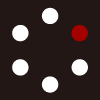
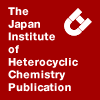
HETEROCYCLES
An International Journal for Reviews and Communications in Heterocyclic ChemistryWeb Edition ISSN: 1881-0942
Published online by The Japan Institute of Heterocyclic Chemistry
e-Journal
Full Text HTML
Received, 15th December, 2008, Accepted, 4th February, 2009, Published online, 6th February, 2009.
DOI: 10.3987/COM-08-11626
■ Thermal Decomposition of N-Alkylated 2-Aminobenzophenones
Evelyn Cuevas Creencia,* Atsushi Takahashi, and Takaaki Horaguchi*
Department of Chemistry, College of Science and Mathematics, MSU-Iligan Institute of Technology, Iligan City 9200, Philippines
Abstract
Thermal decomposition of N-alkylated 2-aminobenzophenones 1a-d was studied at 400-560 °C, using calcium oxide as catalyst. The reactions yielded both 5- and 6-membered heterocyclic compounds. The N-methylated-2-aminobenzophenones 1a-b decomposed at 400-560 °C to give 2-phenylindole 2 (47% at 500 °C from 1b), acridine 4 and phenanthridine 5, while the N-benzylated-2-aminobenzophenones 1c-d decomposed at 400-560 °C to give 2,3-diphenylindole 3 (33% at 450 °C and 400 °C from 1c and 1d, respectively), acridine 4 and phenanthridine 5.Thermal decomposition reaction is an interesting reaction to investigate since it can give a variety of products – some expected while others are unexpected, interesting, and useful in other fields of science.1 The formation of the different products may even occur via different reaction mechanisms. Several literatures report on the synthesis of carbazole by heating 2-nitrobiphenyl with deoxygenating agent such as iron(II) oxalate2 or triethyl phosphite3. Krbechek and Takimoto reported that thermal decomposition of o-substituted phenylazides, whenever possible, generally leads to 5-membered-ring structures.4 The formation of the cyclic products from the nitro- or azido-compounds were found to occur via the nitrene intermediate.5 Primary arylamines were also found to undergo dehydrocyclization reactions at 400-700 oC to yield carbazoles and phenoxazine, as reported by Horaguchi, et al.6 On the other hand, Tsang, et al reported the formation of N-substituted carbazoles from substituted arylamines by heating at 120 oC in the presence of either palladium or copper catalyst.7
In our laboratory, we have studied thermal decomposition of a number of aromatic amines by subjecting them to high temperatures using specialized apparatus in the presence of calcium oxide catalyst (Scheme 1). Thermal decomposition of 2-aminobiphenyl yielded carbazole (80% at 560 oC)8 while the N-alkylated-2-aminobiphenyls gave several products: carbazole, methyl carbazole, phenanthridine and phenanthrene.9 Thermal decomposition of N-alkyl-2-benzylanilines yielded acridine, anthracene, 2-phenylindole and 2,3-diphenylindole while thermal decomposition of N-alkyl-N’-phenyl-o-phenylenediamines yielded acridine, phenazine and 2-phenylbenzimidazole.10 We have proposed the formation of all these products to occur via nucleophilic substitution on the aromatic ring, a rather rare reaction mechanism, except for the phenylindoles and the benzimidazole which were formed by the reaction of either a carbanion or amide ion with an imine carbon.
In this paper, we report the results of thermal decomposition of N-alkylated-2-aminobenzophenones and compared these with our previous studies.
The starting materials used were prepared either by methylating or benzylating 2-aminobenzophenone as described in the Experimental Section. When heated at 400-560 oC in the presence of calcium oxide catalyst, 2-methylaminobenzophenone 1a yielded 2-phenylindole 2 (15-32%) with highest yield obtained at 400 oC (32%), acridine 4 (3-17%) and phenanthridine 5 (3-10%) (Table 1, Entries 1-4). With 2-dimethylaminobenzophenone 1b, the products formed by thermal decomposition at 400-500 oC were 2-phenylindole 2 (38-47%) with highest yield obtained at 500 oC (47%), acridine 4 (4-6%) and phenanthridine 5 (11-21%) (Table 1, Entries 5-7). While thermal decomposition at 450-600 oC of 2-aminobenzophenone8 yielded acridine (3-7%), acridone (21-32%) and carbazole (2-14%), the N-methylated-2-aminobenzophenone produced other products, 2-phenylindole 2 and phenanthridine 5, aside from acridine 4 (Scheme 2).
No carbazole and acridone were detected from the thermal decomposition of 2-methylamino- benzophenone 1a and 2-dimethylaminobenzophenone 1b. When 2-benzylaminobenzophenone 1c was heated at 400-500 oC in the presence of calcium oxide catalyst, 2,3-diphenylindole 3 (28-33%) with
highest yield obtained at 450 oC (33%), acridine 4 (3-5%) and phenanthridine 5 (7-11%) were produced (Table 2, Entries 1-3). 2-Dibenzylaminobenzophenone 1d also yielded 2,3-diphenylindole 3 (17-33%)
with highest yield obtained at 400 oC (33%), acridine 4 (8-16%) and phenanthridine 5 (4-6%) (Table 2, Entries 4-7), when heated at 400-560 oC in the presence of calcium oxide catalyst (Scheme 2). Again, no acridone and carbazole were detected but instead another product, 2,3-diphenylindole 3, was identified. The formation of 2-phenylindole 2 and 2,3-diphenylindole 3 could have occurred by the abstraction of H+ from the methyl or benzyl substituent by the calcium oxide catalyst followed by cyclization and rearrangement to give the final product (Scheme 3). It is interesting to note that the presence of methyl and benzyl groups on the nitrogen of 2-aminobenzophenone changed its chemistry and afforded other products, not observed with the unsubstituted aromatic amine, during thermal decomposition. Hence, a variety of other products may be obtained from thermal decomposition reactions by introducing other substituents on the aromatic amine substrates.
EXPERIMENTAL
Melting points are uncorrected. Column chromatography was performed on silica gel (Wakogel C-200). Unless otherwise stated, anhydrous sodium sulfate was employed as the drying agent. The IR spectra were determined on a Hitachi model 270-30 IR Spectrophotometer. The 1H and 13C NMR spectra were determined at 500MHz and 125MHz, respectively, on a Varian Unity plus-500W NMR Spectrophotometer, using tetramethylsilane as the internal standard.
General Procedure for the Thermolysis Reaction10 of N-Alkylated-2-aminobenzophenones. Elemental analysis apparatus (Micro Elemental Analyzer, Mitamura Riken Kogyo Inc.) was used for the thermolysis reaction. Granules of calcium oxide were obtained by grinding large pieces of calcium oxide (Nakalai Tesque, Inc.) and collecting particles which passed through the 5 mm sieve and retained by the 2 mm sieve. A quartz tube (66 cm in length, 12 mm i.d.) was packed to a length of 28 cm with the calcium oxide granules (18.0 g). The tube was positioned in the horizontal stationary furnace with heating coils (38 cm in length). The tube was then purged with N2 gas at a rate of about 19 mL/min and kept at this condition throughout the experiment. The stationary furnace was heated to the desired reaction temperature (400-560 oC). Starting material (1.0 mmole) was weighed into a quartz boat and placed inside the reaction tube at 3 cm from the stationary furnace and vaporized by the traveling furnace under N2 gas environment. When the desired reaction temperature was 400 oC, 450 oC or 500 oC, the stationary furnace was heated to 400 oC, 450 oC or 500 oC while the traveling furnace was heated to 560 oC. When the desired reaction temperature was 560 oC, the stationary furnace was heated to the desired temperature while the traveling furnace was heated 50 oC higher than the reaction temperature. The traveling furnace was set to motion gradually, reached the stationary furnace in 35 minutes and kept for 5 minutes at this state. Products which came out from the outlet (5 mm i.d.) of the quartz tube were collected in a vessel cooled with ice-water. The products were extracted with acetone. After removal of the acetone, the residue was chromatographed on a silica gel column and eluted with benzene, benzene-hexane or hexane-EtOAc to give various products. Structures of the products were determined from their spectra. 2-Phenylindole 2, acridine 4, and phenanthridine 5 were identified by comparison of the IR, 1H NMR and 13C NMR spectra with those of commercially available samples while 2,3-diphenylindole 3 was identified by comparison of its spectral data with literature value.
Synthesis of 2-methylaminobenzophenone 1a and 2-dimethylaminobenzophenone 1b. 2-Aminobenzophenone (1.978 g, 10.03 mmol) was placed in a 50 mL round bottom flask and added with dimethyl sulfoxide (4mL), tripotassium phosphate (1.589 g, 7.49 mmol), and methyl iodide (1.0 mL, 16.06 mmol). The mixture was heated in an oil bath at 50 oC for 2 h. The progress of the reaction was monitored by thin layer chromatography. After 2 h, methyl iodide (0.8 mL, 12.85 mmol) was again added and the mixture heated for another 1 h in the oil bath at 50 oC. The mixture was then extracted with EtOAc, washed with water and the organic layer separated and dried over anhydrous sodium sulfate. The filtrate was concentrated in a rotary evaporator. The resulting solution was chromatographed on a silica gel column using hexane:EtOAc (15:1) as eluting solvent. The reaction yielded 2-methylaminobenzophenone 1a (0.769 g, 36%), yellow crystals recrystallized in EtOH, and 2-dimethylaminobenzophenone 1b (0.790 g, 35%), yellow liquid.
2-Methylaminobenzophenone (1a).11 mp (EtOH) 66-67 oC (lit.,11 mp 65-69 oC); IR (KBr) 3340 cm-1 (NH), 1616 cm-1 (CO); 1H NMR (CDCl3): δ 2.97 (d, J=5.0 Hz, 3H, CH3), 6.54 (dd, J=8.0 Hz and 8.0 Hz, 1H, Ar-H), 6.76 (d, J=8.5 Hz, 1H, Ar-H), 7.40-7.52 (m, 5H, 5 Ar-H), 7.59 (d, J=8.0 Hz, 2H, 2 Ar-H), 8.54 (broad s, 1H, N-H ); 13C NMR: (CDCl3): δ 29.4 (q), 111.1 (d), 113.6 (d), 117.2 (s), 128.0 (d), 128.9 (d), 130.6 (d), 135.0 (d), 135.5 (d), 140.6 (s), 152. 7 (s), 199.3 (s).
2-Dimethylaminobenzophenone (1b). IR (film): 1652 cm-1 (CO); 1H NMR (CDCl3): δ 2.70 (s, 6H, 2 CH3), 6.90 (dd, J=8.0 Hz and 8.0 Hz, 1H, Ar-H), 6.99 (d, J=8.0 Hz, 1H, Ar-H), 7.32 (d, J=8.0 Hz, 1H, Ar-H), 7.38 – 7.43 (m, 3H, 3 Ar-H), 7.54 (t, J=8.5 Hz, 1H, Ar-H), 7.83 (d, J=8.5 Hz, 2H, 2 Ar-H); 13C NMR (CDCl3): 43.4 (q), 116.4 (d), 118.8 (d), 128.0 (s), 128.1 (d), 129.9 (d), 130.7 (d), 131.4 (d), 132.6 (d), 137.7 (s), 151.6 (s), 198.2 (s).
Synthesis of 2-benzylaminobenzophenone 1c and 2-dibenzylaminobenzophenone 1d. 2-Aminobenzophenone (1.975 g, 10.01 mmol) was placed in a 50 mL round bottom flask containing dimethyl sulfoxide (4 mL). Tripotassium phosphate (1.601 g, 7.54 mmol) and benzyl bromide (1.5 mL, 12.64 mmol) was then added and the mixture was heated in an oil bath at 50 oC for 3 h. The progress of the reaction was monitored by thin layer chromatography. After 3 h, benzyl bromide was added (1.0 mL, 8.42 mmol) and heating was continued for another 2 h. The resulting solution was extracted with EtOAc, washed with water and the organic layer was separated. The organic layer was dried with anhydrous sodium sulfate. The filtrate was then concentrated in a rotary evaporator. The resulting solution was chromatographed on a silica gel column using benzene:hexane (3:1) as the eluting solvent. The reaction yielded 2-benzylaminobenzophenone 1c (1.208 g, 42%), yellow crystals recrystallized in hexane, and 2-dibenzylaminobenzophenone 1d (1.171 g, 31%), yellow liquid.
2-Benzylaminobenzophenone (1c).12 mp (hexane): 81-82 oC (lit.,12 mp 84-86 oC), IR (KBr): 3340 cm-1 (NH), 1628 cm-1 (CO); 1H NMR (CDCl3): δ 4.49 (d, J=6.0 Hz, 2H, CH2), 6.63 (d, J=9.0 Hz, 1H, Ar-H), 7.26 – 7.39 (m, 7H, 7 Ar-H), 7.46 – 7.64 (m, 6H, 6 Ar-H), 8.94 (broad s, 1H, N-H); 13C NMR (CDCl3): δ 46.9 (t), 105.5 (s), 114.0 (d), 118.8 (d), 127.0 (d), 127.3 (d), 128.2 (d), 128.7 (d), 129.0 (d), 131.3 (d), 136.9 (d), 137.4 (d), 137.9 (s), 139.6 (s), 150.3 (s), 198.2 (s).
2-Dibenzylaminobenzophenone (1d). IR (film): 1658 cm-1 (CO); 1H NMR (CDCl3): δ 4.01 (s, 4H, 2 CH2), 6.79 – 6.85 (m, 4H, 4 Ar-H), 6.98 (d, J=8.0 Hz, 1H, Ar-H), 7.08 – 7.18 (m, 7H, 7 Ar-H), 7.34 – 7.39 (m, 2H, 2 Ar-H), 7.44 – 7.47 (m, 2H, 2 Ar-H), 7.59 – 7.63 (m, 1H Ar-H), 7.76 (d, J=8.0 Hz, 2H, 2 Ar-H); 13C NMR (CDCl3): δ 56.5 (t), 121.6 (s), 122.0 (d), 127.0 (d), 128.0 (d), 128.4 (d), 128.7 (d), 129.5 (d), 129.7 (d), 130.7 (d), 132.8 (d), 134.4 (d), 137.1 (s), 138.3 (s), 149.9 (s), 199.1 (s).
2-Phenylindole (2). mp (EtOH) 187-188 oC; IR (KBr): 3444 cm-1 (NH); 1H NMR (CDCl3): δ 6.83 (s, 1H, indole C3-H), 7.12 (dd, J=8.0 Hz and 8.0 Hz, 1H, Ar-H), 7.20 (dd, J=8.0 Hz and 8.0 Hz, 1H, Ar-H), 7.32 (dd, J=8.0 Hz and 8.0 Hz, 1H, Ar-H), 7.40 (d, J=8.0 Hz, 1H, Ar-H), 7.45 (dd, J=8.0 Hz and 8.0 Hz, 2H, 2 Ar-H), 7.63 (d, J=8.0 Hz, 1H, Ar-H), 7.67 ( d, J=8.0 Hz, 2H, 2 Ar-H), 8.33 (broad s, 1H, NH); 13C NMR (CDCl3): δ 100.0 (d), 110.9 (d), 120.2 (d), 120.6 (d), 122.3 (d), 125.1 (d), 127.7 (d), 129.0 (d), 129.2 (s), 132.3 (s), 136.8 (s), 137.9 (s).
2,3-Diphenylindole (3). mp (EtOH) 120-123 oC (lit.,13 mp 122-124 oC); IR (KBr) 3400 cm-1 (NH); 1H NMR (CDCl3): δ 7.15 (dd, J=7.5 Hz and 7.5 Hz, 1H, Ar-H), 7.25 (dd, J=7.5 Hz and 7.5 Hz, 1H, Ar-H), 7.29 (dd, J= 7.5 Hz and 7.5 Hz, 1H, Ar-H), 7.31 (dd, J=7.5 Hz and 7.5 Hz, 1H, Ar-H), 7.33 (dd, J=7.5 Hz and 7.5 Hz, 2H, 2 Ar-H), 7.38 (dd, J=7.5 Hz and 7.5 Hz, 2H, 2 Ar-H), 7.42 – 7.46 (m, 5H, 5 Ar-H), 7.68 (d, J=7.5 Hz, 1H, Ar-H), 8.23 (s, 1H, NH); 13C NMR (CDCl3): δ 110.9 (d), 115.0 (s), 119.7 (d), 120.4 (d), 122.7 (d), 126.2 (d), 127.7 (d), 128.2 (s), 128.5 (d), 128.7 (d), 128.7 (d), 130.1 (d), 132.7 (s), 134.1 (s),
135.0 (s), 135.9 (s). Spectral properties are identical with those in the literature.14
ACKNOWLEDGEMENT
ECC would like to thank the Japan Society for the Promotion of Science (JSPS) for the research grant at Niigata University, Japan.
References
1. R. Mahadevan, D. Lee, H. Sakurai, and M. R. Zachariah, J. Phys. Chem. A, 2002, 106, 11083; CrossRef W. C. Park, A. Atreya, and H. R. Baum, Proc. Combust. Inst., 2007, 31, 2643; CrossRef D. Dirtu, L. Odochian, A. Pui, and I. Humelnicu, CEJC, 2006, 4, 666. CrossRef
2. H. C. Waterman and D. L. Vivian, J. Org. Chem., 1949, 14, 289. CrossRef
3. J. I. G. Cadogan and M. Cameron-Wood, Proc. Chem. Soc., 1962, 361; J. I. G. Cadogan, M. Cameron-Wood, R. K. Mackie and R. J. G. Searle, J. Chem. Soc., 1965, 4831; CrossRef J. I. G. Cadogan, Quart. Rev., 1968, 22, 222. CrossRef
4. L. Krbechek and H. Takimoto, J. Org. Chem., 1968, 33, 4286. CrossRef
5. J. I. G. Cadogan, Acc. Chem. Res., 1972, 5, 303; CrossRef L. Krbechek and H. Takimoto, J. Org. Chem., 1968, 33, 4286; CrossRef J. I. G. Cadogan and S. Kulik, J. Chem. Soc. (C), 1971, 2621. CrossRef
6. T. Horaguchi, L. H. Klemm, and G. S. Norris, J. Heterocycl. Chem., 1995, 32, 797. CrossRef
7. W. C. P. Tsang, N. Zheng, and S. L. Buchwald, J. Am. Chem. Soc., 2005, 127, 14560. CrossRef
8. T. Horaguchi, T. Oyanagi, E. C. Creencia, K. Tanemura, and T. Suzuki, J. Heterocycl. Chem., 2004, 41, 1. CrossRef
9. E. C. Creencia and T. Horaguchi, J. Heterocycl. Chem., 2006, 43, 1441. CrossRef
10. E. C. Creencia, K. Taguchi, and T. Horaguchi, J. Heterocycl. Chem., 2008, 45, 837. CrossRef
11. H. Nishino and K. Kurosawa, Bull. Chem. Soc. Jpn., 1983, 56, 1682; CrossRef A. Couture, E. Deniau, Y. Gimbert, and P. Grandclaudon, J. Chem. Soc., Perkin Trans. 1, 1993, 2463; CrossRef K. K. Park, J. J. Lee, and J. Ryu, Tetrahedron, 2003, 59, 7651. CrossRef
12. K. Kobayashi, R. Nakahashi, A. Shimizu, T. Kitamura, O. Morikawa, and H. Konishi, J. Chem. Soc., Perkin Trans. 1, 1999, 1547. CrossRef
13. V. Nair and K. H. Kim, J. Org. Chem., 1975, 40, 3784; CrossRef W. F. Cockborn, R. A. W. Johnstone, and J. S. Stevens, J. Chem. Soc., 1960, 3340. CrossRef
14. K. M. Biswas, R. N. Dhara, H. Mallik, S. Halder, A. Sinha-Chaudhuri, A. Saha, D. Ganguly, P. De, and A. S. Brahmachari, Monat. Chem., 1996, 127, 111; CrossRef A. Furstner and A. Hupperts, J. Am. Chem. Soc., 1995, 117, 4468. CrossRef