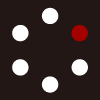
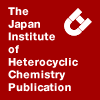
HETEROCYCLES
An International Journal for Reviews and Communications in Heterocyclic ChemistryWeb Edition ISSN: 1881-0942
Published online by The Japan Institute of Heterocyclic Chemistry
e-Journal
Full Text HTML
Received, 25th November, 2008, Accepted, 18th December, 2008, Published online, 25th December, 2008.
DOI: 10.3987/COM-08-11609
■ Novel Charge-Transfer Chromophores Featuring Imidazole as π-Linkage
Anjan Patel, Filip Bureš,* Miroslav Ludwig, Jiří Kulhánek, Oldřich Pytela, and Aleš Růžička
Department of Organic Chemistry, Faculty of Chemical Technology, University of Pardubice, nám. Čs. legií 565, Pardubice, CZ-532 10, Czech Republic
Abstract
Overall 21 imidazole-based chromophores have been synthesized and fully characterized. The imidazole core was systematically substituted with donors (NMe2 and OMe) and acceptors (NO2 and CN groups) additionally separated from the imidazole by π-spacers such as 1,4-phenylen, styryl or thiophen-2-yl in order to finely tune their intramolecular charge transition. The target chromophores were further investigated by UV/Vis spectroscopy as potentially NLO-active compounds.INTRODUCTION
Organic nonlinear optical (NLO) materials have been drawing considerable attention over the last two decades due to their potential applications as an optical data storage medium or in switching or modulating devices.1 Many diverse organic push-pull molecules have already been developed, synthesized and investigated in this context. It is already well known that the second- and third-order optical nonlinearity of organic D-π-A, D-π-D and A-π-A push-pull molecules depends on the length of the planar π-conjugated spacer and the nature of the electron-donating (D) and electron-withdrawing (A) groups attached.2 The representative organic chromophores most commonly consist of a π-conjugated backbone with the attached strong acceptors such as nitro (NO2) or cyano (CN) groups and strong donors as dialkylamino (R2N) or alkoxy (RO) groups. Six-membered aromatic rings (benzene and its polynuclear
derivatives),3 six-membered heteroaromatic rings (pyridine analogues),4 polyenes and polyynes or their mix5 are the most frequently used π-conjugate backbone in such materials to achieve an efficient intramolecular charge transfer from the donor to the acceptor and simultaneously the large molecular nonlinear optical polarizabilities.
In spite of the extensive use of the above-mentioned chromophores as NLO active molecules, these compounds frequently suffer from chemical and thermal lability and tedious and expensive synthesis. Hence, we turned our attention to the heterocyclic chromophores possessing a five-membered imidazole ring as a π-conjugate backbone. The imidazoles are generally easy to synthesize from inexpensive and readily available starting material, offer manifold functionalization in terms of attaching various electron-donors and electron-acceptors and also possess good thermal robustness. Various donor-acceptor substituted imidazoles have already been investigated, mainly by Moylan and co-workers,6 while some triarylimidazole7 and benzimidazole8 push-pull systems are known as well. The general molecular structure of our proposed imidazole-derived chromophores is shown in Figure 1. We have synthesized and further investigated five series of the donor-acceptor substituted imidazoles (see Table 1 and 2 below for more details). Whereas the series 1 and 2 consist of A-π-D and D-π-A push pull systems varying in the length of the π-linkage (2,4,5-triphenyl-1H-imidazole or 2-styryl-4,5-diphenyl-1H-imidazole) and the donors (NMe2, OMe, and Me) and acceptors (NO2 and CN) attached; series 3 and 4 represent A-π-A and D-π-D triphenyl-1H-imidazole chromophores, respectively. Since the thiophene ring has already been established as a useful additional π-linkage and could perform also as an auxiliary electron-donor,9 series 5 is achieved by the introduction of the thiophene-linkage at the C2 position of the imidazole (2-(thiophen-2-yl)-4,5-bis(4-nitrophenyl)-1H-imidazole).
RESULTS AND DISCUSSION
Synthesis of chromophores: With respect to an eventual application of the proposed molecules as NLO-active chromophores, the synthesis of such compounds should be facile, scalable and start from readily available materials with easy functionalization and work-up. Hence, our retro-synthetic strategy leading to target chromophores 1-5 involves facile and one-pot straightforward condensation of 4,4’-disubstituted benzils with various aldehydes in the presence of ammonium acetate in glacial acetic acid - modified Radziszewski synthesis developed for the synthesis of 2,4,5-triphenylimidazole (Lophin, Scheme 1, Table 1).10 Either donor-substituted 4,4’-bis(N,N-dimethylamino)benzil and 4,4’-dimethoxybenzil or acceptor-substituted 4,4’-dinitrobenzil were used as starting 1,2-diketones. Whereas the first two benzils were commercially available, the latter was prepared from benzoin acetate using the method described by Cremlyn11 (see the Experimental for the full spectral characterization) Due to a low-quality of the published X-ray analysis of 4,4’-dinitrobenzil,12 Figure 2 shows the improved ORTEP plot including hydrogens (for more details see Experimental). An initial condensation of the 4,4’-dinitrobenzil with formaldehyde under the above-specified conditions afforded C2-unsubstituted imidazole 6 in 75% yield (see Figure 2 for the ORTEP plot). The X-ray analysis of 6 showed that, whereas one of the 4-nitrophenyl rings is almost coplanar with the residual imidazole (13°), the latter is strongly twisted out of the imidazole plane by 45°.
With this result in hand, we proceeded to the condensation of either donor- or acceptor-substituted benzaldehydes (n = 0) and trans-4-substitutedcinnamaldehydes (n = 1) with the above benzils. The optimized reaction was carried out with 10 equivalents of ammonium acetate at 120 °C and monitoring on a TLC. Following this procedure, we have synthesized seven A-π-D chromophores with nitro groups as acceptors (series 1), seven D-π-A chromophores featuring either N,N-dimethylamino or methoxy groups as donors (series 2), one A-π-A chromophore with all nitro groups (3) and two D-π-D chromophores with all N,N-dimethylamino or methoxy groups (series 4). Chromophores 2a,9b,13 2d,6a,14 2g,14 3,15 4a,16 4b,17 and 613 are already known compounds, however, to the best of our knowledge, only compounds 2a and 2d were investigated as potentially NLO-active chromophores.6a,7b-c
It should be noted here that all of our synthetic attempts to apply the Radziszewski synthesis on 4,4’-dicyanobenzil18 failed. Although the reactions of the 4,4’-dinitro- or 4,4’-dimethoxybenzils with trans-4-nitro-, trans-4-(N,N-dimethylamino)- and trans-4-methoxycinnamaldehydes were sluggish, target chromophore 1b, 1d, and 2e with an additional trans-ethenyl spacer could be isolated in the yields 55, 52, and 61%, respectively. On the other hand, the reaction of 4,4’-bis(N,N-dimethylamino)benzil with trans-4-nitrocinnamaldehyde afforded only an inseparable mixture of several products where the target chromophore was not even detected by an ESI-MS.
In view of the recent reports on the use of thiophene as an auxiliary electron-donor,9 we were also interested in a similar condensation of the commercially available thiophene-2-carbaldehydes as a donor-moiety and 4,4´-dinitrobenzil as an acceptor molecule part. Thus, the last series of chromophores 5 was synthesized in the same way as described for the substituted benzaldehydes and cinnamaldehydes affording similar yields of 54-60% (Scheme 2, Table 2).
UV/Vis spectroscopy: Since the prepared push-pull chromophores 1-5 are overall colored compounds (see Table 1 and 2), their absorption properties were investigated in dichloromethane. The presence of an electron-donor substituent on the imidazole positions 4 and 5 (series 2 and 4) led to a red-shift which depends mainly on the difference between the donors and the acceptors on the 2-phenyl ring (compare λmax for series 4a-2c-2a, and 4b-2g-2d). This is presumably caused due to a better electron delocalization as a result of the direct conjugation known for such push-pull systems.
In the case of the electron-acceptor substituents on the imidazole positions 4 and 5 (series 1 and 3), the structural situation is opposite. The bathochromic shift increases with the rising strength of the appended 2-phenyl donors (compare λmax for series 1a-1c-1f) whereas now the ability of the electron-deficient imidazole molecule part to provide electrons into the conjugation with the 2-phenyl is suppressed by the acceptor-substitution at positions 4 and 5. The combination of donors on both molecule moieties (4a and 4b) caused an electron-enrichment of the imidazole system and, therefore, the higher donating ability of the N,N-dimethylamino groups (4a) resulted in the stronger bathochromic shift than that measured for methoxy-substituted 4b.
The combination of strong acceptors on all imidazole positions (3) led also to the bathochromic shift. This implies that the polarization and, consequently, the spectral behavior of the studied chromophores are mainly affected by the substitution on the 2-phenyl moiety. The imidazole molecule part in such chromophores performs as an electron-donor whereas its donating effect may be further enhanced by the donor-substitution at positions 4 and 5. Thus, the chromophore polarization from the imidazole to the 2-phenyl molecule part seems to be crucial. The observed position of the longest-wavelength bands for the 2- and 4-substituted phenyls (1f vs. 1g, 2a vs. 2b and 2d vs. 2f, respectively) revealed a strong steric effect of the branched and rigid nitro group as compared with the less bulky methyl group. This results in a strong twist of the nitro group from the molecule plane and engagement of the nitro group in less conjugation while a strong hypsochromic shift was observed.
At first sight, the UV/Vis spectra of the chromophores with trans-ethenyl spacer seemed to be inconsistent. However, the combination of the nitro and methoxy substituents (1d vs. 1c and 2e vs. 2d) led to the bathochromic shift which is especially remarkable for 1d vs. 1c (31 nm). This is presumably caused due to a better conjugation in such a system (larger for NO2 group) which significantly affects the transition between the imidazole and trans-ethenyl bridge of the chromophore. Substitution of the 2-phenyl with the strong N,N-dimethylamino donor (1b vs. 1a) led presumably to a significant conjugation between the N,N-dimethylamino substituent and the trans-ethenyl bridge and, consequently, the entire 4-N,N-dimethylaminostyryl substituent at position 2 behaved as an electron-donor. Thus, the observed strong hypsochromic shift (96 nm) is in accordance with the above discussion.
The spectra of the chromophore series 5a-c showed CT bands appearing between 362 and 363 nm (slightly red-shifted compared with 1f). As expected, the most bathochromic shift was measured for the 4-methoxyphenylthiophene substituted imidazole 5d. It should be noted here that 5d showed even better transition than similar 4-methoxyphenyl substituted chromophore 1c. This fact further demonstrates that an elongation of the planar π-conjugated spacer between the donor and acceptor leads to the highest transition and, simultaneously, to the highest molecular polarizability (compare 1c-5d-1d).
Preliminary calculations of the average second-order polarizabilities β using MOPAC19 program confirmed the conclusion made from the UV/Vis spectroscopy while the highest values were calculated for the chromophores with strong NO2-acceptors and NMe2-donors attached and bearing longer π-conjugated spacer (additional trans-ethenyl or thiophen-2-yl π-linkages). A detailed account on the investigation of NLO-properties will be published elsewhere.
In conclusion, the mentioned imidazole-based push-pull chromophores proved to be attractive candidates for device integration, particularly since many of them feature a good thermal robustness (see melting points in Tables 1 and 2) required in fabrication techniques and offer an easy chemical modification in order to finely tune the NLO-properties.
EXPERIMENTAL
Reagents and solvents were reagent-grade and were purchased from Penta, Aldrich, and Acros and used as received. Column chromatography (CC) was carried out with SiO2 60 (particle size 0.040-0.063 mm, 230-400 mesh; Merck) and commercially available solvents. Thin-layer chromatography (TLC) was conducted on Al2O3 sheets coated with SiO2 60 F254 obtained from Merck, with visualization by UV lamp (254 or 360 nm). Melting points (mp) were measured on a Büchi B-540 melting-point apparatus in open capillaries and are uncorrected. 1H- and 13C-NMR spectra were recorded in CDCl3/DMSO-d6 or acetone-d6 at 360/500 MHz and 90/125 MHz, respectively, with a Bruker AMX 360 or Bruker AVANCE 500 instrument at 25 °C. Chemical shifts are reported in ppm relative to the signal of Me4Si. The residual solvent signal in the 1H- and 13C-NMR spectra was used as an internal reference (CDCl3 – 7.25/77.23, DMSO-d6 – 2.55/39.51, and acetone-d6 – 2.05/29.92 ppm, respectively). Coupling constants (J) are given in Hz. The apparent resonance multiplicity is described as s (singlet), br s (broad singlet), d (doublet), dd (doublet of doublet), t (triplet), q (quartet) and m (multiplet). Some carbon atoms and NH protons of the target compounds were not detected by the 1H- and 13C-NMR spectroscopy, most likely due to the imidazole tautomerism (depending mainly on the solvent used).20 The mass spectra were measured either on a GC/MS configuration comprised of an Agilent Technologies – 6890N gas chromatograph (HP-5MS column, length 30 m, I.D. 0.25 mm, film 0.25 µm) equipped with a 5973 Network MS detector (EI 70 eV, mass range 33-550 Da) or on a LC-MS Micromass Quattro Micro API (Waters) instrument with a direct input (ESI+, 0.5 mL/min stream of CH3OH, mass range 200-800 Da and MassLynx software were used). UV/Vis absorption spectra were recorded using a Hewlett Packard 8453 UV/Vis spectrometer. Elemental analyses were performed on an EA 1108 Fisons instrument.
4,4´-Dinitrobenzil. The title compound was synthesized by the literature method11 in 88% yield; mp 212-214 °C, lit.11a 212-213; Rf = 0.74 (SiO2; acetone); 1H-NMR (360 MHz, DMSO-d6): δ = 8.28-8.33 (m, 4H), 8.45-8.47 (m, 4H); 13C-NMR (90 MHz, DMSO-d6): δ = 124.16, 131.90, 136.99, 151.05, 190.52; EI-MS (70 eV): m/z = 300 (M+, 1), 150 (100), 134 (46), 120 (20), 104 (53), 92 (20), 76 (39).
Condensation of substituted benzaldehydes and cinnamaldehydes with 4,4’-disubstitutedbenzils: General Procedure
4,4´-Disubstitutedbenzil (0.33 mmol), substituted benzaldehyde or cinnamaldehyde (0.33 mmol) and ammonium acetate (0.25 g, 3.33 mmol) were heated at 120 °C in glacial acetic acid (5 mL) until the TLC showed reaction completion (usually 2 to 12 h). The reaction mixture was cooled to 25 °C, poured on ice and neutralized with aqueous ammonia to pH 7.0. The precipitate was collected by filtration, washed with water and purified by column chromatography.
2-(4-N,N-Dimethylaminophenyl)-4,5-bis(4-nitrophenyl)-1H-imidazole (1a): Red solid. Yield 35%; mp 272-274 °C; Rf = 0.38 (SiO2; CH2Cl2/EtOAc 9:1); 1H-NMR (360 MHz, DMSO-d6): δ = 3.03 (s, 6H), 6.86 (d, J = 8.7 Hz, 2H), 7.83 (d, J = 8.1 Hz, 4H), 7.98 (d, J = 8.7 Hz, 2H), 8.27-8.32 (m, 4H); 13C-NMR (90 MHz, DMSO-d6): δ = 39.91, 111.89, 117.11, 124.07, 126.84, 148.86, 150.82. ESI-MS: m/z = 452 [M+ + Na], 881 [2M+ + Na]; Anal. Calcd for C23H19N5O4 (429.43): C 64.33, H 4.46, N 16.31. Found: C 64.20, H 4.77, N 15.92.
(E)-2-(4-N,N-Dimethylaminostyryl)-4,5-bis(4-nitrophenyl)-1H-imidazole (1b): Dark red solid. Yield 55%; mp 254-256 °C; Rf = 0.34 (SiO2; EtOAc/hexane 1:1); 1H-NMR (500 MHz, DMSO-d6): δ = 3.00 (s, 6H), 6.79 (d, J = 8.7 Hz, 2H), 6.89 (d, J = 16.4 Hz, 1H), 7.47-7.50 (m, 3H), 7.81 (d, J = 7.7 Hz, 4H), 8.27-8.32 (m, 4H), 12.93 (br s, 1H); 13C-NMR (125 MHz, DMSO-d6): δ = 30.73, 111.25, 112.25, 123.45, 124.10, 128.00, 128.48, 132.76, 146.22, 148.43, 150.56; ESI-MS: m/z = 478 [M+ + Na], 933 [2M+ + Na]; Anal. Calcd for C25H21N5O4 (455.47): C 65.93, H 4.65, N 15.38. Found: C 66.00, H 4.66, N 15.39.
2-(4-Methoxyphenyl)-4,5-bis(4-nitrophenyl)-1H-imidazole (1c): Orange solid. Yield 48%; mp 295-297 °C; Rf = 0.71 (SiO2; CH2Cl2/EtOAc 3:2); 1H-NMR (500 MHz, DMSO-d6): δ = 3.83 (s, 3H), 7.08 (d, J = 8.5 Hz, 2H), 7.79 (t, J = 9.0 Hz, 4H), 8.04 (d, J = 8.5 Hz, 2H), 8.20 (d, J = 9.0 Hz, 2H), 8.31 (d, J = 8.5 Hz, 2H), 13.01 (br s, 1H); 13C-NMR (125 MHz, DMSO-d6): δ = 55.24, 114.18, 122.09, 123.83, 124.07, 127.15, 127.98, 129.17, 136.81, 141.34, 145.87, 146.46, 147.78, 159.98; ESI-MS: m/z = 439 [M+ + Na], 855 [2M+ + Na]; Anal. Calcd for C22H16N4O5 (416.39): C 63.46, H 3.87, N 13.46. Found: C 63.52, H 3.97, N 13.28.
(E)-2-(4-Methoxystyryl)-4,5-bis(4-nitrophenyl)-1H-imidazole (1d): Orange solid. Yield 52%; mp 251-253 °C; Rf = 0.34 (SiO2; EtOAc/hexane 1:1); 1H-NMR (360 MHz, DMSO-d6): δ = 3.85 (s, 3H), 7.00-7.05 (m, 3H), 7.55 (d, J = 16.4 Hz, 1H), 7.62 (d, J = 8.3 Hz, 2H), 7.81 (d, J = 8.3 Hz, 4H), 8.29-8.33 (m, 4H), 13.05 (br s, 1H); 13C-NMR (90 MHz, DMSO-d6): δ = 55.23, 114.09, 114.43, 124.07, 128.21, 128.50, 128.59, 131.85, 146.25, 147.78, 159.66; ESI-MS: m/z = 465 [M+ + Na], 907 [2M+ + Na]; Anal. Calcd for C24H18N4O5 (442.42): C 65.15, H 4.10, N 12.66. Found: C 65.20, H 4.12, N 12.71.
2-(3,4,5-Trimethoxyphenyl)-4,5-bis(4-nitrophenyl)-1H-imidazole (1e): Orange solid. Yield 57%; mp 276-278 °C; Rf = 0.57 (SiO2; CH2Cl2/EtOAc 3:2); 1H-NMR (360 MHz, DMSO-d6): δ = 3.77 (s, 3H), 3.93 (s, 6H), 7.47 (s, 2H), 7.85 (d, J = 8.8 Hz, 4H), 8.30-8.34 (m, 4H); 13C-NMR (90 MHz, DMSO-d6): δ = 56.12, 60.19, 103.09, 124.11, 125.03, 138.38, 147.69, 153.26; ESI-MS: m/z = 499 [M+ + Na], 975 [2M+ + Na]; Anal. Calcd for C24H20N4O7 (476.44): C 60.50, H 4.23, N 11.76. Found: C 60.52, H 4.17, N 11.78.
2-(4-Methylphenyl)-4,5-bis(4-nitrophenyl)-1H-imidazole (1f): Yellow solid. Yield 42%; mp 279-281 °C; Rf = 0.69 (SiO2; CH2Cl2/EtOAc 9:1); 1H-NMR (360 MHz, DMSO-d6): δ = 2.41 (s, 3H), 7.37 (d, J = 8.0 Hz, 2H), 7.84 (d, J = 8.4 Hz, 4H), 8.04 (d, J = 8.0 Hz, 2H), 8.25-8.35 (m, 4H), 13.14 (br s, 1H); 13C-NMR (90 MHz, DMSO-d6): δ = 20.98, 123.97, 124.16, 125.67, 126.87, 128.12, 129.40, 129.47, 138.80, 147.92; ESI-MS: m/z = 423 [M+ + Na], 823 [2M+ + Na] ; Anal. Calcd for C22H16N4O4 (400.39): C 66.00, H 4.03, N 13.99. Found: C 66.09, H 4.07, N 13.78.
2-(2-Methylphenyl)-4,5-bis(4-nitrophenyl)-1H-imidazole (1g): Yellow solid. Yield 41%; mp 275-277 °C; Rf = 0.66 (SiO2; CH2Cl2/EtOAc 9:1); 1H-NMR (360 MHz, DMSO-d6): δ = 2.68 (s, 3H), 7.37-7.42 (m, 3H), 7.78 (d, J = 8.6 Hz, 1H), 7.85 (d, J = 8.7 Hz, 4H), 8.26-8.33 (m, 4H), 13.11 (br s, 1H). 13C-NMR (90 MHz, DMSO-d6): δ = 21.02, 124.04, 125.88, 129.00, 129.07, 129.23, 131.23, 136.65, 146.31, 148.35; ESI-MS: m/z = 423 [M+ + Na], 823 [2M+ + Na]; Anal. Calcd for C22H16N4O4 (400.39): C 66.00, H 4.03, N 13.99. Found: C 66.11, H 4.04, N 13.88.
2-(4-Nitrophenyl)-4,5-bis(4-N,N-dimethylaminophenyl)-1H-imidazole (2a): Dark red solid. Yield 55%; mp 104-106 °C; Rf = 0.52 (SiO2; CH2Cl2/EtOAc 4:1); 1H-NMR (360 MHz, acetone-d6): δ = 2.95 (s, 12H), 6.72 (d, J = 8.8 Hz, 4H), 7.46 (d, J = 8.3 Hz, 4H), 8.3 (s, 4H); 13C-NMR (90 MHz, acetone-d6): δ = 40.57, 111.88, 113.00, 124.98, 126.13, 129.57, 132.51, 137.77, 150.89; ESI-MS: m/z = 450 [M+ + Na], 877 [2M+ + Na] ; Anal. Calcd for C25H25N5O2 (427.50): C 70.24, H 5.89, N 16.38. Found: C 70.52, H 6.08, N 16.19.
2-(2-Nitrophenyl)-4,5-bis(4-N,N-dimethylaminophenyl)-1H-imidazole (2b): Red solid. Yield 60%; mp 189-191 °C; Rf = 0.27 (SiO2; CH2Cl2/EtOAc 4:1); 1H-NMR (360 MHz, DMSO-d6): δ = 2.93 (s, 6H), 2.98 (s, 6H), 6.70 (d, J = 8.0 Hz, 2H), 6.81 (d, J = 8.0 Hz, 2H), 7.38 (d, J = 7.8 Hz, 4H), 7.61 (t, J = 7.8 Hz, 1H), 7.78 (t, J = 7.8 Hz, 1H), 7.90 (d, J = 7.8 Hz, 1H), 8.01 (d, J = 7.8 Hz, 1H), 12.61 (br s, 1H); 13C-NMR (90 MHz, DMSO-d6): δ = 40.12, 112.06, 118.39, 123.30, 123.64, 123.88, 127.62, 127.86, 128.80, 128.90, 129.31, 131.84, 137.02, 139.54, 148.18, 149.07, 149.69; ESI-MS: m/z = 450 [M+ + Na], 877 [2M+ + Na]; Anal. Calcd for C25H25N5O2 (427.50): C 70.24, H 5.89, N 16.38. Found: C 70.27, H 6.01, N 16.48.
2-(4-Cyanophenyl)-4,5-bis(4-N,N-dimethylaminophenyl)-1H-imidazole (2c): Orange solid. Yield 71%; mp 124-126 °C; Rf = 0.59 (SiO2; CH2Cl2/EtOAc 4:1); 1H-NMR (360 MHz, acetone-d6): δ = 3.44 (s, 12H), 7.20 (d, J = 8.8 Hz, 4H), 7.95 (d, J = 8.7 Hz, 4H), 8.27 (d, J = 8.6 Hz, 2H), 8.72 (d, J = 8.6 Hz, 2H). 13C-NMR (90 MHz, DMSO-d6): δ = 50.16, 120.73, 122.56, 129.25, 135.72, 139.14, 142.95, 145.43, 153.22, 160.35; ESI-MS: m/z = 430 [M+ + Na], 837 [2M+ + Na]; Anal. Calcd for C26H25N5 (407.51): C 76.63, H 6.18, N 17.19. Found: C 76.43, H 6.11, N 17.28.
2-(4-Nitrophenyl)-4,5-bis(4-methoxyphenyl)-1H-imidazole (2d): Orange solid. Yield 91%, mp 217-219 °C, lit.,14 217-218 °C; Rf = 0.53 (SiO2; CH2Cl2/EtOAc 9:1); 1H-NMR (360 MHz, acetone-d6): δ = 3.81 (s, 6H), 6.92 (d, J = 8.5 Hz, 4H), 7.50 (d, J = 8.5 Hz, 4H), 8.26-8.28 (m, 4H); 13C-NMR (90 MHz, acetone-d6): δ = 55.63, 114.74, 124.95, 126.35, 130.10, 137.42, 143.94, 147.81, 160.19; ESI-MS: m/z = 424 [M+ + Na], 825 [2M+ + Na]; Anal. Calcd for C23H19N3O4 (401.41): C 68.82, H 4.77, N 10.47. Found: C 69.04, H 4.92, N 10.49.
(E)-2-(4-Nitrostyryl)-4,5-bis(4-methoxyphenyl)-1H-imidazole (2e): Red solid. Yield 61%; mp 101-103 °C; Rf = 0.28 (SiO2; EtOAc/hexane 1:1); 1H-NMR (360 MHz, acetone-d6): δ = 3.82 (s, 6H), 6.90-6.98 (m, 4H), 7.30 (d, J = 16.4 Hz, 1H), 7.43-7.56 (m, 5H), 7.79 (d. J = 8.8 Hz, 2H), 8.22 (d, J = 8.8 Hz, 2H); 13C -NMR (90 MHz, acetone-d6): δ = 55.65, 55.72, 114.68, 114.94, 122.43, 124.98, 127.53, 127.90, 128.01, 129.97, 144.73, 144.93, 147.77, 160.12, 160.88; ESI-MS: m/z = 450 [M+ + Na], 877 [2M+ + Na]; Anal. Calcd for C25H21N3O4 (427.45): C 70.25, H 4.95, N 9.83. Found: C 70.12, H 5.01, N 9.90.
2-(2-Nitrophenyl)-4,5-bis(4-methoxyphenyl)-1H-imidazole (2f): Yellowish solid. Yield 85%; mp 167-169 °C; Rf = 0.34 (SiO2; CH2Cl2/EtOAc 9:1); 1H-NMR (360 MHz, DMSO-d6): δ = 3.78 (s, 3H), 3.84 (s, 3H), 6.93 (d, J = 8.2 Hz, 2H), 7.06 (d, J = 8.2 Hz, 2H), 7.46 (t, J = 7.2 Hz, 4H), 7.64 (t, J = 7.7 Hz, 1H), 7.80 (t, J = 7.7 Hz, 1H), 7.94 (d, J = 8.0 Hz, 1H), 8.02 (d, J = 7.8 Hz, 1H), 12.84 (br s, 1H); 13C-NMR (90 MHz, DMSO-d6): δ = 55.03, 55.20, 113.71, 114.21, 123.07, 123.52, 123.97, 127.41, 127.77, 128.12, 129.23, 129.56, 129.60, 132.00, 136.94, 140.29, 148.28, 158.12, 158.97; ESI-MS: m/z = 424 [M+ + Na], 825 [2M+ + Na]; Anal. Calcd for C23H19N3O4 (401.41): C 68.82, H 4.77, N 10.47. Found: C 69.01, H 5.00, N 10.37.
2-(4-Cyanophenyl)-4,5-bis(4-methoxyphenyl)-1H-imidazole (2g): Orange solid. Yield 93%; mp 241-243 °C, lit.,14 242-243 °C; Rf = 0.43 (SiO2; CH2Cl2/EtOAc 9:1); 1H-NMR (500 MHz, DMSO-d6): δ = 3.76 (s, 3H), 3.79 (s, 3H), 6.88 (d, J = 6.5 Hz, 2H), 7.01 (d, J = 6.5 Hz, 2H), 7.44 (d, J = 8.8 Hz, 4H), 7.90 (d, J = 8.5 Hz, 2H), 8.21 (d, J = 8.5 Hz, 2H); 12.85 (br s, 1H); 13C-NMR (90 MHz, DMSO-d6): δ = 55.10, 55.23, 109.83, 113.76, 114.21, 119.03, 123.04, 125.39, 127.40, 128.33, 128.72, 129.85, 132.79, 134.46, 137.56, 143.08, 158,21, 159.08; ESI-MS: m/z = 404 [M+ + Na], 785 [2M+ + Na]; Anal. Calcd for C24H19N3O2 (381.43): C 75.57, H 5.02, N 11.02. Found: C 75.72, H 5.28, N 10.99.
2,4,5-Tris(4-nitrophenyl)-1H-imidazole (3): Yellow solid. Yield 84%; mp 310-311 °C, lit.,15 331-334 °C; Rf = 0.81 (SiO2; EtOAc/hexane 1:1); 1H-NMR (500 MHz, acetone-d6): δ = 7.94 (d, J = 6.9 Hz, 4H), 8.30 (d, J = 8.7 Hz, 4H), 8.38-8.43 (m, 4H); 13C-NMR (125 MHz, acetone-d6): δ =124.93, 125.09, 125.81, 127.29, 129.88, 136.5, 146.63, 147.92, 148.81; ESI-MS: m/z = 454 [M+ + Na], 885 [2M+ + Na]; Anal. Calcd for C21H13N5O6 (431.36): C 58.47, H 3.04, N 16.24. Found: C 58.62, H 3.00, N 16.16.
2,4,5-Tris(4-N,N-dimethylaminophenyl)-1H-imidazole (4a): Greenish solid. Yield 61%; mp 139-140 °C, lit.,16 245 °C; Rf = 0.22 (SiO2; EtOAc); 1H-NMR (360 MHz, acetone-d6): δ = 2.95 (s, 12H), 2.99 (s, 6H), 6.71 (d, J = 8.4 Hz, 4H), 6.79 (d, J = 8.2 Hz, 2H), 7.37-7.44 (m, 4H), 7.93 (d, J = 8.2 Hz, 2H); 13C-NMR (90 MHz, acetone-d6): δ = 40.56, 40.71, 112.56, 112.80, 113.03, 113.11, 120.68, 126.97, 129.38, 146.12, 150.73, 151.39; ESI-MS: m/z = 426 [MH+], 448 [M+ + Na], 873 [2M+ + Na]; Anal. Calcd for C27H31N5 (425.57): C 76.20, H 7.34, N 16.46. Found: C 76.02, H 7.21, N 16.26.
2,4,5-Tris(4-methoxyphenyl)-1H-imidazole (4b): Off-white solid. Yield 92%, mp 183-184 °C, lit.,17 248-249 °C; Rf = 0.67 (SiO2; EtOAc/hexane 1:1); 1H-NMR (360 MHz, acetone-d6): δ = 3.81 (s, 6H), 3.85 (s, 3H), 6.91 (d, J = 7.3 Hz, 4H), 7.00 (d, J = 8.8 Hz, 2H), 7.42-7.53 (m, 4H), 8.03 (d, J = 8.8 Hz, 2H); 13C-NMR (90 MHz, acetone-d6): δ = 55.63, 55.73, 114.67, 114.94, 124.79, 127.50, 129.95, 146.23, 159.85, 160.88; ESI-MS: m/z = 409 [M+ + Na], 795 [2M+ + Na]; Anal. Calcd for C24H22N2O3 (386.44): C 74.59, H 5.74, N 7.25. Found: C 76.00, H 5.78, N 7.31.
2-(5-Methylthiophen-2-yl)-4,5-bis(4-nitrophenyl)-1H-imidazole (5a): Orange solid. Yield 54%; mp 293-295 °C; Rf = 0.58 (SiO2; CH2Cl2/EtOAc 9:1); 1H-NMR (500 MHz, DMSO-d6): δ = 2.54 (s, 3H), 6.93 (d, J = 3.2 Hz, 1H), 7.59 (d, J = 3.2 Hz, 1H), 7.80 (d, J = 8.6 Hz, 4H), 8.23 (d, J = 8.3 Hz, 2H), 8.35 (d, J = 8.3 Hz, 2H), 13.22 (br s, 1H); 13C-NMR (125 MHz, DMSO-d6): δ = 15.08, 123.97, 124.25, 125.63, 126.56, 128.12, 129.23, 130.46, 136.64, 136.86, 141.03, 141.15, 143.82; ESI-MS: m/z = 429 [M+ + Na], 835 [2M+ + Na]; Anal. Calcd for C20H14N4O4S (406.41): C 59.11, H 3.47, N 13.79, S 7.89. Found: C 59.39, H 3.83, N 13.72, S 7.83.
2-(3-Methylthiophen-2-yl)-4,5-bis(4-nitrophenyl)-1H-imidazole (5b): Yellow solid. Yield 60%; mp 267-269 °C; Rf = 0.65 (SiO2; CH2Cl2/EtOAc 9:1); 1H-NMR (360 MHz, DMSO-d6): δ = 2.59 (s, 3H), 7.09 (d, J = 5.0 Hz, 1H), 7.62 (d, J = 5.0 Hz, 1H), 7.82 (d, J = 8.7 Hz, 4H), 8.26-8.41 (m, 4H), 13.02 (br s, 1H); 13C-NMR (90 MHz, DMSO-d6): δ = 15.25, 123.98, 125.80, 126.06, 131.42, 137.14, 143.46; ESI-MS: m/z = 429 [M+ + Na], 835 [2M+ + Na]; Anal. Calcd for C20H14O4N4S (406.41): C 59.11, H 3.47, N 13.79, S 7.89. Found: C 59.40, H 3.69, N 13.51, S 8.29.
2-(4,5-Dimethylthiophen-2-yl)-4,5-bis(4-nitrophenyl)-1H-imidazole (5c): Orange solid. Yield 56%; mp 303-305 °C; Rf = 0.61 (SiO2; CH2Cl2/EtOAc 9:1); 1H-NMR (360 MHz, DMSO-d6): δ = 2.19 (s, 3H), 2.39 (s, 3H), 7.50 (s, 1H), 7.78 (d, J = 8.8 Hz, 4H), 8.23 (d, J = 8.6 Hz, 2H), 8.34 (d, J = 8.6 Hz, 2H); 13.15 (br s, 1H); 13C-NMR (90 MHz, DMSO-d6): δ = 12.92, 13.46, 123.92, 124.20, 127.74, 127.84, 128.11, 128.31, 129.08, 133.82, 134.39, 136.61, 136.91, 141.06, 143.85, 146.02, 146.58; ESI-MS: m/z = 443 [M+ + Na], 863 [2M+ + Na]; Anal. Calcd for C21H16N4O4S (420.44): C 59.99, H 3.84, N 13.33, S 7.63. Found: C 59.82, H 3.69, N 13.51, S 7.59.
2-[5-(4-Methoxyphenyl)thiophen-2-yl]-4,5-bis(4-nitrophenyl)-1H-imidazole (5d): Red solid. Yield 58%; mp 299-301 °C; Rf = 0.63 (SiO2; CH2Cl2/EtOAc 9:1); 1H-NMR (500 MHz, DMSO-d6): δ = 3.85 (s, 3H), 7.06 (d, J = 8.8 Hz, 2H), 7.51 (d, J = 3.9 Hz, 1H), 7.72 (d, J = 8.8 Hz, 2H), 7.76 (d, J = 3.9 Hz, 1H), 7.83 (d, J = 8.8 Hz, 4H), 8.32 (d, J = 8.6 Hz, 4H), 13.61 (br s, 1H); 13C-NMR (125 MHz, DMSO-d6): δ = 55.34, 114.69, 123.37, 124.16, 125.99, 126.54, 126.86, 128.70, 129.24, 130.94, 143.68, 144.28, 146.40, 159.30; ESI-MS: m/z = 521 [M+ + Na], 1019 [2M+ + Na]; Anal. Calcd for C26H18N4O5S (498.51): C 62.64, H 3.64, N 11.24, S 6.43. Found: C 62.78, H 4.00, N 11.25, S 6.28.
4,5-Bis(4-nitrophenyl)-1H-imidazole (6): The title compound was prepared from the 4,4’-dinitrobenzil, formaldehyde (37%, aq. solution) and ammonium acetate following the general method. Yellowish solid. Yield 75%; mp 277-280 °C, lit.,15 181 °C; Rf = 0.44 (SiO2; EtOAc/ethanol 4:1); 1H-NMR (500 MHz, DMSO-d6): δ = 7.71-7.79 (m, 4H), 8.07 (s, 1H), 8.23-8.34 (m, 4H), 13.13 (br s, 1H); 13C-NMR (125 MHz, DMSO-d6): δ = 123.91, 124.23, 126.94, 128.02, 128.94, 136.30, 137.11, 138.00, 141.58, 145.95, 146.61; ESI-MS: m/z = 333 [M+ + Na], 643 [2M+ + Na]; Anal. Calcd for C15H10N4O4 (310.26): C 58.07, H 3.25, N 18.06. Found: C 58.09, H 3.30, N 18.11.
Crystallography: The X-ray data for 4,4’-dinitrobenzil and 4,5-bis(4-nitrophenyl)-1H-imidazole (6) were obtained at 150K using Oxford Cryostream low-temperature device on a Nonius KappaCCD diffractometer with MoKα radiation (λ = 0.71073 Å), a graphite monochromator, and the φ and χ scan mode. Data reductions were performed with DENZO-SMN[21]. The absorption was corrected by integration methods.[22] Structures were solved by direct methods (Sir92)[23] and refined by full matrix least-square based on F2 (SHELXL97)[24]. Hydrogen atoms were mostly localized on a difference Fourier map, however to ensure uniformity of treatment of crystal, all hydrogen were recalculated into idealized positions (riding model) and assigned temperature factors Hiso(H) = 1.2 Ueq (pivot atom) or of 1.5Ueq for the methyl moiety with C-H = 0.97, and 0.93 Å for methine and hydrogen atoms in aromatic ring and 0.86 Å for N-H moiety, respectively.
Crystallographic data for 4,4’-dinitrobenzil: C14H8N2O6, yellow plate, triclinic, P-1, a = 7.1021(7), b = 7.3989(13), c = 13.2870(7) Å, α = 84.647(9), β = 86.163(8), γ = 63.694(11)°, Z = 2, V = 622.91(13) Å3, Dc = 1.601 g.cm-3, µ = 0.128 mm-1, 11395 measured reflections (θmax = 27.5°), 2818 independent (Rint = 0.0705), 1838 observed I > 2σ(I), 199 parameters, R(obs) = 0.0590, wR = 0.1147.
Crystallographic data for 6: C15H10N4O4, yellow plate, monoclinic, C c, a = 6.845(1), b = 33.834(2), c = 6.426(1) Å, β = 118.29(5)°, Z = 4, V = 1310.4(2) Å3, Dc = 1.573 g.cm-3, µ = 0.118 mm-1, 7651 measured reflections (θmax = 27.5°), 2739 independent (Rint = 0.1068), 2143 observed I > 2σ(I), 209 parameters, R(obs) = 0.0658, wR = 0.1240.
Crystallographic data for structural analysis have been deposited with the Cambridge Crystallographic Data Centre, CCDC no. 719632 and 719633 for 4,4’-dinitrobenzil and 6, respectively. Copies of this information may be obtained free of charge from The Director, CCDC, 12 Union Road, Cambridge CB2 1EY, UK (fax: +44-1223-336033; e-mail: deposit@ccdc.cam.ac.uk or www: http:// www.ccdc.cam.ac.uk).
ACKNOWLEDGEMENTS
This research was supported by the Czech Science Foundation (203/08/0076) and the Ministry of Education, Youth and Sport of the Czech Republic (MSM 0021627501). A.P. thanks to Rector of the University of Pardubice for a post-doctoral intership.
References
1. P. N. Prasad and D. J. Williams, 'Introduction to Nonlinear Optical Effects in Organic Materials and Polymers,' , Wiley, New York, 1991.
2. a) S. R. Marder and J. W. Perry, Adv. Mater., 1993, 5, 804; CrossRef b) R. R. Tykwinski, U. Gubler, R. E. Martin, F. Diederich, C. Bosshard, and P. Gűnter, J. Phys. Chem. B, 1998, 102, 4451; CrossRef c) F. Bureš, O. Pytela, and F. Diederich, J. Phys. Org. Chem., 2008, . CrossRef
3. a) L.-T. Cheng, W. Tam, S. H. Stevenson, G. R. Meredith, G. Rikken, and S. R. Marder, J. Phys. Chem., 1991, 95, 10631 and 10634; CrossRef b) F. Bureš, W. B. Schweizer, C. Boudon, J.-P. Gisselbrecht, M. Gross, and F. Diederich, Eur. J. Org. Chem., 2008, 6, 994. CrossRef
4. A. Abbotto, L. Beverina, S. Bradamante, A. Facchetti, C. Klein, G. A. Pagani, M. Redi-Abshiro, and R. Wortmann, Chem. Eur. J., 2003, 9, 1991. CrossRef
5. a) F. Bureš, W. B. Schweizer, J. C. May, C. Boudon, J.-P. Gisselbrecht, M. Gross, I. Biaggio, and F. Diederich, Chem. Eur. J., 2007, 13, 5378; CrossRef b) J. C. May, I. Biaggio, F. Bureš, and F. Diederich, Appl. Phys. Lett., 2007, 90, 251106. CrossRef
6. a) C. R. Moylan, R. D. Miller, R. J. Twieg, K. M. Betterton, V. Y. Lee, T. J. Matray, and C. Nguyen, Chem. Mater., 1993, 5, 1499; CrossRef b) R. D. Miller, V. Y. Lee, and C. R. Moylan, Chem. Mater., 1994, 6, 1023. CrossRef
7. a) S. Wang, L. Zhao, Z. Xu, C. Wu, and S. Cheng, Mat. Lett., 2002, 56, 1035; CrossRef b) W. Wu, C. Ye, and D. Wang, Arkivoc, 2003, 59; c) W. Wu, Z. Zhang, and X. Zhang, J. Nonlinear Opt. Phys. Mater., 2005, 14, 61; CrossRef d) M. Kimura, H. Nishikawa, H. Kura, H. Lim, and E. H. White, Chem. Lett., 1993, 3, 505. CrossRef
8. a) A. Carella, R. Centore, A. Sirigu, A. Tuzi, A. Quatela, S. Schutzmann, and M. Casalboni, Macromol. Chem. Phys., 2004, 205, 1948; CrossRef b) A. Carella, R. Centore, A. Fort, A. Peluso, A. Sirigu, and A. Tuzi, Eur. J. Org. Chem., 2004, 2620. CrossRef
9. a) C. R. Moylan, B. J. McNelis, L. C. Nathan, M. A. Marques, E. L. Hermstad, and B. A. Brichler, J. Org. Chem., 2004, 69, 8239; CrossRef b) J. Santoz, E. A. Mintz, O. Zehnder, C. Bosshard, X. R. Bu, and P. Gűnter, Tetrahedron Lett., 2001, 42, 805; CrossRef c) W. Wu, Z. Zhang, and X. Zhang, J. Chem. Res., 2004, 617; CrossRef d) X. R. Bu, H. Li, D. Van Derveer, and E. A. Mintz, Tetrahedron Lett., 1996, 37, 7331. CrossRef
10. a) B. Radzisewski, Ber. Dtsch. Chem. Ges., 1882, 15, 1493; CrossRef b) J. Wang, R. Mason, D. VanDerveer, K. Feng, and X. R. Bu, J. Org. Chem., 2003, 68, 5415; CrossRef c) J. G. Lombardino and E. H. Wiseman, J. Med. Chem., 1974, 17, 1182. CrossRef
11. a) R. J. Cremlyn, F. J. Swinbourne, O. O. Shode, and J. Lynch, J. Heterocycl. Chem., 1987, 24, 117; CrossRef b) T. van Es and O. G. Backeberg, J. Chem. Soc., 1963, 1371. CrossRef
12. M. Kimura, R. E. McCluney, and W. H. Watson, Acta Cryst. B, 1979, 35, 483. CrossRef
13. X. R. Bu, D. VanDerveer, J. Santos, F.-L. Hsu, J. Wang, and K. Bota, Anal. Sci., 2003, 19, 469. CrossRef
14. Y. Sakaino, T. Takizawa, Y. Inouye, and H. Kakisawa, J. Chem. Soc., Perkin Trans. 2, 1986, 1623. CrossRef
15. T. van Es and O. G. Backeberg, J. Chem. Soc., 1963, 1363. CrossRef
16. H. Baumgärtel and H. Zimmermann, Chem. Ber., 1966, 99, 843. CrossRef
17. a) R. Gust, S. Busch, R. Keilitz, K. Schmidt, and M. von Rauch, Arch. Pharm.-Pharm. Med. Chem., 2003, 336, 456; CrossRef b) N. A. Lozinskaya, V. V. Tsybezova, M. V. Proskurnina, and N. S. Zefirov, Russ. Chem. Bull., Int. Ed., 2003, 52, 647.
18. a) T. Punniyamurthy, S. J. Karla, and J. Iqbal, Tetrahedron Lett., 1994, 35, 2959; CrossRef b) B. Bhatia, T. Punniyamurthy, and J. Iqbal, J. Org. Chem., 1993, 58, 5518. CrossRef
19. MOPAC2007 James J. P. Stewart, Stewart Computational Chemistry, version 7.264W, , webpage: http://OpenMOPAC.net.
20. F. Bureš, T. Szotkowski, J. Kulhánek, O. Pytela, M. Ludwig, and M. Holčapek, Tetrahedron:Asymmetry, 2006, 17, 900. CrossRef
21. Z. Otwinowski and W. Minor, ‘Methods in Enzymology,’, ed. by C. W. Carter and R. M. Sweet, Academic Press, New York, 1997, 276, pp. 307-326. CrossRef
22. P. Coppens, ‘Crystallographics Computing,’, ed. by F. R. Ahmed, S. R. Hall, and C. P. Huberts, Copenhagen, Munksgaard, 1970, pp. 255-270.
23. A. Altomare, G. Cascarano, C. Giacovazzo, and A. Guagliardi, J. Appl. Crystallogr., 1993, 26, 343. CrossRef
24. G. M. Sheldrick, ‘SHELXL-97’, University of Göttingen, Göttingen, 1997.