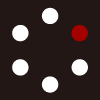
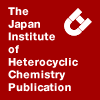
HETEROCYCLES
An International Journal for Reviews and Communications in Heterocyclic ChemistryWeb Edition ISSN: 1881-0942
Published online by The Japan Institute of Heterocyclic Chemistry
e-Journal
Full Text HTML
Received, 16th December, 2008, Accepted, 16th February, 2009, Published online, 17th February, 2009.
DOI: 10.3987/COM-08-11627
■ Synthesis of Indolylindolines Mediated by tert-BuNH2
Oscar R. Suárez-Castillo,* Myriam Meléndez-Rodríguez, Ana L. Morales-García, Indira C. Cano-Escudero, Yaneth M. A. Contreras-Martínez, Martha S. Morales-Ríos, and Pedro Joseph-Nathan
Área Académica de Química, Universidad Autónoma del Estado de Hidalgo, Mineral de la Reforma, Hidalgo, 42184, Mexico
Abstract
An improved synthesis of N1-carbomethoxylated indolylindolines 2 from 5-substituted indoles 1, by replacing the previously employed strong acids or toxic metals with t-BuNH2/MeOCOCl in CH2Cl2/H2O, is described. The substituent effect of electron donor or acceptor groups was examined, revealing that electron-deficient indoles are less reactive to dimerization than electron-rich indoles, with 5-cyano and 5-nitroindoles leading to no reaction. A dynamic process caused by the restricted rotation of the N-CO2Me bond of 2 was evidenced by 1H NMR measurements.INTRODUCTION
Due to the potential synthetic applications and biological effects of indole dimers1 there is a constant need to develop efficient protocols to achieve indole oligomerization. In general, such oligomerizations are effected under harsh reaction conditions in the presence of strong acids or toxic metals and tedious workup procedures are generally required. The acids frequently used are anhydrous HCl,2a,b HBr,2c NBS/AcOH,2d TFA,2e-h HCO2H,2i BF3·Et2O,2c ZnCl2/AcOH,2c Se, Pd-Charcoal2j and POCl3.2k Indole dimerization to indolylindolines is usually carried out by the acid-induced 3H-indolium cation which can react with an unprotonated indole molecule leading to indole dimers and/or 3,3’-(aminophenethylidene)diindoles.3 To the best of our knowledge only one example is reported for the oligomerization of indoles to give 3,3’-(aminophenethylidene)diindoles under basic conditions,4 using aqueous Na2CO3 or NaHCO3, with acyl chlorides or chloroformates. The latter work punctualized that if nitrogenated bases such as Et3N or N-methylpyrrolidine are used together with acyl chlorides, the only products obtained are the N-acyl protected indoles, as expected. In fact, we already reported our results on the protection of indole derivatives with MeOCOCl in the presence of NaH without dimerization or trimerization reactions.5 Recently, the InCl3/MeOCOCl/CH2Cl2 system has been used as catalyst in the synthesis of indole dimers and trimers.6 The authors report that in the absence of MeOCOCl, indoles give trimers.
Although there are a number of protocols to dimerize or trimerize indole derivatives, the main drawback of these procedures is the need to use anhydrous conditions. Thus, we report here on the details concerning an alternative simple procedure in which the use of t-BuNH2/MeOCOCl represents a valuable protocol for the synthesis of N1-carbomethoxylated indolylindolines 2, together with some amounts of 3,3’-(aminophenethylidene)diindoles 3, without the need to use anhydrous conditions. Indeed, reactions were carried out in aqueous mixtures to favor products 2, and this is the first time that dimers 2 and trimers 3 are obtained using a nitrogenated base.
RESULTS AND DISCUSSION
With the aim of exploring new applications of cheap and easily manageable t-BuNH2 in organic synthesis,7a,b as a base of low nucleophilicity when compared to other primary amines of similar basicity due to the steric effect of the t-butyl group,7c we decided to investigate its use to generate indole dimers 2. We examined the reactivity profile for a series of indoles 1a-g with MeOCOCl and t-BuNH2 (Scheme) whose results are summarized in Table 1. Control experiments were initially conducted without water, and it was found that the fastest and cleanest reaction was observed using 10 equivalents of amine and 8 equivalents of chloroformiate in CH2Cl2 at room temperature, although prolonged reaction times (120 h) were required to give indolylindoline 2a, trimer 3a and protected indole 4a8 in 50%, 23% and 5% yield, respectively (Table, Entry 1). Pure 2a showed spectroscopic data (1H NMR, 13C NMR, MS) consistent with those reported in the literature.6 Compound 3a was determined to be the corresponding aniline carbamate derivative as supported by 1H and 13C NMR and MS. With the objective of achieving the conversion of 1a into 2a or 3a in shorter reaction times, the number of equivalents of MeOCOCl were increased to 32 and the reaction was carried out under reflux, whereby the reaction time decreased to 74 h and compounds 2a and 3a were obtained in 60% and 10% yield, respectively (compare Entries 2 and 3).
It is worth noting that when t-BuNH2 and MeOCOCl are added to the solution of 1a in CH2Cl2, a precipitate is formed which is maintained through the reaction. This precipitate was identified by 1H and 13C NMR as tert-butyl ammonium chloride (t-BuNH2·HCl). Based on this information, the reaction for the transformation of 1a into 2a or 3a was carried out using water as a co-solvent thus dissolving the precipitate, and under the new reaction conditions the yields and reaction times vary notably. The optimized procedure for the transformation of 1a into 2a and 3a namely in the presence of 15 equivalents of t-BuNH2 and 30 equivalents of MeOCOCl under reflux of CH2Cl2/H2O, occurred in 21 h to give 2a and 3a in 79% (Lit. 56%)6 and 12% yield, respectively, together with traces of 4a (compare Entries 4 and 5). A plausible mechanism for the formation of dimer 2a and trimer 3a from 1a could involve tert-butylimidodicarbamate hydrochloride as the catalytic species, since the reaction occurs when t-BuNHCO2Me in the presence of an excess of MeOCOCl is used.
With successful reaction conditions established as in Entry 5, in order to explore the scope and limitations of this reaction, the reactivity profile for a series of readily available 5-substituted indoles 1b-g was studied, and the results are summarized in Table 1 (Entries 6-11). When 5-methylindole (1b) and 5-methoxyindole (1c) were reacted under the above optimized reaction conditions, the corresponding indolylindolines 2b and 2c were obtained in 67% and 59% yield, respectively (Entries 6 and 7). It should be noted that 5-aminoindole (1d) provides only dimer 2d (Entry 8); however, compared to 1a-c (Entries 5-7), the reaction was significantly slower, requiring 65 h for the complete consumption of starting 1d. Interestingly for 5-bromoindole (1e) the main product was trimer 3e, isolated in 48% yield, whereas dimer 2e was obtained in only 27% yield (Entry 9).
Attempts to dimerize indoles 1f and 1g carrying stronger electron-withdrawing groups such as –CN or –NO2 failed even after prolonged reaction times (Entries 10 and 11), as starting 1f or 1g were recovered. It is also worth noting that in the case of indoles 1e and 1g, the C-3 tert-butyl derivatives 4e and 4g were obtained in trace amounts (Entries 9 and 11). These compounds could be formed by reaction of 1e and 1g with the tert-butyl cation generated by thermal scission of tert-butylimidodicarbamate.7a,9 Indoles with substituents at C-3 like 3-methylindole did not react under the above reaction conditions.
Although indolylindoline 2a is known,6 its spectroscopic data are ambiguous mainly due to signal broadening, suggesting the presence of conformational isomers arising from slow rotation around the N-CO2Me bond,10 giving rise to the two mayor E and Z isomers denoted by the torsion angle C(7a)-N(1)-C=O. The room temperature (293 K) 1H NMR spectra of 2a, recorded in CDCl3 and Cl2CDCDCl2 exhibited extensive line broadening of aromatic protons and the N-carbomethoxyl group signals (Figure 1, B and C). When the 1H NMR spectrum of 2a was recorded in DMSO-d6 line broadening increased but the aromatic proton signals were dispersed (Figure 1, A) allowing unambiguous assignment by means of 1D (homonuclear 1H-1H decoupling, 13C{1H}) and 2D (1H-13C HMQC and HMBC) experiments.
The broadening of the aromatic protons and the N-carbomethoxyl group signals in 2a indicate that rotation around the N-CO2Me bond is the dominant dynamic process.10 This assumption was confirmed when the 1H NMR spectrum of 2a was obtained in Cl2CDCDCl2 at 100 °C (Figure 1, D), whereby the signals for H7 and the N-CO2Me group became sharpened. The dynamic process was also evidenced by comparison of the 1H NMR spectra of 2a and 2-(3’-indolyl)indoline 5,2i since the latter exhibited only sharp lines11 (Figure 1, E).
Furthermore, conformational evaluations of 2a were carried out by means of systematic and Monte Carlo search protocols within the Spartan 04 program12 employing the MMFF94 molecular mechanics force field, which afforded four conformers. These four structures were submitted to geometry optimization using DFT calculations at the B3LYP/6-31G(d) level of theory (Figure 2), from where the 3JH2,H3syn and 3JH2,H3anti values were calculated13 (Table 2), thus allowing individual assignments of the two H3 signals as is shown in Figure 1.
Compound 2a gave single crystals suitable for X-ray diffraction analysis, the corresponding structure being shown in Figure 3, where it is evident that the Z isomer is preferred in the crystalline state. The sum of bond angles around the carbamate N atom is 360°, suggesting that the geometry of the nitrogen atom is totally planar according to the degree of pyramidalization, defined as 360° - ∑(R-N-R).14 The five membered ring adopts a preferred enveloped conformation in which C-2 is the flap, as is evidenced from the torsion angles C(2)-C(3)-C(3a)-C(7a) (-13.6°) and C(2)-N(1)-C(7a)-C(3a) (12.4°).
In conclusion, we have developed a simple protocol for the oligomerization of indoles 1 into the corresponding selectively protected dimers 2 and trimers 3. The procedure employs operationally facile reaction conditions in yields ranging from fair to good, providing advantages over those previously reported. The method can be applied to indoles with substituent groups other than deactivating functionalities at the C-5 position on the indole ring.
EXPERIMENTAL
All commercial grade reagents were used without further purification. Analytical thin-layer chromatography (TLC) was performed on silica gel 60 F254 coated aluminum sheets (0.25 mm thickness) with a fluorescent indicator. Visualization was accomplished with UV light (254 nm). Flash chromatography was performed using silica gel 60 (230–400 mesh). Melting points were determined on a Büchi B-540 apparatus and are uncorrected. IR spectra were recorded on a Perkin Elmer 2000 FT-IR spectrophotometer. The 1H and 13C NMR spectra were recorded on JEOL Eclipse+ 400 and Varian Mercury spectrometers working at 400, 300 and 100, 75.4 MHz for 1H and 13C, respectively, using CDCl3 or DMSO-d6 as the solvent and TMS as the internal reference unless indicated otherwise. For complete data assignments, 2D HMQC and HMBC NMR spectra were used. Chemical shifts are reported as follows: chemical shift from TMS, integration, multiplicity (s = singlet; d = doublet; t = triplet; q = quartet; br = broad; m = multiplet), coupling constants (Hz) and assignment. NMR data are reported in parts per million downfield from tetramethylsilane. GC/MS analyses were conducted on a Varian CP 3800 GC equipped with a selective mass Varian Saturn 2000 detector and a 30 m, 0.25 mm i.d., 0.25 mm CP-SIL capillary column, using helium as carrier gas (1 mL/min), programmed from 70 °C to 250 °C at a rate of 30 °C/min, with the injector temperature at 200 °C. Relative percentage amounts were calculated from total ion chromatograms by the computer. MS analyses were obtained in the electron impact (EI) mode at an ionizing voltage of 70 eV on a Hewlett Packard 5989-A spectrometer. High-resolution (HR) mass spectra were measured at the UCR Mass Spectrometry Facility, University of California, Riverside, CA. Microanalytical determinations were performed on a Perkin Elmer 2400 Series PCII apparatus.
General procedure for the preparation of indolylindolines 2a-e and trimers 3a-d.
To a solution of the appropriate indole 1a (0.5 g, 4.30 mmol), 1b (0.15 g, 1.14 mmol), 1c (0.5 g, 3.40 mmol), 1d (0.15 g, 1.10 mmol), 1e (0.15 g, 0.80 mmol), 1f (0.15 g, 1.1 mmol) 1g (0.5 g, 3.10 mmol) in CH2Cl2/H2O (4.5-15/4.5-15 mL) were added 15 equiv of t-BuNH2. The solution was cooled to 5 °C and treated dropwise, over 15 min, with 30 equiv of ClCO2Me. After addition was complete, the mixture was stirred under reflux during 21 h for 1a, 27 h for 1b, 33 h for 1c, 65 h for 1d, 100 h for 1e, 100 h for 1f, and 164 h for 1g. After cooling to rt the organic phase was washed with a saturated aqueous solution of NH4Cl (2 x 20 mL), saturated aqueous solution of NaHCO3 (2 x 20 mL), brine (2 x 20 mL) dried over Na2SO4, filtrated and concentrated in vacum. The resultant crude products were purified by flash column chromatography with EtOAc/hexane 1:9.
N-Methoxycarbonyl-2-(3’-indolyl)indoline (2a).
Prepared from 1a as a white powder (0.493 g, 79.0%); mp 172-173 °C (CH2Cl2). Although 2a is known,4 its spectroscopic data are ambiguous. Thus, NMR data follow: 1H NMR (DMSO-d6, 400 MHz) δ 10.95 (1H, brs, N-H), 7.74 (1H, brs, H-7), 7.36 (1H, d, J = 8.1 Hz, H7’), 7.24 (1H, d, J = 6.5 Hz, H-4), 7.23 (1H, t, J = 6.5 Hz, H-6), 7.12 (1H, s, H-2’), 7.11 (1H, d, J = 7.7 Hz, H-4’), 7.04 (1H, t, J = 7.7 Hz, H-6’), 7.02 (1H, t, J = 7.6 Hz, H-5), 6.86 (1H, t, J = 7.4 Hz, H-5’), 5.76 (1H, dd, J = 10.2, 1.7 Hz, H-2), 3.71 (1H, dd, J = 16.3, 10.4 Hz, H-3syn), 3.63 (3H, s, Me), 3.04 (1H, dd, J = 16.3, 1.7 Hz, H-3anti); 13C NMR (DMSO-d6, δ 100 MHz) δ 153.1 (C=O), 142.0 (C-7a), 136.7 (C-7a’), 130.7 (C-3a), 127.4 (C-6), 124.9 (C-4), 124.5 (C-3a’), 122.8 (C-5), 122.6 (C-2’), 121.1 (C-6’), 118.7 (C-5’), 118.6 (C-4’), 116.7 (C-3’), 114.4 (C-7), 111.8 (C-7’), 56.2 (C-2), 52.3 (Me), 36.2 (C3); Anal. Calcd for C18H16N2O2: C 73.95; H 5.52; N 9.58. Found: C 73.81; H 5.52; N 9.30.
N-Methoxycarbonyl-2-[2,2-bis-(1H-indol-3-yl)ethyl]phenylamine (3a).
Prepared from 1a as a brown powder (69 mg, 12.0%); mp 170-172 °C (EtOAc/hexane); 1H NMR (DMSO-d6, 400 MHz) δ 10.70 (2H, brd, N-H), 8.91 (1H, brs, NH-CO2Me), 7.50 (2H, d, J = 8.1 Hz, H-4), 7.26 (2H, d, J = 8.0 Hz, H-7), 7.21 (1H, d, J = 7.7 Hz, H-12), 7.14 (2H, d, J = 2.2 Hz, H-2), 7.04 (1H, t, J = 7.6 Hz, H-13), 6.99 (1H, d, J = 7.5 Hz, H-15), 6.98 (2H, t, J = 7.8 Hz, H-6), 6.86 (1H, t, J = 7.4 Hz, H-14), 6.85 (2H, t, J = 7.5 Hz, H-5), 4.77 (1H, t, J = 7.7 Hz, H-8), 3.65 (3H, s, Me), 3.48 (2H, d, J = 7.7 Hz, H-9); 13C NMR (DMSO-d6, 100 MHz) δ 155.3 (C=O), 136.4 (C-7a), 136.1 (C-11), 136.0 (C-10), 129.9 (C-15), 126.6 (C-3a), 126.1 (C-12), 126.0 (C-13), 124.9 (C-14), 122.2 (C-2), 120.6 (C-6), 119.0 (C-4), 118.3 (C-3), 117.9 (C-5), 111.3 (C-7), 51.7 (Me), 36.8 (C-9), 33.3 (C-8); IR (CsI) νmax 3442, 3061, 3042, 2954, 1678, 1598, 1544, 1430 cm-1; EIMS m/z 409 [M+]+ (8), 292 (13), 245 (100), 218 (13), 117 (9). FABHRMS m/z 409.1785 (calcd for C26H23N3O2, 409.1790).
N-Methoxycarbonylindole (4a).
Prepared from 1a as a pale yellow oil (0.017 g, 2.0%).8
N-Methoxycarbonyl-2-(5’-methyl-3’-indolyl)-5-methylindoline (2b).
Prepared from 1b as a white powder (0.122 g, 67.0%); mp 136-139 °C (EtOAc/hexane); 1H NMR (DMSO-d6, 400 MHz) δ 10.76 (1H, s, N-H), 7.60 (1H, brs, H-7), 7.23 (1H, d, J = 8.4 Hz, H-7’), 7.03 (1H, brd, J = 8.1 Hz, H-6), 7.02 (1H, s, H-4), 6.97 (1H, d, J = 2.9 Hz, H-2’), 6.96 (1H, d, J = 1.8 Hz, H-4’), 6.88 (1H, dd, J = 8.4, 1.4 Hz, H-6’), 5.71 (1H, dd, J = 10.1, 2.4 Hz, H-2), 3.63 (1H, dd, J = 16.8, 10.6 Hz, H-3syn), 3.61 (3H, s, NCO2Me), 2.94 (1H, dd, J = 16.1, 1.8 Hz, H-3anti), 2.25 (6H, s, C5-Me, C5’-Me); 13C NMR (DMSO-d6, 100 MHz) δ 153.2 (C=O), 139.8 (C-7a), 135.1 (C-7a’), 131.9 (C-5), 130.9 (C-3a), 127.7 (C-6), 127.1 (C-5’), 125.7 (C-4), 125.0 (C-3a’), 122.9 (C-6’), 122.3 (C-2’), 118.5 (C-4’), 116.5 (C-3’), 114.3 (C-7), 111.6 (C-7’), 56.4 (C-2), 52.3 (NCO2Me), 36.4 (C-3), 21.5 (C5’-Me), 20.7 (C5-Me); IR (CsI) νmax 3339, 3019, 2952, 2857, 1686, 1596, 1449 cm-1; EIMS m/z 321 [M+1] + (20.9), 320 [M]+ (100), 262 (21), 190 (5), 130 (4). Anal. Calcd for C20H20N2O2: C 74.98; H 6.29; N 8.74. Found: C 74.82; H 6.34; N 8.48.
N-Methoxycarbonyl-2-[2,2-bis-(1H-5-methylindol-3-yl)ethyl]-4-methylphenylamine (3b).
Prepared from 1b as a pale yellow oil (24 mg, 14.0%); 1H NMR (CDCl3, 300 MHz) δ 7.86 (2H, s, N-H), 7.18 (2H, d, J = 8.0 Hz, H-7), 7.17 (2H, s, H-4), 7.05 (1H, brs, H-15), 6.95 (2H, dd, J = 8.4, 1.1 Hz, H-6), 6.96 (1H, d, J = 8.4 Hz, H-12), 6.92 (1H, dd, J = 8.5, 1.9 Hz, H-13), 6.88 (2H, d, J = 2.2 Hz, H-2), 5.75 (1H, brs, NH-CO2Me), 4.63 (1H, t, J = 7.3 Hz, H-8), 3.55 (3H, s, NCO2Me), 3.41 (2H, d, J = 7.7 Hz, H-9), 2.34 (6H, s, C5-Me), 2.25 (3H, s, C14-Me); 13C NMR (CDCl3, 75 MHz) δ 155.2 (C=O), 135.1 (C-7a), 134.5 (C-14), 133.5 (C-11), 132.9 (C-10), 131.1 (C-15), 128.6 (C-5), 127.5 (C-13), 127.0 (C-3a), 123.7 (C-6), 123.5 (C-12), 122.3 (C-2), 119.3 (C-4), 118.7 (C-3), 111.0 (C-7), 52.2 (NCO2Me), 37.6 (C-9), 36.4 (C-8), 21.6 (C5-Me), 21.1 (C14-Me); IR (CsI) νmax 3407, 3121, 3014, 2920, 1713, 1614, 1516 cm-1; EIMS m/z 451 [M]+ (1), 274 (25), 273 (100), 86 (26), 49 (57). FABHRMS m/z 451.2253 (calcd for C29H29N3O2, 451.2260).
N-Methoxycarbonyl-5-methylindole (4b).
Prepared from 1b as a yellow powder (6 mg, 3.0%); mp 53-55 °C (EtOAc/hexane); 1H NMR (CDCl3, 400 MHz) δ 8.03 (1H, brd, J = 7.0 Hz, H-7), 7.53 (1H, d, J = 3.3 Hz, H-2), 7.33 (1H, d, J = 0.8 Hz, H-4), 7.13 (1H, d, J = 8.4 Hz, H-6), 6.50 (1H, d, J = 3.7 Hz, H-3), 4.00 (3H, s, NCO2Me), 2.42 (3H, s, C5-Me); 13C NMR (CDCl3, 100 MHz) δ 151.7 (C=O), 133.5 (C-7a), 132.6 (C-5), 130.8 (C-3a), 125.9 (C-6), 125.6 (C-2), 121.0 (C-4), 114.8 (C-7), 108.0 (C-3), 53.8 (NCO2Me), 21.4 (C5-Me); IR (CsI) νmax 3023, 3153, 2955, 1732, 1585, 1468 cm-1; EIMS m/z 190 [M+1] + (15), 189 [M]+ (100), 174 (3), 144 (46), 130 (22). FABHRMS m/z 189.0785 (calcd for C11H11N1O2, 189.0790).
N-Methoxycarbonyl-2-(5’-methoxy-3’-indolyl)-5-methoxyindoline (2c).
Prepared from 1c as a white powder (0.352 g, 59.0%); mp 190-192 °C (EtOAc/hexane); 1H NMR (DMSO-d6, 400 MHz) δ 10.78 (1H, s, N-H), 7.63 (1H, brs, H-7), 7.23 (1H, d, J = 8.4 Hz, H-7’), 7.09 (1H, d, J = 2.6 Hz, H-2’), 6.92 (1H, d, J = 2.2 Hz, H-4), 6.81 (1H, dd, J = 8.6, 2.4 Hz, H-6), 6.68 (1H, dd, J = 8.6, 2.4 Hz, H-6’), 6.48 (1H, d, J = 2.6 Hz, H-4’), 5.69 (1H, dd, J = 10.0, 2.0 Hz, H-2), 3.71 (3H, s, C5-OMe), 3.66 (1H, dd, J = 16.5, 5.5 Hz, H-3syn), 3.62 (3H, brs, NCO2Me), 3.50 (3H, s, C5’-OMe), 2.98 (1H, brdd, J = 16.4, 1.4 Hz, H-3anti); 13C NMR (DMSO-d6, 100 MHz) δ 155.7 (C-5), 153.1 (C-5’, C=O), 135.7 (C-7a), 132.7 (C-3a), 131.8 (C-7a’), 124.8 (C-3a’), 123.6 (C-2’), 116.7 (C-3’), 115.1 (C-7), 112.5 (C-7’), 112.4 (C-6), 111.3 (C-6’), 111.0 (C-4), 100.8 (C-4’), 56.7 (C-2), 55.5 (C5-OMe), 55.0 (C5’-OMe), 52.3 (NCO2Me), 36.3 (C-3); IR (KBr) νmax 3446, 2956, 1675, 1597, 1542 cm-1; EIMS m/z 353 [M+1] + (21), 352 [M]+ (100), 293 (21), 147 (27). Anal. Calcd for C20H20N2O4: C 68.17; H 5.72; N 7.95. Found: C 68.11; H 5.76; N 7.89.
N-Methoxycarbonyl-2-[2,2-bis-(1H-5-methoxyindol-3-yl)ethyl]-4-methoxyphenylamine (3c).
Prepared from 1c as a pale yellow oil (46 mg, 8.0%); 1H NMR (DMSO-d6, 400 MHz) δ 10.56 (2H, brs, N-H), 8.75 (1H, brs, NH-CO2Me), 7.17 (1H, d, J = 8.8 Hz, H-7), 7.13 (1H, d, J = 1.9 Hz, H-2), 7.06 (1H, brd, J = 7.7 Hz, H-12), 7.00 (1H, d, J = 2.2 Hz, H-4), 6.66 (1H, dd, J = 8.8, 2.2 Hz, H-6), 6.60 (1H, dd, J = 8.6, 2.8 Hz, H-13), 6.50 (1H, d, J = 2.9 Hz, H-15), 4.67 (1H, t, J = 7.5 Hz, H-8), 3.68 (6H, s, 2C5-OMe), 3.61 (3H, s, NCO2Me), 3.41 (3H, s, C14-OMe), 3.39 (2H, d, J = 7.5 Hz, H-9); 13C NMR (DMSO-d6, 100 MHz) δ 156.5 (C-14), 155.8 (C=O), 152.6 (C-5), 138.2 (C-10), 131.6 (C-7a), 129.0 (C-11), 127.8 (C-12), 127.1 (C-3a), 123.1 (C-2), 118.2 (C-3), 115.4 (C-15), 111.8 (C-7), 111.3 (C-13), 110.5 (C-6), 101.4 (C-4), 55.4 (C5-OMe), 54.7 (C14-OMe), 51.6 (NCO2Me), 37.1 (C-9), 33.1 (C-8); IR (KBr) νmax 3418, 2918, 2849, 1706, 1485 cm-1; EIMS m/z 499 [M]+ (7), 353 (13), 352 (53), 305 (100), 147 (39). FABHRMS m/z 499.2111 (calcd for C29H29N3O5, 499.2107).
N-Methoxycarbonyl-5-methoxyindole (4c).8
Prepared from 1c as a yellow powder (9 mg, 1.0%); mp 77-78 °C (EtOAc/hexane); 1H NMR (CDCl3, 400 MHz) δ 8.05 (1H, s, H-7), 7.57 (1H, d, J = 3.0 Hz, H-2), 7.03 (1H, d, J = 2.5 Hz, H-4), 6.94 (1H, dd, J = 8.8, 2.5 Hz, H-6), 6.53 (1H, d, J = 4.1 Hz, H-3), 4.02 (3H, s, NCO2Me), 3.85 (3H, s, C5-OMe); 13C NMR (CDCl3, 100 MHz) δ 156.2 (C-5), 151.6 (C=O), 131.4 (C-3a), 130.1 (C-7a), 126.3 (C-2), 115.9 (C-7), 113.4 (C-6), 108.2 (C-3), 103.7 (C-4), 55.8 (C5-OMe), 53.9 (NCO2Me); IR (CsI) νmax 3001, 2956, 2834, 1736, 1588, 1474 cm-1; EIMS m/z 206 [M+1] + (13), 205 [M]+ (100), 190 (41), 174 (1), 146 (28), 131 (5), 118 (37), 59 (3).
N-Methoxycarbonyl-2-(5’-amino-3’-indolyl)-5-aminoindoline (2d).
Prepared from 1d as a white powder (0.146 g, 59.0%); mp 197-200 °C (EtOAc/hexane); 1H NMR (DMSO-d6, 400 MHz, at 70 °C) δ 10.62 (1H, s, N-H’), 9.26 (2H, s, NH), 8.99 (2H, s, NH), 7.60 (1H, d, J = 8.4 Hz, H-7), 7.44 (1H, brs, H-4’), 7.34 (1H, brs, H-4), 7.27 (1H, dd, J = 8.6, 2.0 Hz, H-6), 7.26 (1H, d, J = 8.4 Hz, H-7’), 7.09 (1H, dd, J = 8.5, 1.6 Hz, H-6’), 6.97 (1H, d, J = 2.2 Hz, H-2’), 5.72 (1H, dd, J = 9.7, 2.3 Hz, H-2), 3.68 (1H, dd, J = 16.5, 9.9 Hz, H-3syn), 3.66 (3H, s, OMe), 3.64 (3H, s, OMe), 3.63 (3H, s, OMe), 3.00 (1H, dd, J = 16.3, 2.0 Hz, H-3anti); 13C NMR (DMSO-d6, 100 MHz, at 70 °C) δ 154.6, 154.3, 153.1 (3C=O), 137.1, 134.3, 133.3, 131.0, 130.6, 124.8, 122.6, 120.7, 119.4, 117.8, 116.7, 116.0, 115.6, 114.4, 111.4, 109.8, 56.1 (C-2), 52.1 (OMe), 51.4 (OMe), 51.3 (OMe), 36.4 (C-3); IR (CsI) νmax 3537, 2953, 2923, 1703, 1551 cm-1; EIMS m/z 438 [M]+ (13), 406 (51), 374 (100), 315 (23), 190 (15), 158 (34). Anal. Calcd for C22H22N4O6: C 60.27; H 5.06; N 12.78. Found: C 60.21; H 5.05; N 12.48.
N-Methoxycarbonyl-2-(5’-bromo-3’-indolyl)-5-bromoindoline (2e).
Prepared from 1e as a pale yellow oil (46 mg, 27.0%); 1H NMR (CDCl3, 300 MHz) δ 8.09 (1H, brs, N-H), 7.70 (1H, brs, H-7), 7.49 (1H, brs, H-4’), 7.37 (1H, dd, J = 8.6, 2.0 Hz, H-6), 7.30 (1H, brs, H-4), 7.27 (1H, dd, J = 8.5, 1.9 Hz, H-6’), 7.21 (1H, d, J = 6.7 Hz, H-7’), 6.99 (1H, brs, H-2’), 5.76 (1H, dd, J = 10.3, 2.4 Hz, H-2), 3.72 (3H, brs, Me), 3.69 (1H, dd, J = 16.4, 10.1 Hz, H-3syn), 3.10 (1H, dd, J = 16.5, 2.4 Hz, H-3anti); 13C NMR (CDCl3, 75 MHz) δ 153.9 (C=O), 141.5 (C-7a), 135.1 (C-7a’), 132.7 (C-3a), 130.6 (C-6), 127.9 (C-4), 127.0 (C-3a’), 125.2 (C-6’), 122.6 (C-2’), 121.7 (C-4’), 118.0 (C-3’), 118.0 (C-7), 115.4 (C-5), 113.1 (C-5’), 112.8 (C-7’), 56.1 (C-2), 52.9 (Me), 36.2 (C-3); IR (CsI) νmax 3336, 3076, 2955, 1705, 1567, 1478 cm-1; EIMS m/z 451 [M+1]+ (20), 450 [M]+ (100), 449 (47), 310 (15), 114 (2). FABHRMS m/z 447.9418 (calcd for C18H14N2O2Br2, 447.9422).
N-Methoxycarbonyl-2-[2,2-bis-(1H-5-bromoindol-3-yl)ethyl]-4-bromophenylamine (3e).
Prepared from 1e as a pale yellow oil (80 mg, 48.0%); 1H NMR (CDCl3, 300 MHz) δ 8.19 (2H, s, N-H), 7.43 (2H, s, H-4), 7.29 (1H, s, H-12), 7.25 (1H, d, J = 1.2 Hz, H-15), 7.25 (1H, dd, J = 6.7, 2.3 Hz, H-13), 7.20 (2H, dd, J = 8.7, 1.8 Hz, H-6), 7.16 (2H, d, J = 8.5 Hz, H-7), 6.92 (2H, d, J = 2.3 Hz, H-2), 5.76 (1H, s, NH-CO2Me), 4.51 (1H, t, J = 7.5 Hz, H-8), 3.57 (3H, s, Me), 3.35 (2H, d, J = 7.5 Hz, H-9); 13C NMR (CDCl3, 75 MHz) δ 154.5 (C=O), 135.2 (C-7a), 134.9 (C-14), 134.3 (C-10), 133.1 (C-15), 130.1 (C-13), 127.9 (C-3a), 125.0 (C-6), 124.6 (C-12), 123.0 (C-2), 121.7 (C-4), 117.7 (C-11), 117.4 (C-3), 112.8 (C-7), 112.8 (C-5), 52.4 (Me), 36.6 (C-9), 36.1 (C-8); IR (CsI) νmax 3427, 3365, 3123, 2871, 1716, 598 cm-1; EIME m/z 647 [M+1]+ (2), 646 [M]+ (1), 404 (55), 402 (100), 400 (51). FABHRMS m/z 644.9095 (calcd for C26H20N3O2Br3, 644.9085).
3-tert-Butyl-5-bromoindole (4e).
Prepared from 1e as a pale yellow oil (14 mg, 7.0%); IR 1H NMR (CDCl3, 400 MHz) δ 7.93 (1H, d, J = 0.8 Hz, H-4), 7.91 (1H, brs, N-H), 7.25 (1H, dd, J = 8.5, 1.6 Hz, H-6), 7.22 (1H, d, J = 8.4 Hz, H-7), 6.94 (1H, d, J = 2.6 Hz, H-2), 1.43 (9H, s, 3Me); 13C NMR (CDCl3, 100 MHz) δ 135.9 (C-7a), 127.8 (C-3a), 126.7 (C-3), 124.4 (C-6), 123.9 (C-4), 120.7 (C-2), 112.8 (C-7), 112.2 (C-5), 31.7 (C-8), 30.9 (3Me); IR (CsI) νmax 3391, 2963, 2905, 1459, 1435, 1413 cm-1; EIME m/z 253 [M+1]+ (19), 252 [M]+ (6), 238 (100), 236 (96), 157 (56), 115 (3), 57 (2). FABHRMS m/z 251.0309 (calcd for C12H14N1Br1, 251.0310).
3-tert-Butyl-5-nitroindole (4g).
Prepared from 1g as a pale yellow oil (20 mg, 3.0%); 1H NMR (CDCl3, 400 MHz) δ 8.79 (1H, d, J = 1.8 Hz, H-4), 8.43 (1H, brs, N-H), 8.09 (1H, dd, J = 8.8, 2.2 Hz, H-6), 7.39 (1H, d, J = 9.1 Hz, H-7), 7.10 (1H, d, J = 2.2 Hz, H-2), 1.48 (9H, s, 3Me); 13C NMR (CDCl3, 100 MHz) δ 141.1 (C-5), 140.4 (C-7a), 129.6 (C-3), 125.4 (C-3a), 122.5 (C-2), 118.6 (C-4), 117.4 (C-6), 111.3 (C-7), 31.8 (C-8), 31.0 (3Me); IR (CsI) νmax 3354, 3093, 2900, 1620, 1542 cm-1; EIME m/z 219 [M+1]+ (3), 218 [M]+ (11), 204 (12), 203 (100), 157 (18). FABHRMS m/z 218.1058 (calcd for C12H14N2O2, 218.1055).
Preparation of t-BuNHCO2Me and t-BuNH2·HCl.
A sample of ClCO2Me (128 mmol, 9.9 mL) was added dropwise to cooled (ice-water bath) t-BuNH2 (64 mmol, 6.7 mL) and the mixture was stirred at room temperature for 30 min. The solid formed was filtrated and washed with CHCl3 (2 x 5 mL) affording t-BuNH2·HCl (2.37 g, 34%) as a white solid; mp 303-305 °C. The filtrated was evaporated under reduced pressure to give pure t-BuNHCO2Me as a pale yellow oil; 1H NMR for t-BuNH2·HCl (DMSO-d6, 400 MHz) δ 8.26 (3H, s, RNH3), 1.29 (9H, s, 3Me); 13C NMR for t-BuNH2·HCl (DMSO-d6, 100 MHz) δ 51.1 (C[Me]3), 27.1 (3Me); IR (CsI) νmax 2895, 2961 cm-1; EIMS m/z 74 [M]+ (11), 58 (100), 57 (8); 1H NMR for t-BuNHCO2Me (CDCl3, 400 MHz) δ 4.81 (1H, s, N-H), 3.47 (3H, s, OMe), 1.19 (9H, s, 3Me); 13C NMR for t-BuNHCO2Me (CDCl3, 100 MHz) δ 155.4 (NCO2Me), 51.2 (NCO2Me), 50.1 (C[Me]3), 28.9 (3Me).
X-Ray diffraction analysis of 2a. Single crystals of 2a were grown by slow crystallization from methylene chloride. The X-ray data were collected on a Bruker-Nonius CAD4 diffractometer equipped with Cu-Kα radiation (λ = 1.54184 Å). The data were collected in the ω-2θ scan mode. Unit cell refinements were done using the CAD4 Express v 2.0 software. The structure was solved by direct methods using the SIR0215 program included in the WinGX v 1.64.05 crystallographic software package.16 For the structural refinement, the non-hydrogen atoms were treated anisotropically, and the hydrogen atoms, included in the structure factor calculation, were refined isotropically. Compound 2a crystallized as colorless blocks in the monoclinic space group P21/a with cell dimensions a = 7.736(4) Å, b = 24.096(3) Å, c = 7.989(5) Å, β = 101.71(5)° and was refined to final R indices (all data) R (%) = 4.8, Rw (%) = 13.4. Data collection and refinement parameters, atom coordinates, bond lengths and bond angles have been deposited with the Cambridge Crystallographic Data Centre. The CCDC deposition number is 715888.
ACKNOWLEDGEMENTS
This research was supported in part by CONACYT (Mexico) grant 2002-C01-40641/A-1 and UAEH-PAI 2006-30B.
References
1. (a) Y.-H. Wu, W. G. Lobeck, and R. P. Ryan, J. Med. Chem., 1972, 15, 529; CrossRef (b) N. Wahlström, J. Slätt, B. Stensland, A. Ertan, J. Bergman, and T. Janosik, J. Org. Chem., 2007, 72, 5886. CrossRef
2. (a) R. L. Hinman and E. R. Shull, J. Org. Chem., 1961, 26, 2339; CrossRef (b) W. E. Noland and C. F. Hammer, J. Org. Chem., 1960, 25, 1525; CrossRef (c) H. Ishii, K. Murakami, E. Sakurada, K. Hosoya, and Y. Murakami, J. Chem. Soc., Perkin Trans. 1, 1988, 2377; CrossRef (d) T. Hino, M. Tonozuka, and M Nakagawa, Tetrahedron, 1974, 30, 2123; CrossRef (e) Y. Omori, Y. Matsuda, S. Aimoto, Y. Shimonishi, and M. Yamamoto, Chem. Lett., 1976, 805; CrossRef (f) J. D. Chisholm, and D. L. Van Vranken, J. Org. Chem.,, 1995, 60, 6672; CrossRef (g) S. J. Stachel, R. L. Habeeb, and D. L. Van Vranken, J. Am. Chem. Soc., 1996, 118, 1225; CrossRef (h) V. Bocchi and G. Palla, Tetrahedron, 1986, 42, 5019; CrossRef (i) M. Chakrabarty, S. Khasnobis, Y. Harigaya, and Y. Konda, Synth. Commun., 2000, 30, 187; CrossRef (j) J. Bergman, J. Heterocycl. Chem., 1973, 121; CrossRef (k) J. R. Frost, B. R. P. Gaudillière, E. Kauffmann, D. Loyaux, N. Normand, G. Petry, P. Poirier, E. Wenkert, and A. E. Wick, Heterocycles, 1989, 28, 175. CrossRef
3. (a) G. F. Smith, Chem. Ind., 1954, 1451; (b) G. F. Smith, Adv. Heterocycl. Chem., 1963, 2, 287; CrossRef (c) J. A. Joule, K. Mills, and G. F. Smith, In Heterocyclic Chemistry; 3rd ed., Chapman and Hall: London, 1995.
4. P. Bourgeois, J. Mesdouze, and E. Philogène, J. Heterocycl. Chem., 1983, 20, 1043. CrossRef
5. O. R. Suárez-Castillo, M. Sánchez-Zavala, M. Meléndez-Rodríguez, L. E. Castelán-Duarte, M. S. Morales-Ríos, and P. Joseph-Nathan, Tetrahedron, 2006, 62, 3040. CrossRef
6. B. Pal, V. S. Giri, and P. Jaisankar, Catal. Commun., 2005, 6, 711. CrossRef
7. (a) O. R. Suárez-Castillo, L. A. Montiel-Ortega, M. Meléndez-Rodríguez, and M. Sánchez-Zavala, Tetrahedron Lett., 2007, 48, 17; CrossRef (b) O. R. Suárez-Castillo, L. A. Montiel-Ortega, M. J. Fragoso-Vázquez, M. Meléndez-Rodríguez, and M. Sánchez-Zavala, Tetrahedron Lett., 2008, 49, 996; CrossRef (c) F. Brotzel, Y. C. Chu, and H. Mayr, J. Org. Chem., 2007, 72, 3679. CrossRef
8. W.-C. Shieh, S. Dell, A. Bach, O. Repiĉ, and T. J. Blacklock, J. Org. Chem., 2003, 68, 1954. CrossRef
9. E. L. Dias, K. W. Hettenbach, and D. J. am Ende, Org. Process Res. Dev., 2005, 9, 39. CrossRef
10. O. R. Suárez-Castillo, Y. M. A. Contreras-Martínez, L. Beiza-Granados, M. Meléndez-Rodríguez, J. R. Villagómez-Ibarra, J. M. Torres-Valencia, M. S. Morales-Ríos, and P. Joseph-Nathan, Tetrahedron, 2005, 61, 8809. CrossRef
11. J. Banerji, R. Saha, A. Patra, S. Manna, N. Shoolery, T. Prange, and C. Pascard, Indian J. Chem., 1982, 21B, 83.
12. B. Ganguly, D. A. Freed and M. C. Kozlowski, J. Org. Chem., 2001, 66, 1103. CrossRef
13. PCMODEL V 6.00 Serena Software, Bloomington, IN 47402-3076, USA.
14. (a) MMFF94 force field and DFT B3LYP/6-31G(d) as implemented in the computer package Spartan ’04, windows v 1.0.1; Wavefunction Inc. Irvine, CA, USA, 2004; (b) G. Chang, W. C. Guida, and W. C. Still, J. Am. Chem. Soc., 1989, 111, 4379; CrossRef (c) W. J. Hehre, A Guide to Molecular Mechanics and Quantum Chemical Calculations, Wavefunction: Irvine, CA, USA, 2003; (d) U. Burket and N. L. Allinger, Molecular Mechanics; ACS Monograph 177, American Chemical Society: Washington, DC, 1982; (e) W. J. Hehre, L. Radom, P. V. R. Schleyer, and J. A. People, Ab Initio Molecular Orbital Theory, Wiley: New York, 1986.
15. M. C. Burla, M. Camalli, B. Carrozzini, G. L. Cascarano, C. Giacovazzo, G. Polidori, and R. Spagna, J. Appl. Cryst., 2003, 36, 1103. CrossRef
16. L. J. Farrugia, J. Appl. Cryst., 1999, 32, 837. CrossRef