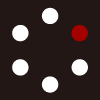
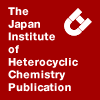
HETEROCYCLES
An International Journal for Reviews and Communications in Heterocyclic ChemistryWeb Edition ISSN: 1881-0942
Published online by The Japan Institute of Heterocyclic Chemistry
e-Journal
Full Text HTML
Received, 2nd December, 2008, Accepted, 9th January, 2009, Published online, 14th January, 2009.
DOI: 10.3987/COM-08-11615
■ Convenient Synthesis of Novel Bis(hydrazone) and Bis(indole) Derivatives
Nabila A. Kheder*
Department of Chemistry, Faculty of Science, University of Cairo, Giza 12613, Egypt
Abstract
The synthetic potency of (Z,Z)-3,3'-(ethane-1,2-diyldiimino)-dibut-2-enoate (1) as a versatile precursors for the synthesis of novel bis(hydrazone) and bis(indole) derivatives via its reaction with some electrophiles and nitrogen nuclephiles was investigated.The term enaminones was introduced by Greenhill to define the enamine of a 1,3-diketone, β-ketoester or similar 1,3-difunctional reagents, and is used to indicate any compound containing the conjugated system N-C=C-C=O.1a Several enaminone derivatives have proven to be valuable synthons for the synthesis of a wide variety of biologically active heterocyclic systems.1-3 In addition, enaminones are versatile intermediates that combine the ambident nucleophlicity of enamine with the ambident electrophlicity of enones. The chemistry of diethyl (Z,Z)-3,3'-(ethane-1,2-diyldiimino)dibut-2-enoate (1)4 is potentially very interesting when one considers that there are six nucleophilic sites (a, a', c, c', e and e') and four electrophilic sites (b, b', d and d') present in this moiety (Figure 1).
Compound 1 lies about a twofold axis and has a `twist' conformation, it adopts the Z,Z-configuration, which is shown to be governed mainly by intramolecular hydrogen bonds.5
Spectroscopic methods have shown that primary and secondary enaminones exist predominately, and in many cases completely, in the Z,s-Z form in solution of a polar solvents. An increase in solvent polarity, particularly solvents that form strong hydrogen bonds, increase contribution of the E forms.6
Bis(heterocycles) have received grate deal of attention, not only for being model compounds for main chain polymers,7-12 but also because many biologically active natural and synthetic products have molecular symmetry.13 Encouraged by these findings and in continuation of our previous work aimed at the synthesis of a variety of heterocyclic systems for biological and pharmacological evaluation,14-17 it was found that compound 1, is versatile and readily accessible building block for the synthesis of several new bis(heterocyclic) compounds of biological potency. In view of the above point, the reactions of compound 1 with some electrophiles and nitrogen nucleophiles were investigated.
Treatment of compound 1 with 3-chlorobenzenediazonium chloride (2) in EtOH, buffered with sodium acetate, afforded diethyl 3,3'-[ethane-1,2-diylbis(azan-1-yl-1-ylidene)]bis(2-[2-(3-chlorophenyl)hydrazo- no]butanoate) (3a) (Scheme 1). The IR spectrum of 3a showed an absorption band at 1693 cm-1 due to carbonyl group, in addition to absorption bands at 2982 and 3067 cm-1 due to aliphatic CH and NH groups, respectively. Its 1H NMR spectrum showed a triplet signal at δ 1.34 (J = 7.2 Hz) due to CH3 protons, a singlet signal at δ 2.44 due to CH3, a singlet signal at δ 4.0 due to NCH2 and a quartet signal at δ 4.30 (J = 7.2 Hz) due to CH2 protons. It showed also D2O-exchangeable signal at δ 15.0 due to NH proton, in addition to an aromatic multiplet in the region 7.16-7.58. Its mass spectrum revealed a peak at m/z 561 corresponding to its molecular ion. The key for determining the structure of the hydrazone (3a) is the presence of two D2O-exchangeable signals at δ 15.0 due to two NH protons of hydrazone, which indicate that the coupling occurred at position c and c' of compound 1. Compound 3a reacts with hydrazine hydrate to afford 4-(2-(3-chlorophenyl)hydrazono)-3-methyl-1H-pyrazol-5(4H)-one (5) (Scheme 1).
The IR spectrum of 5 showed an absorption band at 1666 cm-1 due to carbonyl group, in addition to absorption bands at 3036 and 3190 cm-1 due to two NH groups. Its 1H NMR spectrum showed signal at δ 2.12 due to CH3 and two D2O-exchangeable signals at δ 11.69 and 13.60 due to two NH protons, in addition to an aromatic multiplet in the region δ 7.11-7.70. The above reaction is assumed to proceed via an initial addition of the amino group of hydrazine to the imino moiety in the hydrazone (3a) to yield the corresponding acyclic non-isolable intermediate 4 which underwent intramolecular cyclization through loss of two EtOH and ethylenediamine molecules to give the final product 5 (Scheme 1). In a similar manner, compound (1) coupled smoothly with 4-methyl-3-phenylpyrazolyldiazonium chloride (6) in EtOH, buffered with sodium acetate to furnish a single product identified as pyrazolo[5,1-c] [1,2,4]triazine structure 8 (Scheme 2).
The 1H NMR spectrum of 8 showed a triplet signal at δ 1.51 (J = 7.2 Hz) due to CH3 protons, a singlet signal at δ 2.78 due to CH3, a singlet signal at δ 3.2 due to CH3, and a quartet signal at δ 4.59 (J = 7.2 Hz) due to CH2 protons, in addition to an aromatic multiplet in the region 7.41-7.91. Its mass spectrum showed a peak at m/z 296 due to its molecular ion. The indole moiety is featured in a variety of pharmacologically and biologically active compounds.18 Also, bis(indole) derivatives display diverse pharmacological activities and are useful in the treatment of fibromyalgia, chronic fatigue and irritable bowel syndrome.19 These compounds also inhibit the proliferation of both estrogen dependent and independent cultured breast tumor cells.20,21 In addition, bis(indole) was found in several naturally occurring alkaloids with important biological activity.22 In view of these findings, the present work was undertaken to develop a new method for the synthesis of bis(indole) derivatives. Thus, when compound (1) was treated with p-benzoquinone and with 2-methyl p-benzoquinone in acetic acid at 60 oC, yielded the bis(indole) structures (12a) and (12b), respectively (Scheme 3). The latter products were assumed to be formed via initial addition of the electron-rich moieties c and c' in the compound (1) to the electron-poor double bond system in the quinones followed by elimination of two water molecules to afford the final products (12a) and (12b), respectively.
The proposed mechanism is similar to examples reported in literature4b and to Nenitzescu reaction23-25 of quinones with enaminones. Spectroscopic data as well as elemental analyses of the obtained products were in complete agreement with the assigned structures of the compounds (12a,b).
The behaviour of compound 1 towards 5-amino-4-methyl-3-phenylpyrazole (13)26 as a nitrogen nucleophile and as potential precursors for the biologically interesting pyrazolo[1,5-a]pyrimidines was also investigated. Thus, when 1 was treated with 13, in the presence of piperidine, it afforded 3,5-dimethyl-2-phenylpyrazolo[1,5-a]pyrimidin-7(H)-one (17)26 (Scheme 4). The spectroscopic data as well as elemental analysis of the obtained product are in complete agreement with the assigned structure 17. Although the endocyclic NH in compound 13 is the most nucleophilic center, it is also the most sterically hindered site.27,28 Therefore, addition takes place at the exocyclic-NH2 to the α,β-unsaturated moiety in 1 to yield the corresponding acyclic non-isolable intermediate 14 which undergoes intramolecular cyclization through loss of two EtOH and ethylenediamine molecules to give the final product (17) (Scheme 4). The product obtained 17 was found to be identical in all respects [mp, mixed mp and IR spectrum] with that obtained from the reaction of ethyl acetoacetate with 13.
In conclusion, the reactivity of diethyl (Z,Z)-3,3'-(ethane-1,2- diyldiimino)dibut-2-enoate was investigated as a versatile, readily accessible building block for the synthesis of new compounds of biological and pharmaceutical importance.
EXPERIMENTAL
All melting points were measured on a Gallenkamp melting point apparatus. The infrared spectra were recorded in potassium bromide disks on a Pye Unicam SP 3300 and Shimadzu FT IR 8101 PC infrared spectrophotometers. The NMR spectra were recorded on a Varian Mercury VX-300 NMR spectrometer. 1H spectra were run at 300 MHz and 13C spectra were run at 75.46 MHz in deuterated dimethyl sulfoxide (DMSO-d6). Chemical shifts were related to that of the solvent. Mass spectra were recorded on a Shimadzu GCMS-QP 1000 EX mass spectrometer at 70 e.V. Elemental analyses were carried out at the Microanalytical Center of Cairo University, Giza, Egypt. Diethyl (Z,Z)-3,3'-(ethane-1,2-diyldiimino) dibut-2-enoate (1)4 and 5-amino-4-methyl-3-phenylpyrazole (13)26 were prepared according to the reported literature.
Synthesis of diethyl 3,3'-[ethane-1,2-diylbis(azan-1-yl-1-ylidene)]bis(2-[2-(3-chlorophenyl)hydrazono]- butanoate) (3a) and ethyl 4,8-dimethyl-7-phenylpyrazolo[5,1-c][1,2,4]triazine-3-carboxylate (8): General procedure.
To a cold solution of the compound (1) (0.284 g, 1 mmol) in EtOH (20 mL), buffered with sodium acetate trihydrate (3 g), was added the appropriate diazonium salt generated from 3-chloroaniline (2) or 5-amino-4- methyl-3-phenylpyrazole (6) [prepared by diazotizing the appropriate amine (2 mmol) in hydrochloric acid (6M, 1.2 mL) with sodium nitrite solution (0.138 g, 2 mmol) in water (1.0 mL)]. The addition was carried out portionwise with stirring at 0-5 ºC over a period of 30 min. After complete addition, the reaction mixture was stirred for further 4 h, then kept in an ice chest for 12 h, and finally diluted with water. The precipitated solid was collected by filtration, washed with water, dried and finally recrystallized from EtOH to afford dark yellow crystals from the corresponding products 3a and 8, respectively.
Diethyl 3,3'-[ethane-1,2-diylbis(azan-1-yl-1-ylidene)]bis(2-[2-(3-chlorophenyl)hydrazono]butanoate) (3a): Yield (72%), mp 176 °C; IR (KBr) ν 1693 (C=O), 3067 (NH) cm-1; 1H NMR (DMSO-d6) δ 1.34 (t, 6H, 2 COOCH2CH3, J = 7.2 Hz), 2.44 (s, 6H, 2CH3), 4.0 (s, 4H, 2NCH2), 4.30 (q, 4H, 2 COOCH2CH3, J = 7.2 Hz), 7.16-7.58 (m, 8H, Ar-H), 15.0 (s, 2H, D2O-exchangeable 2NH); MS m/z (%) 562 (5.23), 561 (M+, 3.14), 280 (12.83), 281 (3.37). Anal. Calcd for C26H30Cl2N6O4: C, 55.62; H, 5.39; N, 14.97; Cl, 12.63. Found: C, 55.65; H, 5.35; N, 14.99; Cl, 12.67%.
Ethyl 4,8-dimethyl-7-phenylpyrazolo[5,1-c][1,2,4]triazine-3-carboxylate (8): Yield (70%), mp 170 °C; IR (KBr) ν 1709 ( C=O) cm-1; 1H NMR (DMSO-d6) δ 1.51 (t, 3H, COOCH2CH3, J = 7.2 Hz), 2.78 (s, 3H, CH3), 3.2 (s, 3H, CH3), 4.59 (q, 2H, COOCH2CH3, J = 7.2 Hz), 7.41-7.91 (m, 5H, Ar-H); MS m/z (%) 298 (6.7), 297 (24.0), 296 (M+, 100.0), 224 (81.9), 155 (32.3), 115 (69.8), 77 (10.1), 67 (25.6). Anal. Calcd for C16H16N4O2: C, 64.85; H, 5.44; N, 18.91. Found: C, 64.80; H, 5.40; N, 18.96%.
Synthesis of 4-(2-(3-chlorophenyl)hydrazono)-3-methyl-1H-pyrazol-5(4H)-one (5):
To an ethanolic solution of hydrazone (3a) (0.561 g, 1 mmol) and hydrazine hydrate (80%, 0.2 mL, 2 mmol) was added a few drops of piperidine and the reaction mixture was refluxed for 4 h. The solvent was evaporated under reduced pressure and the residue was triturated with EtOH, filtered off, washed with EtOH and finally purified by recrystallization from EtOH/dioxane to afford yellow crystals of 4-(2-(3-chlorophenyl)hydrazono)-3-methyl-1H-pyrazol-5(4H)-one (5), yield (55%), mp 210 oC; IR (KBr) ν 1666 (C=O), 3036 (NH), 3190 (NH) cm-1; 1H NMR (DMSO-d6) δ 2.12 (s, 3H, CH3), 7.11-7.70 (m, 4H, Ar-H), 11.96 (s, 1H, D2O-exchangeable NH), 13.60 (s, 1H, D2O-exchangeable NH); 13C NMR (DMSO-d6) δ 11.46, 115.15, 119.85, 125.40, 128.54, 129.67, 130.43, 137.52, 146.59, 160.33. Anal. Calcd for C10H9ClN4O: C, 50.75; H, 3.83; N, 23.67; Cl, 14.98. Found: C, C, 50.77; H, 3.82; N, 23.65; Cl, 14.96%.
Synthesis of diethyl 1,1'-(ethane-1,2-diyl)bis(5-hydroxy-2-methyl-1-H-indole-3-carboxylate derivative (12a,b).
General procedure.
To a stirred solution of the compound (1) (0.284 g, 1 mmol) in AcOH (30 mL), was added p-benzoquinone or 2-methyl p-benzoquinone (2 mmol) with stirring for 12 h at 60 oC. The reaction mixture was evaporated in vacuo, and the obtain solid product was filtered off and recrystallized from DMF to afford the corresponding bis(indole) derivatives (12a,b).
Diethyl 1,1'-(ethane-1,2-diyl)bis(5-hydroxy-1-H-indole-3-carboxylate) (12a): Yield (72%), mp > 300 °C; IR (KBr) ν 1639 (C=O), 2978 (aliphatic CH), 3314 (OH) cm-1; 1H NMR (DMSO-d6) δ 1.28 (t, 6H, 2COOCH2CH3, J = 6.9 Hz), 2.00 (s, 6H, 2CH3,), 4.22 (q, 4H, 2COOCH2CH3, J = 6.9 Hz), 4.48 (s, 4H, 2NCH2), 6.64 (d, 2H, Ar-H), 7.16 (d, 2H, Ar-H), 7.36 (s, 2H, Ar-H), 8.95 (s, 2H, D2O-exchangeable 2OH); MS m/z (%) 465 (32.6), 464 (M+, 38.7), 435 (29.3), 434 (2.8), 232 (M+ /2, 95.6). Anal. Calcd for C26H28N2O6: C, 67.23; H, 6.08; N, 6.03. Found: 67.25; H, 6.04; N, 6.07%.
Diethyl 1,1'-(ethane-1,2-diyl)bis(5-hydroxy-2,6-dimethyl-1-H-indole-3-carboxylate (12b): Yield (66%), mp > 300 °C; IR (KBr) ν 1638 (C=O), 2977 (aliphatic CH), 3314 (OH) cm-1; 1H NMR (DMSO-d6) δ 1.07 (t, 6H, 2COOCH2CH3, J = 6.8 Hz), 1.90 (s, 6H, 2CH3,), 2.15 (s, 6H, 2CH3,), 4.21 (q, 4H, 2COOCH2CH3, J = 6.8 Hz), 4.44 (s, 4H, 2NCH2), 6.92 (s, 2H, Ar-H), 7.38 (s, 2H, Ar-H), 8.94 (s, 2H, D2O-exchangeable 2OH); MS m/z (%) 247 (17.41), 246 (M+/2, 100.0), 228 (16.18). Anal. Calcd for C28H32N2O6: C, 68.28; H, 6.55; N, 5.69. Found: C, 68.26; H, 6.54; N, 5.67%.
Synthesis of 3,5-dimethyl-2-phenylpyrazolo[1,5-a]pyrimidin-7(H)-one (17):
To a mixture of the compound 1 (0.284 g, 1 mmol) and compound 13 (0.173, 2 mmol) in absolute EtOH (25 mL) was added a few drops of piperidine and the reaction mixture was refluxed for 6 h then left to cool. The formed solid product was filtered off and recrystallized from DMF to afford the 3,5-dimethyl-2- phenylpyrazolo[1,5-a]pyrimidin-7(H)-one (17) in 58% yield, mp 308-310 °C (lit.26 mp 308-310°C); IR (KBr) ν 1630 (C=N), 1687 (C=O), 2883 (CH aliphatic), 3158 (NH) cm-1; 1H NMR (DMSO-d6) δ 2.25 (s, 3H, CH3), 2.28 (s, 3H, CH3), 5.56 (s, 1H, CH), 7.31-7.47 (m, 5H, Ar-H), 11.79 (s, 1H, D2O-exchangeable NH); 13C NMR (DMSO-d6) δ 13.0, 18.66, 95.55, 95.60, 126.69, 128.58, 129.35, 130.74, 138.65, 149.69, 150.62, 155.80; MS m/z (%) 241 (1.86), 240 (21.33), 239 (M+, 100.0). Anal. Calcd for C14H13N3O: C, 70.28; H, 5.48; N, 17.56. Found: C, 70.26; H, 5.47; N, 17.54%.
References
1. (a) J. V. Greenhill, Chem. Soc., Rev., 1977, 6, 277; CrossRef (b) E. Bejan, H. Ait-Haddou, J. C. Daran, and G. G. A. Balavoine, Eur. J. Org. Chem., 1998, 2907; CrossRef (c) M. N. Eberlin and C. Kascheres, J. Org. Chem., 1988, 53, 2084; CrossRef (d) A. W. Trautwein and G. Jung, Tetrahedron Lett., 1998, 39, 8263. CrossRef
2. (a) A. Alberola, L. A. Calvo, A. G. Ortega, M. C. S. Ruiz, P. Yustos, S. G. Granda, and E. Garcia- Rodriguez, J. Org. Chem., 1999, 64, 9493; CrossRef (b) I. Chaaban, J. V. Greenhill, and P. Akhtar, J. Chem. Soc., Perkin Trans. 1, 1979, 1593; CrossRef (c) R. Augusti and C. Kascheres, J. Org. Chem., 1993, 58, 7079; CrossRef (d) A. Muller, A. Maier, R. Neumann, and G. Maas, Eur. J. Org. Chem., 1998, 1177. CrossRef
3. B. Stanovnik and J. Svete, Chem. Rev., 2004, 104, 2433. CrossRef
4. (a) T. Mason, Ber., 1887, 20, 273; (b) A. S. Shyadligeri, G. S. Gadaginamath, and L. R. Subramanian, J. Chem. Res. (S), 1996, 114.
5. Z. Zhang, S. Jin, Z. Shang, S. Huang, B. Liu, and J. Guo, Acta Cryst., 2004, 60, 176.
6. C. M. Kascheres, J. Braz. Chem. Soc., 2003, 14, 945. CrossRef
7. S. Furuya, H. Matsumoto, Y. Hayase, N. Suzuki, and T. Imada, PCT Int Appl. WO 97 41, 126, [Chem. Abstr., 1998, 128, 13211f].
8. H. Vieweg, S. Leistner, J. Prantz, N. Bohm, and G. Wagner, Pharmazie, 1992, 47, 841.
9. A. G. Griffin and T. R. Britt, J. Am. Chem. Soc., 1981, 103, 4957. CrossRef
10. G. Galli, M. Laus, and A. S. Angeloni, Makromol. Chem., 1986, 187, 289. CrossRef
11. H. Ringsdorf, B. Schlarh, and J. Venzmer, Angew. Chem., Int. Ed. Engl., 1988, 27, 115. CrossRef
12. H. Finkelman, Angew. Chem., Int. Ed. Engl., 1987, 26, 816. CrossRef
13. C. Aguilera, M. Parra, and G. Fuentes, Z. Naturforsch, 1998, 53B, 367.
14. N. A. Khedr, Y. N. Mabkhot, and A. M. Farag, Heterocycles, 2008, 75, 887. CrossRef
15. N. A. Khedr, Y. N. Mabkhot, and A. M. Farag, Synth. Commun., 2008, 38, 3170. CrossRef
16. N. A. Khedr, Y. N. Mabkhot, and A. M. Farag, Heterocycles, 2008, 75, 2937. CrossRef
17. K. M. Dawood, A. M. Farag, and N. A. Khedr, Arkivoc, 2008, XV, 116.
18. R. J. Sundberg, The Chemistry of Indoles, Academic Press, New York, 1996, p. 113.
19. (a) A. Kathleen and A. G. Merrill, PCT. Int. Appl. WO 99, [Chem. Abstr., 1999, 130, 276765]; (b) C. A. Bradfield and L. F. Bjeldanes, J. Toxicol. Environ. Health, 1987, 21, 311; (c) R. H. Dashwood, L. Uyetake, A. T. Fong, J. D. Hendricks, and G. S. Bailey, Food Chem. Toxicol.,, 1987, 27, 385. CrossRef
20. (a) G. Xiaokang, F. A. Fares, and S. Yannai, Anticancer Res., 1999, 19, 3199; (b) Y. C. Chang, J. Riby, G. H. F. Chang, G. F. Peng, and L. F. Bjeldanes, Biochem. Pharmacol., 1999, 58, 825. CrossRef
21. (a) A. D. Shilling, D. B. Carlson, S. Katchamart, and D. Williams, Appl. Pharmacol., 2001, 170, 191; CrossRef (b) X. Ge, S. Yannai, G. Rennert, N. Gruener, and F. A. Fares, Biochem. Biophys. Res.Commun., 1996, 228, 153; CrossRef (c) X. Ge, F. A. Fares, and S. Yannai, Anticancer Res., 1999, 19, 3199.
22. Ki-Bong Oh, W. Mar, S. Kim, Ji-Yun Kim, Tae-Hoon Lee, Jae-Gyu Kim, D. Shin, C. J. Sim, and J. Shin, Biol. Pharm. Bull., 2006, 29, 570. CrossRef
23. C. D. Nenitzescu, Bull. Soc. Chem. Fr., 1929, 11, 37.
24. G. R. Allen, Org. Reactions, 1973, 20, 337.
25. G. R. Allen, C. Pidacks, and M. J. Weiss, J. Am. Chem. Soc., 1966, 88, 2536. CrossRef
26. S. Checchi, P. Papini, and M. Ridi, Gazz. Chim. Ital., 1955, 85, 1160.
27. M. H. Elnagdi, N. H. Taha, F. A. Abdel-All, R. M. Abdel-Motaleb, and F. F. Mahmoud, Collect. Czech. Chem. Commun., 1989, 54, 1082. CrossRef
28. A. M. Farag, K. M. Dawood, and H. A. Elmenoufy, Heteroatom Chem., 2004, 15, 508. CrossRef