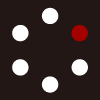
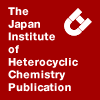
HETEROCYCLES
An International Journal for Reviews and Communications in Heterocyclic ChemistryWeb Edition ISSN: 1881-0942
Published online by The Japan Institute of Heterocyclic Chemistry
e-Journal
Full Text HTML
Received, 8th December, 2008, Accepted, 22nd January, 2009, Published online, 23rd January, 2009.
DOI: 10.3987/COM-08-11620
■ Synthesis of Homoproline Analogues Containing Heterocyclic Rings and Their Activity as Organocatalysts for Michael Reaction
Efrosini Barbayianni, Paola Bouzi, Violetta Constantinou-Kokotou, Valentine Ragoussis, and George Kokotos*
Laboratory of Organic Chemistry, Department of Chemistry, University of Athens, Panepistimiopolis, Athens 15771, Greece
Abstract
Two homoproline derivatives containing either the 5-thioxo-1,2,4-oxadiazole or the 2-oxo-1,2,3,5-oxathiodiazole bioisosteric groups, in replacement of the carboxyl group, were synthesized and their catalytic activities in Michael reactions were evaluated. The derivative containing the 5-thioxo-1,2,4-oxadiazole ring outperforms proline in the context of enantioselectivity in the reactions between β-nitrostyrene and acetone or cyclohexanone, indicating that the conversion of the carboxylic group of homoproline to a bioisosteric heterocyclic ring leads to a superior organocatalyst.INTRODUCTION
During the last few years organocatalysis has found wide application in asymmetric synthesis.1 In 2000, List, Lerner and Barbas reported that L-proline (1, Figure 1) was an effective asymmetric catalyst for the direct aldol reaction between acetone and a variety of aldehydes.2 Since then, numerous proline derivatives, as well as other chiral organic molecules have been described to catalyze enantioselective reactions.3 The use of different bioisosteric acid functionalities on proline-based catalysts has improved both the yield and the enantioselectivity for some aldol reactions. It has been shown by us and other groups that acyl sulfonamide derivatives of proline (2a) and 4-substituted proline (2b) are excellent organocatalysts for the asymmetric aldol reaction.4-7 Prolyl amides8 and thioamides9 have also been tested as catalysts for aldol reaction. Among the several carboxyl isosteric groups, heterocyclic rings are of great interest. The tetrazolyl analogue of proline (3a) has been studied as a catalyst for aldol and Michael reactions.7,10 The faster reaction rates with this tetrazolyl analogue of proline in DMSO were attributed to the lower pKa of the tetrazole moiety as compared to the carboxylic acid group of proline in DMSO.5,11 In addition, the tetrazole analogue is more soluble than proline in many organic solvents. Homoproline as well as homoproline tetrazole (3b) have also been reported as efficient catalysts for Michael reactions.12,13 The aim of the present work was to synthesize analogues of homoproline containing heterocyclic rings and evaluate their activity as organocatalysts in Michael reactions.
RESULTS AND DISCUSSION
Bioisosterism represents an interesting approach used in medicinal chemistry for the rational modification of lead compounds into agents exhibiting improved properties.14 A similar approach, followed for the design of new organocatalysts, led to the development of the acyl sulfonamides of proline4-7 and the tetrazole analogue of proline7,10 and homoproline.13 All these catalysts present better catalytic properties than the parent compound proline in aldol and Michael reactions. The 5-thioxo-1,2,4,-oxadiazole as well as the 2-oxo-1,2,3,5-oxathiodiazole rings are considered bioisosteric to the carboxylic group and there are examples in medicinal chemistry, where the replacement of a carboxyl by these rings leads to products with improved biological properties.15 The design of the new catalysts in this work was based on the replacement of the carboxyl of homoproline by the 5-thioxo-1,2,4,-oxadiazole and the 2-oxo-1,2,3,5-oxathiodiazole rings.
The synthesis of two homoproline analogues 8 and 10, where the carboxyl group is replaced by heterocyclic rings is depicted in Scheme 1. N-Benzyloxycarbonyl-L-prolinol (4) was prepared according to literature procedures.16,17 The hydroxyl group was activated by conversion to mesylate and was replaced by a cyano group by treatment with KCN.18 Amidoximes are compounds bearing both a hydroxyimino and an amino group at the same carbon atom, which makes them versatile building blocks for the synthesis of various heterocycles.19 Amidoxime 6 was prepared by treatment of nitrile 5 with hydroxylamine15,19 and served as a key intermediate for the construction of the heterocyclic rings. The 5-thioxo-1,2,4-oxadiazole derivative 7 was obtained by reaction of amidoxime 6 with 1,1′-thiocarbonyldiimidazole (TCDI) in the presence of 1,8-diazabicyclo[5.4.0]undec-7-ene (DBU). The 2-oxo-1,2,3,5-oxathiodiazole ring of compound 9 was formed after condensation of amidoxime 6 with thionyl chloride in the presence of pyridine.15 The benzyloxycarbonyl group of derivatives 7 and 9 was removed by treatment with 33% HBr/AcOH, affording the corresponding hydrobromic salts of catalysts 8 and 10.
The asymmetric Michael addition of carbonyl compounds to nitro-olefins is a very important and well studied synthetic transformation.20 L-Proline is known to catalyze the reaction between cyclohexanone and β-nitrostyrene in high yield and diastereoselectivity, but low enantioselectivity.21 In this work, this Michael reaction was studied and the results are summarized in Table 1. In accordance with literature, the product of the proline catalyzed reaction was isolated in 89% yield with a 15:1 dr and low ee (entry 1, Table 1). Catalyst 8 afforded the desired product in 72% yield and 79% ee when employed at 20% loading (entry 2, Table 1). Lower loading of 8 led to decreased yield and slightly improved ee (81%, entry 3, Table 1). In both cases, the diastereoselectivity of the reaction was high (12:1). When derivative 10 was used at 10% loading, the desired product was obtained in low yield, but quite high ee (69%, entry 4, Table 1). Thus, among the two heterocyclic derivatives, compound 8 at 20% catalyst loading provides the product of the reaction between cyclohexanone and β-nitrostyrene in good yield and high diastereoselectivity and enantioselectivity. Using this heterocyclic derivative, the enantioselectivity is far higher than that observed with proline and comparable to that using tetrazolyl homoproline as a catalyst.13 As it has been reported, homoproline by itself can not catalyze this particular Michael reaction.13
The Michael reaction between acetone and β-nitrostyrene was also studied. In accordance with literature,21 L-proline catalyzed this reaction with very low enantioselectivity at 20% catalyst loading (entry 1, Table 2). However, when catalyst 8 was used at the same loading, the results obtained were better than those using proline (entry 2, Table 2). Employing 8 at 10% loading, both the yield and the enantioselectivity were clearly improved (entry 3, Table 2). At an even lower catalyst loading, the yield was significantly decreased, while ee did not change (entry 4, Table 2). When the heterocyclic derivative 10 was used at 5-20% loading, the Michael product was isolated in very low yields, while ees did not exceed 28% (entries 5-7, Table 2). It is clear that the 5-thioxo-1,2,4-oxadiazole derivative 8 outperforms proline in the context of both enantioselectivity and yield.
Finally, the Michael reaction between acetophenone and β-nitrostyrene was also studied, since to our knowledge, the catalysis of this reaction by a proline derivative has not been reported up to now. However, both catalysts 8 and 10, when used at 10% loading, led to very low yields (5-10%), even for long reaction times (96 h), and moderate ees (43-47%).
Regarding the catalytic mechanism, two possible transition states have been described in the literature to explain the observed enantioselectivity in Michael reactions catalyzed by proline and its derivatives.13,22,23 In accordance with those data, two similar models may be proposed for the catalysis of the reaction between acetone and β-nitrostyrene by the 5-thioxo-1,2,4-oxadiazole derivative 8 (Figure 2). In both cases, an electrostatic interaction between the nitro group and the nitrogen of the enamine is involved. Model (A) suggests an extended hydrogen-bonded transition state. Model B proposes that the enantioselectivity is due to the steric hindrance of the heterocyclic ring substituent.
CONCLUSION
The present study shows that the replacement of the carboxyl group of homoproline by the bioisosteric 5-thioxo-1,2,4-oxadiazole moiety leads to an improved organocatalyst, concerning the reaction of β-nitrostyrene with cyclohexanone. The desired product is obtained in high chemical yield and high enantio- and diastereoselectivity. Using the same catalyst, the product between acetone and β-nitrostyrene is obtained in high chemical yield and four times higher enantioselectivity in comparison to that obtained using proline itself. Moreover, this is the first example of a heterocyclic analogue, other than the tetrazole homoproline, which leads to improved organocatalytic activities.
EXPERIMENTAL
General. All chemicals were purchased from Aldrich, Fluka or Alfa. Anhydrous solvents were prepared according to literature known procedures. TLC plates (silica gel 60 F254) and silica gel 60 (70–230 or 230–400 mesh) for column chromatography were purchased from Merck. Visualization of spots was effected with UV light and/or phosphomolybdic acid and/or ninhydrin, both in EtOH stain. Melting points were determined on a Buchi 530 apparatus and are uncorrected. Specific rotations were measured at 25 °C on a Perkin-Elmer 343 polarimeter, using a 10 cm cell. NMR spectra were recorded on a Varian Mercury (200 Mz) spectrometer. Where rotamers are apparent and resolved, peaks for major and minor rotamers are reported. Electron spray ionization (ESI) mass spectra were recorded on a Finnigan, Surveyor MSQ Plus spectrometer.
Synthesis of catalysts:
(S)-Benzyl 2-(cyanomethyl)pyrrolidine-1-carboxylate (5). To a stirred solution of alcohol 4 (2.82 g, 12 mmol) in CH2Cl2 (36 mL) were added Et3N (2.5 mL, 18 mmol) and methanesulfonyl chloride (1.4 mL, 18 mmol) in three doses at 0 oC. The resulting mixture was stirred at 0 oC for 30 min and at rt for 2 h. The organic solution was washed with a saturated aqueous solution of KHSO4, brine, 5% aqueous NaHCO3, dried over Na2SO4 and concentrated under reduced pressure. The residue was purified by column chromatography using EtOAc-petroleum ether (bp 40-60 °C) 1:1 as eluent. Oil; Yield 91% (3.05 g).
KCN (1.63 g, 25 mmol) was added to a stirred solution of the (S)-benzyl 2-(methylsulfonylmethyl)pyrrolidine-1-carboxylate (2.79 g, 10 mmol) in anhydrous DMF (20 mL). The resulting mixture was stirred at 60 oC for 48 h, diluted with water, and extracted with CH2Cl2. The organic layer was washed with brine, dried over Na2SO4 and evaporated under reduced pressure. The residue was purified by column chromatography using EtOAc-petroleum ether (bp 40-60 °C) 4:6 as eluent. Yield 76% (1.86 g); Oil; [α]D −84.8 (c 1, CHCl3); 1H NMR (200 MHz, CDCl3) δ 7.49-7.20 (m, 5H, Ph), 5.19-5.12 (m, 2H, CH2Ph), 4.12-4.02 (m, 1H, CH), 3.60-3.40 (m, 2H, CH2N), 2.98-2.56 (m, 2H, CH2CN), 2.25-1.78 (m, 4H, 2×CH2); 13C NMR (50 MHz, CDCl3) δ 154.8, 136.4, 128.4, 128.0, 127.8, 117.7, 66.9 (67.2), 54.1 (53.5), 46.9 (47.2), 30.3 (31.1), 23.6 (23.1), 22.1 (22.8); MS (ESI): m/z (%) 267 (100) [M + Na]+; Anal. Calcd for C14H16N2O2: C, 68.83; H, 6.60; N, 11.47. Found: C, 68.63; H, 6.84; N, 11.36.
(S, Z)-Benzyl 2-(2-amino-2-(hydroxyimino)ethyl)pyrrolidine-1-carboxylate (6). To a solution of nitrile 5 (1.83 g, 7.5 mmol) in MeOH (30 mL) cooled to 0 oC, hydroxylamine hydrochloride (0.76 g, 11 mmol) and triethylamine (1.5 mL, 11 mmol) were added. The mixture was stirred overnight at 60 oC. MeOH was removed under reduced pressure and CHCl3 was added. The organic layer was washed with brine, dried over Na2SO4 and evaporated under reduced pressure. The residue was purified by column chromatography using CHCl3/MeOH 9:1 as eluent. Yield 58% (1.2 g); Oil; [α]D −32.9 (c 1, CHCl3); 1H NMR (200 MHz, CDCl3) δ 7.60-7.26 (m, 5H, Ph), 5.21-5.08 (m, 2H, CH2Ph), 5.00 (br s, 2H, NH2), 4.39 (br s, 1H, OH), 4.19-3.98 (m, 1H, CH), 3.52-3.23 (m, 2H, CH2N), 2.75-2.25 (m, 1H, CHH), 2.23-2.07 (m, 1H, CHH), 1.98-1.75 (m, 4H, 2×CH2); 13C NMR (50 MHz, CDCl3) δ 155.0, 152.3, 136.4, 128.5, 128.0, 127.8, 66.8, 56.0, 46.5, 35.8, 29.8, 23.4; MS (ESI): m/z (%) 300 (100) [M + Na]+. Anal. Calcd for C14H19N3O3: C, 60.63; H, 6.91; N, 15.15. Found: C, 60.32; H, 7.04; N, 15.03.
(S)-Benzyl 2-((5-thioxo-4,5-dihydro-1,2,4-oxadiazol-3-yl)methyl)pyrrolidine-1-carboxylate (7). A mixture of amidoxime 6 (0.42 g, 1.5 mmol), TCDI (0.41 g, 2.3 mmol) and DBU (0.91 g, 6.0 mmol) in MeCN (15 mL) was stirred at rt overnight. The reaction mixture was concentrated in vacuo, diluted with water, adjusted to pH 2 with 1N HCl and extracted with EtOAc. The extract was dried over Na2SO4 and evaporated under reduced pressure. The residue was purified by column chromatography using EtOAc-petroleum ether (bp 40-60 °C) 1:1 as eluent. Yield 67% (0.32 g); Oil; [α]D +28.4 (c 0.5, CHCl3); 1H NMR (200 MHz, CDCl3) δ 7.40-7.21 (m, 5H, Ph), 5.21 (s, 2H, CH2Ph), 4.33-4.17 (m, 1H, CH), 3.61-3.38 (m, 2H, CH2N), 3.16 (dd, J1 = 4.4 Hz, J2 = 14.6 Hz, 1H, CHH), 2.73 (dd, J1 = 8.4 Hz, J2 = 14.6 Hz, 1H, CHH), 2.10-1.75 (m, 4H, 2×CH2); 13C NMR (50 MHz, CDCl3) δ 187.0, 157.6, 155.8, 135.8, 128.4, 128.0, 127.3, 67.6, 54.7, 46.6, 29.9, 28.4, 23.1; MS (ESI): m/z (%) 342 (44) [M + Na]+; Anal. Calcd for C15H17N3O3S: C, 56.41; H, 5.37; N, 13.16. Found: C, 56.23; H, 5.52; N, 13.04.
(S)-Benzyl 2-(2-oxo-1,2,3,5-oxathiodiazol-3-yl)methyl)pyrrolidine-1-carboxylate (9). To an ice-cooled solution of amidoxime 6 (0.55 g, 2.0 mmol) and pyridine (0.32 mL, 4.0 mmol) in THF (45 mL), a solution of thionyl chloride (0.15 mL, 2.02 mmol) in CH2Cl2 (9 mL) was added dropwise. The resulting mixture was stirred at 5 oC overnight. The reaction mixture was concentrated in vacuo, diluted with water, and extracted with CHCl3. The organic layer was dried over Na2SO4 and evaporated under reduced pressure. The residue was purified by column chromatography using EtOAc-petroleum ether (bp 40-60 °C) 1:1 as eluent. Yield 41% (0.26 g); Oil; [α]D −25.2 (c 0.85, CH3OH); 1H NMR (200 MHz, CDCl3) δ 7.66 (s, 1H, NH), 7.39-7.23 (m, 5H, Ph), 5.14 (s, 2H, CH2Ph), 4.23-4.09 (m, 1H, CH), 3.58-3.38 (m, 2H, CH2N), 3.00-2.59 (m, 2H, CH2), 2.08-1.81 (m, 4H, 2×CH2); 13C NMR (50 MHz, CDCl3) δ 155.9, 151.3, 136.3, 128.9, 128.2, 127.9, 67.3, 55.1 (55.5), 46.8, 30.7 (31.1), 28.8, 23.4; MS (ESI): m/z (%) 322 (100) [M − H]−; Anal. Calcd for C14H17N3O4S: C, 52.00; H, 5.30; N, 12.99. Found: C, 51.63; H, 5.14; N, 12.76.
Removal of Z group
A solution of the Z-protected compound (1 mmol) and 33% HBr/AcOH (10.5 mL) was stirred for 2 h at rt. The reaction mixture was poured in a mixture of petroleum ether (bp 40-60 °C)/Et2O 1:1 (10.5 mL) and the upper layer was removed. Anhydrous Et2O was added and the precipitated hydrobromide was filtered and washed with anhydrous Et2O.
(S)-3-(Pyrrolidin-2-ylmethyl)-1,2,4-oxadiazole-5(4H)-thione hydrobromide (8). Yield 84%; Hygroscopic white solid; [α]D +19.9 (c 2.4, MeOH); 1H NMR (200 MHz, CD3OD) δ 4.08-3.98 (m, 1H, CH), 3.43-3.01 (m, 4H, 2×CH2), 2.40-1.88 (m, 4H, 2×CH2); 13C NMR (50 MHz, CD3OD) δ 187.1, 166.2, 57.3, 47.0, 31.5, 27.7, 24.5; MS (ESI): m/z (%) 264 (100) [M − H]−; Anal. Calcd for C7H12BrN3OS: C, 31.59; H, 4.54; N, 15.79. Found: C, 31.32; H, 4.84; N, 15.47.
(S)-2-((2-Oxo-1,2,3,5-oxathiodiazol-3-yl)methyl)pyrrolidine hydrobromide (10). Yield 91%; Hygroscopic white solid; [α]D −3.4 (c 0.5, MeOH); 1H NMR (200 MHz, CD3OD) δ 4.09-3.91 (m, 1H, CH), 3.51-3.32 (m, 2H, CH2), 3.21-2.89 (m, 2H, CH2), 2.45-1.78 (m, 4H, 2×CH2); 13C NMR (50 MHz, CD3OD) δ 160.4, 58.2, 46.6, 31.6, 30.3, 23.9; MS (ESI): m/z (%) 212 (57) [M + Na - HBr]+; Anal. Calcd for C6H12BrN3O2S: C, 26.68; H, 4.48; N, 15.55. Found: C, 26.29; H, 4.79; N, 15.26.
General procedure for the Michael reaction.
To a suspension of catalyst in a mixture of iPrOH and EtOH (1:1, 8 mL), was added triethylamine (equimolar amount with the catalyst). trans-β-Nitrostyrene (0.15 g, 1 mmol) was added followed by acetone (2.2 mL, 30 mmol) or cyclohexanone (1.0 mL, 10 mmol) or acetophenone (1.2 mL, 10 mmol). The resulting mixture was allowed to stir at rt for 18 h (for 4 days for the reaction with acetophenone) and the solvents were evaporated in vacuo. EtOAc (30 mL) was added and the organic layer was washed with a saturated aqueous solution of NH4Cl (2×10 mL), dried over Na2SO4 and evaporated under reduced pressure. The residue was purified by column chromatography using EtOAc-petroleum ether (bp 40-60 °C) 2:8 as eluent.
(R)-5-Nitro-4-phenylpentan-2-one.21 1H NMR (200 MHz, CDCl3) δ 7.38-7.07 (m, 5H, Ph), 4.69 (dd, J1 = 7.0 Hz, J2 = 12.4 Hz, 1H, CHHNO2), 4.57 (dd, J1 = 7.6 Hz, J2 = 12.4 Hz, 1H, CHHNO2), 3.99 (qu, 1H, J = 6.8 Hz, CH), 2.90 (d, 1H, J = 7.0 Hz, COCH2), 2.10 (s, 3H, CH3); 13C NMR (50 MHz, CDCl3) δ 205.4, 138.8, 129.0, 127.9, 127.3, 79.4, 46.1, 39.0, 30.4; HPLC analysis: Daicel Chiralpak AD-H, hexane/i-PrOH 94:6, flow rate 1 mL/min, retention time: 11.86 (minor) and 12.78 (major).
(S)-2-[(R)-3-Nitro-1-phenylethyl]cyclohexanone.21 1H NMR (200 MHz, CDCl3) δ 7.36-7.07 (m, 5H, Ph), 4.93 (dd, 1H, J1 = 12.4 Hz, J2 = 4.8 Hz, CHHNO2), 4.61 (dd, 1H, J1 = 12.4 Hz, J2 = 10.0 Hz, CHHNO2), 3.83-3.65 (m, 1H, CH), 2.80-2.60 (m, 1H, CH), 2.58-2.32 (m, 2H, 2×CHH), 2.18-1.99 (m, 1H, CHH), 1.81-1.40 (m, 4H, 4×CHH), 1.28-1.16 (m, 1H, CHH); 13C NMR (50 MHz, CDCl3) δ 211.9, 137.7, 128.9, 128.1, 127.7, 78.9, 52.5, 43.9, 42.7, 33.2, 28.5, 25.0; HPLC analysis: Daicel Chiralpak AD-H, hexane/i-PrOH 95:5, flow rate 1 mL/min, retention time: 14.80 (minor) and 18.73 (major).
(R)-4-Nitro-1,3-diphenylbutan-1-one.24 1H NMR (200 MHz, CDCl3) δ 7.95-7.20 (m, 10H, Ph), 4.93-4.51 (m, 2H, CH2NO2), 4.25-4.07 (m, 1H, CH), 3.43 (d, J = 7.0 Hz, 2H, COCH2); 13C NMR (50 MHz, CDCl3) δ 196.8, 139.1, 136.4, 133.5, 129.1, 128.7, 128.0, 127.9, 127.4, 79.5, 41.5, 39.3; HPLC analysis: Daicel Chiralpak AD-H, hexane/iPrOH 90:10, flow rate 1 mL/min, retention time: 15.43 (minor) and 20.91 (major).
ACKNOWLEDGEMENTS
The project is co-funded by the European Social Fund and National Resources (EPEAEK II).
References
1. For books, see: (a) A. Berkessel and H. Groger, “Asymmetric Organocatalysis - From Biomimetic Concepts to Powerful Methods for Asymmetric Synthesis”, Wiley-VCH, Weinheim, 2005; (b) P. I. Dalko, “Enantioselective Organocatalysis: Reactions and Experimental Procedures”, Wiley-VCH, Weinheim, 2007.
2. B. List, R. A. Lerner, and C. F. Barbas III, J. Am. Chem. Soc., 2000, 122, 2395. CrossRef
3. For reviews, see: (a) P. I. Dalko and L. Moisan, Angew. Chem. Int. Ed., 2004, 43, 5138; CrossRef (b) M. S. Taylor and E. N. Jacobsen, Angew. Chem. Int. Ed., 2006, 45, 1520; CrossRef (c) B. List, Chem. Commun., 2006, 819; CrossRef (d) A. G. Doyle and E. N. Jacobsen, Chem. Rev., 2007, 107, 5713; CrossRef (e) H. Pellissier, Tetrahedron, 2007, 63, 9267; CrossRef (f) G. Guillena, C. Najera, and D. J. Ramon, Tetrahedron: Asymmetry, 2007, 18, 2249; CrossRef (g) S. Mukherjee, J. W. Yang, S. Hoffmann, and B. List, Chem. Rev., 2007, 107, 5471; CrossRef (h) S. Sulzer-Mosse and A. Alexakis, Chem. Commun., 2007, 3123; CrossRef (i) D. A. Longbottom, V. Franckevicius, S. Kumarn, A. J. Oelke, V. Wascholowski, and S. V. Ley, Aldrichimica Acta, 2008, 41, 3; (j) H. Kotsuki, H. Ikishima, and A. Okuyama, Heterocycles, 2008, 75, 493; CrossRef (k) H. Kotsuki, H. Ikishima, and A. Okuyama, Heterocycles, 2008, 75, 757. CrossRef
4. E. Bellis, K. Vasilatou, and G. Kokotos, Synthesis, 2005, 2407. CrossRef
5. A. Hartikka and P. I. Arvidsson, Tetrahedron: Asymmetry, 2004, 15, 1831. CrossRef
6. A. Berkessel, B. Koch, and J. Lex, Adv. Synth. Catal., 2004, 346, 1141. CrossRef
7. A. J. A. Cobb, D. M. Shaw, D. A. Longbottom, J. B. Gold, and S. V. Ley, Org. Biomol. Chem., 2005, 3, 84. CrossRef
8. (a) Z. Tang, Z.–H. Yang, X.-H. Chen, L.-F. Cun, A.-Q. Mi, Y.-Z. Jiang, and L.-Z. Gong, J. Am. Chem. Soc., 2005, 127, 9285; CrossRef (b) J. R. Chen, H.-H. Lu, X.-Y. Li, L. Cheng, J. Wan, and W.-J. Xiao, Org. Lett., 2005, 7, 4543; CrossRef (c) Z. Tang, Z.-H. Yang, L.-F. Cun, and L.-Z. Gong, Org. Lett., 2004, 6, 2285. CrossRef
9. D. Gryco and R. Lipinski, Adv. Synth. Catal., 2005, 347, 1948. CrossRef
10. (a) H. Torii, M. Nakadai, K. Ishihara, S. Saito, and H. Yamamoto, Angew. Chem. Int. Ed., 2004, 43, 1983; CrossRef (b) Y. Yamamoto, N. Momiyama, and H. Yamamoto, J. Am. Chem. Soc., 2004, 126, 5962. CrossRef
11. F. G. Bordwell, Acc. Chem. Res., 1988, 21, 456. CrossRef
12. D. Terakado, M. Takano, and T. Oriyama, Chem. Lett., 2005, 34, 962. CrossRef
13. C. E. T. Mitchell, A. J. A. Cobb, and S. V. Ley, Synlett, 2005, 611. CrossRef
14. G. A. Patani and E. J. LaVoie, Chem. Rev., 1996, 96, 3147. CrossRef
15. Y. Kohara, K. Kubo, E. Imamiya, T. Wada, Y. Inada, and T. Naka, J. Med. Chem., 1996, 39, 5228. CrossRef
16. G. Kokotos, Synthesis, 1990, 299. CrossRef
17. G. Kokotos and C. Noula, J. Org. Chem., 1996, 61, 6994. CrossRef
18. V. Constantinou-Kokotou and G. Kokotos, Org. Prep. Proced. Int., 1994, 26, 599.
19. K. C. Fylaktakidou, D. Hadjipavlou-Litina, K. E. Litinas, E. A. Varella, and D. N. Nicolaides, Curr. Pharm. Des., 2008, 14, 1001. CrossRef
20. For recent reviews, see: (a) S. B. Tsogoeva, Eur. J. Org. Chem., 2007, 1701; CrossRef (b) J. L. Vicario, D. Badia, and L. Carillo, Synthesis, 2007, 2065. CrossRef
21. B. List, P. Pojarliev, and H. J. Martin, Org. Lett., 2001, 3, 2423. CrossRef
22. D. Enders and A. Seki, Synlett, 2002, 26. CrossRef
23. Y. Hayashi, H. Gotoh, T. Hayashi, and M. Shoji, Angew. Chem. Int. Ed., 2005, 44, 4212. CrossRef
24. H. Huang and E. N. Jacobsen, J. Am. Chem. Soc., 2006, 128, 7170. CrossRef