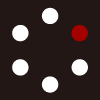
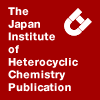
HETEROCYCLES
An International Journal for Reviews and Communications in Heterocyclic ChemistryWeb Edition ISSN: 1881-0942
Published online by The Japan Institute of Heterocyclic Chemistry
e-Journal
Full Text HTML
Received, 16th November, 2008, Accepted, 29th January, 2009, Published online, 2nd February, 2009.
DOI: 10.3987/COM-08-11603
■ Tetrahydropyrimidine Derivatives as Efficient Organic Reductants for Transfer Hydrogenation
Yongbin Zhang, Guofu Zhou, and Wei Guo*
School of Chemistry and Chemical Engineering, Shanxi University, Taiyuan 030006, China
Abstract
Two six-membered heterocyclic organic reductants, 1-acetyl-2,3-dimethyltetrahydropyrimidine (ADMP) and 1-tosyl-2,3-dimethyltetrahydropyrimidine (TDMP), were designed and synthesized for transfer hydrogenation. It was shown that both the two reductants could directly reduce a variety of aldehydes and imines to the corresponding saturated products in good yields.The investigations on heterocycles having hydrogen-donating ability as practical reductants have attracted attention in organic chemistry in recent years due to their good chemoselectivity, mild reaction condition, easily asymmetric modification as well as transition metal-free property which is especially important in medicinal area.1-23 Because 1,4-dihydropyridines are known to be the active part of the reduced form of the nicotinamide adenine dinucleotide (NADH), which plays a vital role in many biological reductions,1 1-benzyl-1,4-dihydronicotinamide, Hantzsch 1,4-dihydropyridine, 10-methyl-9,10-dihydroacridine, and many other 1,4-dihydropyridine derivatives have been extensively used as models to mimic the function of NADH, and indeed showed to have excellent reducibility in some reducing reactions.2-14 In addition to 1,4-dihydropyridine derivatives, some attention has also been paid to five-membered heterocycles, such as 2-phenylbenzimidazoline (PBI) and its derivative 1,3-dimethyl-2-phenylbenzimidazoline (DMBI).
Twenty years ago, Chikashita and coworkers reported that DMBI and PBI could selectively reduce C-C double bonds conjugated with strong electron-withdrawing groups (CN, NO2, COMe)15-17 to the corresponding saturated compounds. Thereafter, this group reported that DMBI could reduce a series of α-halocarbonyl compounds to their corresponding aldehydes or ketones without affecting the carbonyl group.18 Also, DMBI or its analog were used as reductant in photoinduced electron transfer (PET) reaction systems for the reduction of α,β-epoxy ketone, aromatic ketones, etc.19-23 However, subsequent activities have been carried out to focus on the mechanistic details of these systems,24-32 little attention has been paid in organic synthesis.
Recently, we developed a mild, efficient, and selective five-membered heterocyclic organic reductant, 1-acetyl-2,3-dimethylimidazolidine (ADMI), for the reduction of aromatic, aliphatic and α,β-unsaturated aldehydes as well as imines in good yields.33 In the present work, we wish to report two six-membered heterocyclic organic reductants, 1-acetyl-2,3-dimethyltetrahydropyrimidine (ADMP) and 1-tosyl- 2,3-dimethyltetrahydropyrimidine (TDMP), and these successful applications in transfer hydrogenation reaction.
Recently, we described ADMI as a five-membered heterocyclic compound showing excellent reactivity for reducing a series of aldehydes and imines.33 The deuterated experiment demonstrated that the reduction involving the transfer of a hydrogen atom to substrates from C-2 position of ADMI. A plausible mechanism is that the 1,3-diaza-type structure in ADMI possibly allows a hyperconjugative interaction between nonbonding tertiary amine lone pair and the σ* (C2-H) orbital (expressed by resonance between the canonical structure A-A′, Scheme 3), thus, induces a weakening of the C2-H bond and an increase of negative charge density at the hydrogen atom.32 In fact, similar structural feature is also found in the structure of PBI, DMBI as well as a 1,3,2-diazaphospholene compound.34 Although the detailed mechanism is unclear at present, the results have prompted us to exploit new 1,3-diaza-type heterocycles, such as six-membered heterocycles ADMP and TDMP, for their application in organic synthesis.
ADMP could be readily prepared in a three-step procedure (eq-1) involving (i) acetylation of the starting material, 2-methyl tetrahydropyrimidine (1), with Ac2O to 2, and (ii) methylation of 2 with CH3I to 3 and (iii) subsequent reduction with NaBH4 to the objective compound. TDMP was synthesized by the same procedure except that TsCl was used in the first step (eq-2).
Subsequently, the potential reduction ability of ADMP and TDMP was tried with 4-nitrobenzaldehyde as substrate in our standard conditions (reductant: 1 mmol, substrate: 1 mmol, Mg(ClO4)2: 0.1 mmol, MeCN-MeOH: 1:1, 4 mL).33 As expected, both the two reductants showed good reactivity at room temperature. The reactions could be completed within 12 hr and 4-nitrobenzyl alcohol was obtained in 85% and 83% yields for ADMP and TDMP, respectively. It is interesting to note that when the temperature was raised to 50 oC, the reactions could be completed after 0.5 hr with the improved yields of 90% and 92% for ADMP and TDMP, respectively. The results suggest that the reaction rate of this type of reductive reaction is sensitive to temperature, and ADMP and TDMP have similar reactivity in the condition.
Next, we examined the application scope with ADMP as reductant and various aldehydes as substrates, as shown Table 1. It was found that all aldehydes tested in this study, including electron-deficient, electron-rich, and aliphatic aldehydes could be efficiently reduced in good yields within 1 hr (Entry 1-6). Noteworthy is that the α, β-unsaturated aldehydes examined can be reduced to the corresponding unsaturated alcohols in high yields without any effect on the carbon-carbon double bonds (Entry 7-9), and the desired unsaturated alcohols were obtained as the sole products. The scope of this new reduction was further extended to a variety of imines. As shown in Table 2, for both N-tosyl imines and N-phenyl imines, the reductions worked well in our conditions. The low yield for N-tosyl-4-nitrobenzaldimine (Entry 1) and N-phenyl-4-nitrobenzaldimines (Entry 4) is mainly due to their quick decomposition in reaction conditions. In addition, similar case could also be found when TDMP as reductant. However, no effort was made to separate these reducing products, and only TLC was used to evaluate its reactivity toward various substrates.
In summary, we have developed two efficient six-membered heterocyclic organic reductants,
1-acetyl-2,3-dimethylpyrimidine (ADMP) and 1-Ts-2,3-dimethylpyrimidine (TDMP), for the reduction
of aromatic, aliphatic and α,β-unsaturated aldehydes as well as imines in good yields. Investigation to understand the reducing mechanism and to evaluate the process with a broader scope of substrates are in progress in our lab.
EXPERIMENTAL
Commercially available compounds were used without further purification. Solvents were dried according to standard procedures. All reactions were magnetically stirred and monitored by thin-layer chromatography (TLC) using Huanghai GF254 silica gel coated plates. Flash chromatography (FC) was carried out using silica gel 60 (230-400 mesh). The 1H NMR and 13C NMR spectra were recorded at 300 and 75MHz, respectively. The following abbreviations were used to explain the multiplicities: s = singlet; d = doublet; t = triplet; q = quartet; m = multiplet; br = broad. MS spectra were obtained on a JMS-D300 GC/MS spectrometer. IR spectra were obtained on a Shimadzu IR-1700 spectrophotometer. Melting points were uncorrected.
Synthesis of 1-acetyl-2,3-dimethyltetrahydropyrimidine (ADMP)
2-Methyltetrahydropyrimidine (0.98 g, 10 mmol) was dissolved in 15 mL dry CH2Cl2 at 0 oC, followed by the addition of triethylamine (1.01 g, 10 mmol). Then this solution was added dropwise a solution of acetic anhydride (1.02 g, 10 mmol) in 5 mL CH2Cl2. After the addition, the reaction mixture was allowed to rt for an additional 3 h. The resulted solution was treated with 10% aqueous NaHCO3 (10 mL). After stirring 0.5 h, the mixture was extracted with CH2Cl2, and the organic layers were washed with the saturated brine, and dried over anhydrous sodium sulfate. Evaporation of CH2Cl2 in vacuo gave 0.96 g of 1-acetyl-2-methyltetrahydropyrimidine (2a) as an oil (yield: 69%).
1-Acetyl-2-methyltetrahydropyrimidine (0.96 g, 6.9 mmol) and iodomethane (1.3 mL, 20.7 mmol) were stirred in a sealed flask for 5 h in rt. Then 2 mL of acetone was added, the mixture was stirred for a few minutes, and the solid obtained was collected by vacuum filtration to give 1.36 g of 1-acetyl-2,3-dimethyltetrahydropyrimidinium iodide (3a) as a white solid (yield: 70%). 1H NMR (300 MHz, δ ppm, CDCl3): 2.38 (m, 2H), 2.54 (s, 3H), 2.75 (s, 3H), 3.50 (s, 3H), 3.86 (m, 2H), 4.14 (t, J = 5.70, 2H).
1-Acetyl-2,3-dimethyltetrahydropyrimidinium iodide (0.85 g, 3 mmol) was dissolved in 10 mL of MeCN at 0 oC, followed by the addition of NaBH4 (0.13 g, 3.3 mmol). After the addition, the reaction mixture was allowed to rt for an additional 5 h. The resulted solution was treated with 50 mL of water. The mixture was extracted with CH2Cl2, and the organic layers were washed with the saturated brine, and dried over anhydrous sodium sulfate. Evaporation of dichloromethane in vacuo gave 0.43 g of 1-Acetyl-2,3-dimethyltetrahydropyrimidine (ADMP) as a clear oil (yield: 91%). 1H NMR (300 MHz, δ ppm, D2O): 1.29−1.44 (2×m, 5H), 1.82−1.92 (2×s, 3H), 2.36−2.45 (2×s, 3H), 2.65 and 3.58 (2×m, 2H), 3.21 and 4.14 (2×m, 2H), 4.53 and 5.01 (2×m, 1H). IR (film) cm-1: 2960, 2381, 1635, 1427, 1369, 1296, 1072. MS m/z: 157 ([M+1]+). Anal. Calcd for C8H16N2O: C, 61.50; H, 10.32; N, 17.93. Found C, 61.34; H, 10.21, N, 17.85.
Synthesis of 1-tosyl-2,3-dimethyltetrahydropyrimidine (TDMP)
2-Methyltetrahydropyrimidine (0.98 g, 10 mmol) was dissolved in 15 mL dry CH2Cl2 at 0 oC, followed by the addition of triethylamine (1.01 g, 10 mmol). Then this solution was added dropwise a solution of TsCl (1.905 g, 10 mmol) in 10 mL CH2Cl2. After the addition, the reaction mixture was allowed to rt for an additional 3 h. The resulted solution was treated with 10% NaHCO3 (10 mL). After stirring 0.5 h, the mixture was extracted with CH2Cl2, and the organic layers were washed with the saturated brine, and dried over anhydrous sodium sulfate. Evaporation of CH2Cl2 in vacuo gave 1.27 g of 1-tosyl-2-methyltetrahydropyrimidine (2b) as an oil (yield: 50%).
1-Tosyl-2-methytetrahydropyrimidine (1.27 g, 5 mmol) and iodomethane (0.94 mL, 15 mmol) were refluxed in Et2O (10 mL) for 8 h. After cooled to rt, 1 mL of acetone was added. The mixture was stirred for several minutes, and the solid obtained was collected by vacuum filtration to give 1.38 g of 1-tosyl-2,3-dimethyltetrahydropyrimidinium iodide (3b) as a white solid (yield: 70%). 1H NMR (300 MHz, δ ppm, CDCl3): 2.33 (m, 2H), 2.44 (s, 3H), 2.71 (s, 3H), 3.45 (s, 3H), 3.85 (m, 2H), 4.30 (m, 2H), 7.44 (d, J = 7.89, 2H), 7.91 (d, J = 7.92, 2H).
1-Tosyl-2,3-dimethyltetrahydropyrimidinium iodide (1.18 g, 3 mmol) was dissolved in 10 mL of MeCN at 0 oC, followed by the addition of NaBH4 (0.13 g, 3.3 mmol). After the addition, the reaction mixture was allowed to rt for an additional 5 h. The resulted solution was treated with 50 mL of water. The mixture was extracted with CH2Cl2, and the organic layers were washed with the saturated brine, and dried over anhydrous sodium sulfate. Evaporation of CH2Cl2 in vacuo gave 0.76 g of 1-tosyl-2,3-dimethyltetrahydropyrimidine (TDMP) as a clear oil (yield: 95%). 1H NMR (300 MHz, δ ppm, CDCl3): 1.33 (d, J = 6.54, 3H), 1.54 (m, 1H), 2.05 (m, 1H), 2.40 (s, 3H), 2.77 (s, 3H), 2.83 (m, 1H), 2.96 (t, J = 12.96, 1H), 3.24 (t, J = 12.69, 1H), 3.75 (m, 1H), 4.74 (m, 1H), 7.28 (d, J = 7.74, 2H), 7.60 (d, J = 7.86, 2H). IR (film) cm-1: 2956, 2380, 1596, 1450, 1384, 1338, 1157. MS m/z: 269 ([M+1]+). Anal. Calcd for C13H20N2O2S: C, 58.18; H, 7.51; N, 10.44. Found C, 58.24; H, 7.25, N, 10.25.
General procedure for the transfer hydrogenation reaction with ADMP and TDMP as reductants
To a solution of substrate (1 mmol) and Mg(ClO4)2 (22.3 mg, 0.1 mmol) in MeOH (2 mL) was added a solution of reductant (1 mmol) in 2 mL of anhydrous MeCN. The reaction mixture was stirred at 50 oC and monitored by TLC. Upon completion, the solvent was removed in vacuo and the residue was chromatographed on silica gel to give the desired products.
ACKNOWLEDGEMENTS
We thank the National Natural Science Foundation of China (No. 20502014 and 20772073) for financial support.
References
1. L. Stryer, Biochemistry, 3rd ed., Freeman: New York, 1998; Chapters 15-18.
2. D. D. Tanner and A. R. Stein, J. Org. Chem., 1988, 53, 1642. CrossRef
3. C. A. Coleman, J. G. Rose, and C. H. Murray, J. Am. Chem. Soc., 1992, 114, 9755. CrossRef
4. M. Rueping, E. Sugiono, C. Azap, T. Theissmann, and M. Bolte, Org. Lett., 2005, 7, 3781. CrossRef
5. J. W. Yang, M. T. Hechavarria, and B. List, Angew. Chem. Int. Ed., 2004, 43, 6660. CrossRef
6. S. G. Ouellet, J. B. Yuttle, and D. W. C. MacMillan, J. Am. Chem. Soc., 2005, 127, 32. CrossRef
7. J. W. Yang, M. T. Hechavarria, N. Vignola, and B. List, Angew. Chem. Int. Ed., 2005, 44, 108. CrossRef
8. S. Hoffmann, A. M. Seayad, and B. List, Angew. Chem. Int. Ed., 2005, 44, 7424. CrossRef
9. J. B. Tuttle, S. G. Ouellet, and D. W. C. MacMillan, J. Am. Chem. Soc., 2006, 128, 12662. CrossRef
10. K. Miyashita, M. Nishimoto, H. Murafuji, A. Murakami, S. Obika, Y. In, T. Ishida and T. Imanishi, Chem. Commun., 1996, 22, 2535. CrossRef
11. N. Kanomata and T. Nakata, Angew. Chem., Int. Ed. Engl., 1997, 36, 1207. CrossRef
12. X.-Q. Zhu, Y.-C. Liu, and J.-P. Cheng, Cheng, J. Org. Chem., 1999, 64, 8980. CrossRef
13. X.-Q. Zhu, Y. Liu, B.-J. Zhao, and J.-P. Cheng, J. Org. Chem., 2001, 66, 370. CrossRef
14. X.-Q. Zhu, Y. Yang, M. Zhang, and J.-P. Cheng, J. Am. Chem. Soc., 2003, 125, 15298. CrossRef
15. H. Chikashita, S. Nishida, M. Miyazaki, and K. Itoh, Synth. Commun., 1983, 13, 1033. CrossRef
16. H. Chikashita, Y. Morita, and K. Itoh, Synth. Commun., 1985, 15, 527. CrossRef
17. H. Chikashita and K. Itoh, Bull. Chem. Soc. Jpn., 1986, 59, 1747. CrossRef
18. H. Chikashita, H. Ide, and K. Itoh, J. Org. Chem., 1986, 51, 5400. CrossRef
19. E. Hasegawa, A. Yoneoka, K. Suzuki, T. Kato, T. Kitazume, and K. Yanagi, Tetrahedron, 1999, 55, 12957. CrossRef
20. E. Hasegawa, T. Seida, N. hiba, T. Takahashi, and H. Ikeda, J. Org. Chem., 2005, 70, 9632. CrossRef
21. E. Hasegawa, N. Chiba, T. Takahashi, S. Takizawa, T. Kitayama, and T. Suzuki, Chem. Lett., 2004, 33, 18. CrossRef
22. E. Hasegawa, S. Takizawa, T. Seida, A. Yamaguchi, N. Yamaguchi, N. Chiba, T. Takahashi, H. Ikeda and K. Akiyama, Tetrahedron, 2006, 62, 6581. CrossRef
23. E. Hasegawa, N. Chiba, A. Nakajima, K. Suzuki, A. Yoneoka, and K. Iwaya, Synthesis, 2001, 1248. CrossRef
24. D. D. Tanner and J. J. Chen, J. Org. Chem., 1992, 57, 662. CrossRef
25. I. H. Lee, E. H. Heoung, and M. M. Kreevoy, J. Am. Chem. Soc., 1997, 119, 2722. CrossRef
26. I. H. Lee and E. H. Heoung, J. Org. Chem., 1998, 63, 7275. CrossRef
27. I. H. Lee, E. H. Heoung, and M. M. Kreevoy, J. Am. Chem. Soc., 2001, 123, 7492. CrossRef
28. I. H. Lee, Y. R. JI, and E. H. Heoung, J. Phys. Chem. A, 2006, 110, 3875. CrossRef
29. S. M. Ramos, M. Tarazi, and J. D. Wuest, J. Org. Chem., 1987, 52, 5437. CrossRef
30. B. Bachand, S. M. Ramos, and J. D. Wuest, J. Org. Chem., 1987, 52, 5443. CrossRef
31. F. Montgrain, S. M. Ramos, and J. D. Wuest, J. Org. Chem., 1988, 53, 1489. CrossRef
32. X.-Q. Zhu, M.-T. Zhang, A. Yu, C.-P. Cheng, J. Am. Chem. Soc., 2008, 130, 2501. CrossRef
33. D. Li, Y. Zhang, G. Zhou, and W. Guo, Synlett, 2008, 225. CrossRef
34. S. Burck, D. Gudat, M. Nieger, and W. Du Mont, J. Am. Chem. Soc., 2006, 128, 3946. CrossRef