smith, J. Am. Chem. Soc., 1930, 52, 397; CrossRef c) R. Lukes and V. Pliml, Chem. Listy, 1955, 49, 1836.
13. L. M. Bloom, Ph. D. Thesis, University of Florida, 1990.
14. E. Leete, J. Am. Chem. Soc., 1969, 91, 1697. CrossRef
15. L. A. Summers, ‘Advances Heterocyclic Chemistry,’ 35, pp. 282-374, Academic Press, New York, 1984.
16. P. Krumholz, J. Am. Chem. Soc., 1951, 73, 3487. CrossRef
17. E. Leete, J. Chem. Soc., Chem. Commun., 1978, 610. CrossRef
18. a) A. H. Warfield, W. D. Galloway, and A. G. Kallianos, Phytochem, 1972, 11, 3371; CrossRef b) S. Matsushima, T. Ohsumi, and S. Sugawara, Biol. Chem., 1983, 47, 507.
19. W. R. Kem and F. Soti, Hydrobiologia, 2001, 456, 221. CrossRef
20. W. R. Kem, F. Soti, and D. Rittschof, Biomol. Engr., 2003, 20, 355. CrossRef
21. H. Hatt and I. Schmiedel-Jacob, J. Comp. Physiol. A, 1984, 154, 855. CrossRef