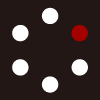
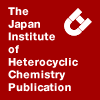
HETEROCYCLES
An International Journal for Reviews and Communications in Heterocyclic ChemistryWeb Edition ISSN: 1881-0942
Published online by The Japan Institute of Heterocyclic Chemistry
e-Journal
Full Text HTML
Received, 12th January, 2009, Accepted, 29th January, 2009, Published online, 30th January, 2009.
DOI: 10.3987/COM-09-11649
■ Synthesis of (–)-Heliannuol E via Mercuric Triflate Catalyzed Arylene Cyclization
Tomoyo Kamei, Tamiko Takahashi, Masahiro Yoshida, and Kozo Shishido*
Graduate School of Pharmaceutical Sciences, University of Tokushima, Sho-machi, Tokushima 770-8505, Japan
Abstract
The third-generation synthesis of (–)-heliannuol E has been accomplished employing mercuric triflate catalyzed arylene cyclization as the key step.Heliannuol E (1),1 an irregular sesquiterpenoid exhibiting allelopathic activity, was isolated from the extracts of Helianthus annuus L. cv. SAH-222® by Macías and co-workers. This natural product possesses a characteristic 4-vinylchroman skeleton with two tertiary stereogenic centers at the C2 and C4 positions. The absolute configurations were established to be 2R and 4R by the synthesis of Nishiyama and co-workers.2b Because of its fascinating structural features and allelopathic activity, three enantioselective2 and two racemic syntheses3 have been reported. One of the drawbacks in the previous enantioselective syntheses2 is the introduction of the vinyl moiety at the benzylic position; that is, the use of a stoichiometric amount of o-nitrophenylselenocyanate for the dehydration of the primary alcohol.4 In a recent publication, Nishizawa and co-workers demonstrated that the mercury(II) trifluoromethanesulfonate [Hg(OTf)2]5 catalyzed arylene cyclization6 proceeded cleanly to give the six-membered carbocycle fused to aryl and indole rings with a vinyl moiety at the benzylic position. We thought that this transformation could be used to construct the vinylchroman backbone of heliannuol E. Herein we report the synthesis of (–)-heliannuol E (1) using a Hg(OTf)2 catalyzed arylene cyclization as the key step. Approaching the synthesis from a retrosynthetic perspective, we envisioned the following scheme: (–)-1 would be derived from the bicyclic ester (2R,4R)-2 by a sequential Grignard reaction and demethylation. The ester 2 would be obtained by the C2-epimerization of (2S,4R)-3a, which would be prepared from the optically active allyl alcohol (S)-5 with an acyl moiety containing a chiral auxiliary (Xc) by the Hg(OTf)2 catalyzed cyclization followed by methanolysis of the resulting 4a,b. We chose (S)-5 as a substrate based upon mechanistic consideration of the possible transition states in the key cyclization (shown in Scheme 3), in which the product with a cis relationship between the two substituents at the C2 and C4 positions would predominate. Since the C2 stereogenic center can be epimerized, the major diastereoisomer would be converted to (–)-1. The allyl alcohol 5 could be derived from 4-methoxy-3-methylphenol (6). (Scheme 1)
As the chiral auxiliary for the creation of the tertiary stereogenic center at the future C2 (chroman numbering), we chose the Evans’ bromoacetyloxazolidinone 7.7 The phenol 68 was reacted with 7 in the presence of NaH to produce the ether 8 which was treated with the allylic iodide 99 and LiHMDS as a base10,11 to give 10 with high diastereoselectivity (>99 %de). After desilylation, the allyl alcohol 11, the substrate for the key conversion, was treated with 2 mol% of Hg(OTf)2 in toluene (0.05 M solution) at 90 °C for 2 h. The resulting mixture of cyclized products, which was immediately exposed to a methanol solution containing 4-DMAP at rt for 2 h,11 provided the expected 3 (cis:trans=3:1) and the regioisomer 12 (dr=10:1) in 43% and 8% yield, respectively. Both could be separated by silica gel column chromatography and were obtained as an inseparable mixture of diastereoisomers. Fortunately, 3 was obtained as a crystalline solid and recrystallization from hexane produced 3a in 31% yield (from 11), the X-ray crystallographic analysis12 of which showed that the structure of the major diastereoisomer was (2S,4R)-3a, as we had predicted. (Scheme 2)
The diastereoselectivity can be explained by comparing the possible π-complex6 transition states T1 and T2. In transition state T2, the steric repulsion between the acyl moiety (COXc) and the complexed mercury can be observed, and the sterically less demanding transition state T1 should predominate with cis-4a being generated as the major product. (Scheme 3)
Next we examined the epimerization at C2 of 3a. Treatment of 3a with DBU at 90 °C for 1h13 provided the recovered 3a and two new products, the epimerized 2 and the exocyclic alkene 13,14 in a ratio of 1:0.7:0.9 in 79% yield. Cis-3a was separated by preparative HPLC;15a however, 2 and 13 were inseparable. A mixture of 2 and 13 was reacted with MeMgBr in THF and still gave an inseparable mixture of the tertiary alcohols 14 and 15 (ca. 1:1) quantitatively. Final demethylation3b,16 was realized by sequential treatment with tris(pentafluorophenyl)borane/triethylsilane and TBAF to give a separable15b mixture of (–)-heliannuol E 1 and the reduced product 16 (dr=4:1, inseparable)17,18 in 33% and 10% yield, respectively, along with unidentified products which would be generated from 15. The spectral properties and the optical rotation {[α]D –88.5 (c 0.45, CHCl3); lit.2b [α]D –77.1 (c 0.10, CHCl3), lit.2c [α]D –76.5 (c 0.08, CHCl3)} of the synthetic 1 were identical with those of the natural product. (Scheme 4)
In summary, we have completed the enantioselective total synthesis of (–)-heliannuol E (1), which is the third-generation synthesis from our group, using a Hg(OTf)2 catalyzed arylene cyclization as the key step. This is the first example of a diastereoselective Nishizawa cyclization for the synthesis of a chroman derivative. The synthetic route developed here can be applied to the synthesis of other heliannane sesquiterpenoids.
ACKNOWLEDGEMENTS
This work was supported in part by a Grant-in-Aid program for the Promotion of Basic and Applied Research for Innovations in Bio-oriented Industry.
References
1. F. A. Macías, R. M. Varela, A. Torres, and J. M. G. Molinillo, Tetrahedron Lett., 1999, 40, 4725. CrossRef
2. (a) K. Sato, T. Yoshimura, M. Shindo, and K. Shishido, J. Org. Chem., 2001, 66, 309; CrossRef (b) F. Doi, T. Ogamino, T. Sugai, and S. Nishiyama, Tetrahedron Lett., 2003, 44, 4877; CrossRef (c) T. Kamei, M. Shindo, and K. Shishido, Synlett, 2003, 2395. CrossRef
3. (a) F. Doi, T. Ogamino, T. Sugai, and S. Nishiyama, Synlett, 2003, 411; CrossRef (b) J. R. Vyvyan, J. M. Oaksmith, B. W. Parks, and E. M. Peterson, Tetrahedron Lett., 2005, 46, 2457. CrossRef
4. P. A. Grieco, S. Gilman, and M. Nishizawa, J. Org. Chem., 1976, 41, 1485. CrossRef
5. For reviews, see: (a) M. Nishizawa and H. Imagawa, J. Synth. Org. Chem. Japan, 2006, 64, 744; (b) M. Nishizawa, ‘Electronic Encyclopedia of Reagents for Organic Synthesis’, (e-EROS), ed. by L. A. Paquette, John Wiley & Sons, Ltd, West Sussex, UK, (2007), .
6. K. Namba, H. Yamamoto, I. Sasaki, K. Mori, H. Imagawa, and M. Nishizawa, Org. Lett., 2008, 10, 1767. CrossRef
7. D. A. Evans and A. E. Weber, J. Am. Chem. Soc., 1987, 109, 7151. CrossRef
8. F. M. Hauser and D. Ganguly, J. Org. Chem., 2000, 65, 1842. CrossRef
9. M. Shindo, T. Sugioka, Y. Umaba, and K. Shishido, Tetrahedron Lett., 2004, 45, 8863. CrossRef
10. M. T. Crimmins, K. A. Emmitte, and J. D. Katz, Org. Lett., 2000, 2, 2165. CrossRef
11. H. Kim, H. Lee, D. Lee, S. Kim, and D. Kim, J. Am. Chem. Soc., 2007, 129, 2269. CrossRef
12. Crystal data of 3a: C15H18O4, formula weight 262.30, mp 96-96.5 °C, orthorhombic, space group P212121, a = 7.6220(6) Å, b = 7.9760(6) Å, c = 22.853(2) Å, V = 1389.3(2) Å3, Z = 4, Dcalc = 1.254 g/cm3, F(000) = 560.00, crystal dimensions = 0.80x0.70x0.50 mm, number of observations (all reflections) = 12440, number of variables = 190, reflection/parameter ratio = 65.47, R (all reflections) = 0.075, R (I>2.00σ(I)) = 0.073, Rw (all reflections) =0.148. Crystallographic data (excluding structure factors) for the structures in this paper have been deposited with the Cambridge Crystallographic Data Centre as supplementary publication number CCDC 714678. Copies of the data can be obtained, free of charge, on application to CCDC, 12 Union Road, Cambridge CB2 1EZ, UK [fax: +44(0) 1223 336033 or e-mail: deposit@ccdc.cam.ac.uk] .
13. T. Yoshimura, T. Bando, M. Shindo, and K. Shishido, Tetrahedron Lett., 2004, 45, 9241. CrossRef
14. From the 1HNMR of a mixture of 2 and 13, it was revealed that 13 was obtained as a single product. The stereochemistry was determined to be E from NOE spectral correlations (between the olefin proton and the C5-aromatic proton) as shown in Scheme 4 .
15. Conditions of HPLC separation: (a) Column, Mightysil 60 250-20; eluent, hexane/AcOEt=9:1; flow rate, 5 ml/min; (b) Column, Mightysil 60 250-20; eluent, hexane/AcOEt=7:3; flow rate, 5 ml/min.
16. V. Gevorgyan, M. Rubin, S. Benson J. X. Liu, and Y. Yamamoto, J. Org. Chem., 2000, 65, 6179. CrossRef
17. Compound 16 would be generated from 15 by treatment with B(C6F5)3 and Et3SiH. An example of the similar transformation has been reported by Wanner, et al., see: C. E. Hoesl, G. Höfner, and T. T. Wanne, Tetrahedron, 2004, 60, 307. CrossRef
18. Hydrogenation of 1 (H2, 5% Pd-C, EtOH, rt, 0.5 h) provided the reduced product (51%), dihydroheliannuol E, which was identical with the minor diastereomer of 16. It was clarified that the stereochemistry of the major diasteromer was cis, however, the absolute structure is unknown.