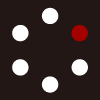
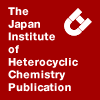
HETEROCYCLES
An International Journal for Reviews and Communications in Heterocyclic ChemistryWeb Edition ISSN: 1881-0942
Published online by The Japan Institute of Heterocyclic Chemistry
e-Journal
Full Text HTML
Received, 22nd January, 2009, Accepted, 16th February, 2009, Published online, 17th February, 2009.
DOI: 10.3987/COM-09-S(S)6
■ Tin-Hydride-Mediated Radical Addition of Alkyl Halides to 2-Methylene-1,3-dithiane Monoxide as a Ketene Equivalent
Suguru Yoshida, Hideki Yorimitsu,* and Koichiro Oshima*
Graduate School of Science, Kyoto University, Sakyo, Kyoto 606-8502, Japan
Abstract
Reductive radical addition of alkyl halides to ketene dithioacetal monoxide in the presence of tributyltin hydride and a radical initiator provides the corresponding adducts, 2-alkyl-1,3-dithiane monoxides, in good yields.INTRODUCTION
Intermolecular addition of alkyl radicals to activated alkenes mediated by tin hydride is one of the most important methodologies for carbon–carbon bond formation via radical processes.1 α,β-Unsaturated carbonyl compounds, unsaturated nitriles, and styrenes are usually employed as radical acceptors.
We have been interested in ketene dithioacetal and its derivatives as useful ketene equivalents in organic synthesis.2 Recently, we disclosed that ketene dithioacetal monoxides 1 serve as activated alkenes in rhodium-catalyzed addition of arylboronic acids.3 We anticipated that ketene dithioacetal monoxides 1 could behave as activated alkenes also in intermolecular radical addition. The captodative effect4 from the sulfanyl and sulfinyl groups should stabilize radical intermediate 2, facilitating the radical addition (Scheme 1). The stability of intermediate 2 could prevent undesired side reactions such as polymerization. Here, we report the full details5 of tin-hydride-mediated radical addition of alkyl halides to ketene dithioacetal monoxide 1 as a ketene equivalent.6
RESULTS AND DISCUSSION
Tributyltin hydride (2.0 equiv) was added dropwise over 1 h to a benzene solution of tert-butyl bromide (3.0 equiv), 2-methylene-1,3-dithiane 1-oxide (1a, 1.0 equiv), and 2,2’-azobis(isobutyronitrile) (AIBN, 0.10 equiv) at reflux (Table 1, entry 1). The resulting mixture was stirred for an additional 1 h to afford 3a in 84% isolated yield. Thanks to the highly polar nature of 3a, sulfoxide 3a could be separated by silica-gel column purification without contamination by tin residues.
It is worth noting that the reaction with acyclic ketene dithioacetal monoxide 1b yielded a complex mixture including polymeric materials (Figure 1). Phenyl-substituted 1c failed to participate in the radical addition, probably because of the steric reason. Treatment of tert-butyl bromide with 2-methylene-1,3-dithiane (4) provided the corresponding adduct in only 43% yield.
The use of triphenyltin hydride instead of tributyltin hydride allowed us to decrease the amount of alkyl halide employed. Addition of triphenyltin hydride (2.0 equiv) dropwise over 1 h to a solution of tert-butyl bromide (1.0 equiv), 1a (1.2 equiv), and AIBN (0.20 equiv) furnished 3a in 70% yield (Equation 1). Smoother hydrogen abstraction of the stabilized radical intermediate 2 from triphenyltin hydride would increase the efficiency of the reaction, because of the weaker Sn−H bond of triphenyltin hydride.7
We next investigated the scope of alkyl halides (Table 1). The addition of tertiary alkyl bromides proceeded smoothly to yield the desired products in high yields (entries 1–4). The phenylthio group was compatible under the reaction conditions (entry 4). Secondary alkyl iodides also underwent the radical reaction smoothly with 1a, although the products were mixtures of two diastereomers (entries 5 and 6). Primary alkyl iodide reacted with 1a to provide 3g in moderate yield with lower diastereoselectivity. In all cases, trans isomers were predominantly formed.8 The reason for the high trans selectivity is not clear.
We next examined the utility of the products. Treatment of sulfoxide 3b with a catalytic amount of sulfuric acid in boiling toluene afforded aldehyde 5 almost quantitatively (Equation 2). Sulfoxide 3b could be easily reduced into 1,3-dithiane.9 Thus, treatment of sulfoxide 3b with trifluoroacetic anhydride and sodium iodide gave 1,3-dithiane 6 in excellent yield (Equation 3).
The reaction of tertiary alkyl bromide 7 bearing an amide moiety10 with 1a and tributyltin hydride proceeded efficiently to give desired adduct 8 in high yield (Scheme 2). Treatment of adduct 8 with hydrochloric acid followed by an addition of benzoyl chloride provided dihydropyrrole 9 having a quaternary carbon atom in good yield. The present protocol would be useful for the synthesis of heterocycles bearing such a quaternary carbon moiety.
In conclusion, we have developed radical addition of alkyl halides to a ketene equivalent, 2-methylene-1,3-dithiane 1-oxide (1a), in the presence of tributyltin hydride and AIBN. The reaction afforded alkanal equivalents protected as dithioacetal monoxide, which could be subjected to a variety of organic transformations.
EXPERIMENTAL
Instrumentation and Chemicals
1H NMR (500 MHz) and 13C NMR (126 MHz) spectra were taken on Varian UNITY INOVA 500 spectrometers and were recorded in CDCl3. Chemical shifts (δ) are in parts per million relative to tetramethylsilane at 0.00 ppm for 1H and relative to CDCl3 at 77.23 ppm for 13C unless otherwise noted. IR spectra were determined on a SHIMADZU FTIR-8200PC spectrometer. Mass spectra (FAB unless otherwise noted) were determined on a JEOL MStation 700 spectrometer. TLC analyses were performed on commercial glass plates bearing 0.25-mm layer of Merck Silica gel 60F254. Silica gel (Wakogel 200 mesh) was used for column chromatography. Elemental analyses were carried out at the Elemental Analysis Center of Kyoto University.
Unless otherwise noted, materials obtained from commercial suppliers were used without further purification. Tributyltin hydride and triphenyltin hydride were purchased from Aldrich. Benzene was purchased from Wako Pure Chemical and dried over slices of sodium. Ketene dithioacetal monoxides 111 and dithiane 42 were prepared according to the literature. Most of alkyl halides and AIBN were purchased from Wako Pure Chemical. 2-Bromo-2-methyldecane12 (Table 1, entry 2) and 6-bromo-6-methyl-1-phenylthioheptane13 (entry 4) were prepared by bromination of the corresponding alcohol with hydrobromic acid. Alkyl halide 7 was prepared according to the literature.10 All reactions were carried out under argon atmosphere.
Typical Procedure for Radical Addition Reaction (Table 1, entry 1): A benzene (1.0 mL) solution of 2-methylene-1,3-dithiane 1-oxide (1a, 49.4 mg, 0.33 mmol), 2-bromo-2-methylpropane (0.11 mL, 0.99 mmol), and 2,2’-azobis(isobutyronitrile) (5.0 mg, 0.030 mmol) was placed in a flask under an atmosphere of argon. Then, a benzene (1.0 mL) solution of tributyltin hydride (0.18 mL, 0.66 mmol) was added over 1 h with a syringe pump at reflux. After the addition was completed, the mixture was stirred for an additional 1 h at the same temperature. The reaction mixture was poured into saturated aqueous NaHCO3 (5 mL) and extracted with AcOEt (3 × 10 mL). The combined organic layer was dried over anhydrous Na2SO4 and concentrated in vacuo. Purification by chromatography on silica gel (hexane/AcOEt = 1/2) provided 2-(2,2-dimethylpropyl)-1,3-dithiane 1-oxide (3a, 58.0 mg, 0.28 mmol, 84%).
Transformation of 3b to 5: A toluene (2.0 mL) solution of 2-(2,2-dimethyldecyl)-1,3-dithiane 1-oxide (3b, 61.6 mg, 0.20 mmol) was placed in a flask under an atmosphere of argon. Then, sulfuric acid (7.1 mg, 0.072 mmol) was added, and the resulting mixture was stirred at reflux for 2 h. The reaction mixture was poured into sat. aq NaHCO3 (5 mL) and extracted with AcOEt (3 × 10 mL). The combined organic layer was dried over anhydrous Na2SO4 and concentrated in vacuo. Purification by chromatography on silica gel (hexane/AcOEt = 40/1) provided 3,3-dimethylundecanal (5, 39.6 mg, 0.20 mmol, 99%).
Transformation of 3b to 6: A solution of 2-(2,2-dimethyldecyl)-1,3-dithiane 1-oxide (3b, 30.2 mg, 0.10 mmol) and sodium iodide (38.3 mg, 0.25 mmol) in acetone (2.0 mL) was placed in a flask under argon. Trifluoroacetic anhydride (0.042 mL, 0.30 mmol) was then added dropwise at 0 °C, and the resulting mixture was stirred at 25 °C for 1 h. The reaction mixture was poured into sat. aq NaHCO3 (5 mL) and extracted with AcOEt (3 × 10 mL). The combined organic layer was dried over anhydrous Na2SO4 and concentrated in vacuo. Chromatographic purification on silica gel (hexane/AcOEt = 40/1) yielded 2-(2,2-dimethyldecyl)-1,3-dithiane (6, 26.3 mg, 0.091 mmol, 92%).
Synthesis of 8: A benzene (1.0 mL) solution of 2-methylene-1,3-dithiane 1-oxide (1a, 44.6 mg, 0.30 mmol), tert-butyl N-(2-bromo-2-ethylbutyl)carbamate (7, 250 mg, 0.90 mmol), and 2,2’-azobis(isobutyronitrile) (5.0 mg, 0.030 mmol) was placed in a flask under an atmosphere of argon. Tributyltin hydride (0.18 mL, 0.60 mmol) in benzene (1.0 mL) was then added over 1 h with a syringe pump at reflux. After the addition was completed, the mixture was stirred for an additional 1 h at the same temperature. The reaction mixture was poured into sat. aq NaHCO3 (5 mL) and extracted with AcOEt (3 × 10 mL). The combined organic layer was dried and concentrated. Silica gel column purification by using hexane/AcOEt = 1/2 as eluent afforded 2-[2-(tert-butoxycarbonylaminomethyl)-2- ethylbutyl]-1,3-dithiane 1-oxide (8, 95.4 mg, 0.27 mmol, 90%).
Synthesis of 9: An acetonitrile (5.0 mL) solution of 2-[2-(tert-butoxycarbonylaminomethyl)-2-ethyl butyl]-1,3-dithiane 1-oxide (8, 59.0 mg, 0.17 mmol) was placed in a flask under argon atmosphere. Hydrochloric acid (0.31 mL, 11 M, 3.4 mmol) was added at 25 °C, and the resulting mixture was stirred at the same temperature for 48 h. Benzoyl chloride (0.096 mL, 0.82 mmol) was then added at 25 °C, and the resulting mixture was stirred at the same temperature for 12 h. The reaction mixture was poured into sat. aq NaHCO3 (5 mL). Extractive workup followed by silica gel column purification (hexane/AcOEt = 10/1) provided N-benzoyl-3,3-diethyl-2,3-dihydropyrrole (9, 23.8 mg, 0.10 mmol, 62%).
Characterization Data of Compounds
2-(2,2-dimethylpropyl)-1,3-dithiane 1-oxide (3a)
IR (neat) 2955, 2907, 2868, 2844, 1474, 1425, 1367, 1241 cm–1; 1H NMR (CDCl3) δ 1.05 (s, 9H), 1.41 (dd, J = 14.5, 4.5 Hz, 1H), 2.25–2.34 (m, 1H), 2.37 (d, J = 14.5 Hz, 1H), 2.45–2.50 (m, 1H), 2.53–2.58 (m, 1H), 2.67 (ddd, J = 10.0, 10.0, 2.0 Hz, 1H), 2.75 (dd, J = 12.0, 12.0 Hz, 1H), 3.40–3.45 (m, 1H), 3.56 (d, J = 9.5 Hz, 1H); 13C NMR (CDCl3) δ 29.4, 30.2, 30.6, 31.4, 42.1, 53.9, 63.0. Anal. Calcd for C9H18OS2: C, 52.38; H, 8.79%. Found: C, 52.55; H, 8.75%.
2-(2,2-dimethyldecyl)-1,3-dithiane 1-oxide (3b)
IR (neat) 2925, 2853, 1468, 1425, 1389, 1367, 1171, 1038, 870, 830, 404 cm–1; 1H NMR (CDCl3) δ 0.87 (t, J = 7.0 Hz, 3H), 0.97 (s, 3H), 0.98 (s, 3H), 1.21–1.36 (br, 14 H), 1.41 (dd, J = 15.0, 5.0 Hz, 1 H), 2.20–2.34 (m, 2H), 2.40–2.58 (m, 2H), 2.64 (ddd, J = 13.0, 13.0, 3.0 Hz, 1H), 2.72 (dd, J = 12.0, 12.0 Hz, 1H), 3.39 (br d, J = 12.0 Hz, 1H), 3.53 (d, J = 9.0 Hz, 1H); 13C NMR (CDCl3) δ 14.2, 22.8, 24.1, 27.6, 27.9, 29.2, 29.5, 29.8, 30.4, 30.6, 32.0, 33.7, 40.2, 42.8, 53.9, 62.9. HRMS (FAB+) (m/z) Observed: 305.1979 (Δ = +1.9 ppm). Calcd for C16H33OS2 [MH+]: 305.1973.
2-(1-adamantylmethyl)-1,3-dithiane 1-oxide (3c, the major diastereomer)
Mp 130–131 °C; IR (nujol) 2922, 2853, 1685, 1654, 1559, 1507, 1378, 1279, 1024 cm–1; 1H NMR (CDCl3) δ 1.27 (dd, J = 15.0, 4.5 Hz, 1H), 1.58–1.75 (m, 12 H), 1.99 (br s, 3H), 2.20 (d, J = 15.0 Hz, 1H), 2.23–2.33 (m, 1H), 2.42–2.56 (m, 2H), 2.65 (ddd, J = 13.0, 13.0, 2.0 Hz, 1H), 2.75 (dd, J = 13.0, 13.0 Hz, 1H), 3.38–3.44 (m, 1H), 3.59 (d, J = 10.0 Hz, 1H); 13C NMR (CDCl3) δ 28.7, 29.3, 30.5, 33.1, 37.0, 42.8, 42.9, 53.8, 61.2. HRMS (FAB+) (m/z) Observed: 284.1268 (Δ = –0.2 ppm). Calcd for C15H24OS2: 284.1269.
2-(7-phenylthio-2,2-dimethylheptyl)-1,3-dithiane 1-oxide (3d)
IR (neat) 2921, 2853, 1584, 1480, 1471, 1438, 1425, 1367, 1092, 1038, 740, 691 cm–1; 1H NMR (CDCl3) δ 0.99 (s, 6H), 1.26–1.36 (m, 4H), 1.37–1.44 (m, 3H), 1.62–1.70 (m, 2H), 2.22–2.36 (m, 2H), 2.42–2.48 (m, 1H), 2.50–2.55 (m, 1H), 2.65 (ddd, J = 10.0, 10.0, 2.0 Hz, 1H), 2.73 (dd, J = 12.0, 12.0 Hz, 1H), 2.93 (t, J = 7.0 Hz, 2H), 3.38–3.43 (m, 1H), 3.53 (d, J = 8.0 Hz, 1H), 7.15–7.19 (m, 1H), 7.26–7.30 (m, 2H), 7.31–7.35 (m, 2H); 13C NMR (CDCl3) δ 23.6, 27.7, 27.8, 29.3, 29.7, 30.5, 33.7, 33.8, 40.1, 42.5, 53.9, 62.7, 125.9, 129.1, 129.2, 137.3. HRMS (FAB+) (m/z) Observed: 370.1462 (Δ = +0.9 ppm). Calcd for C19H30OS3: 370.1459.
2-(cyclohexylmethyl)-1,3-dithiane 1-oxide (3e, the major diastereomer)
Mp 93–94 °C; IR (nujol) 2920, 2853, 1685, 1559, 1507, 1447, 1375, 1023 cm–1; 1H NMR (CDCl3) δ 0.85–1.45 (m, 6H), 1.46–1.76 (m, 5H), 1.80–1.86 (m, 1H), 2.20–2.32 (m, 2H), 2.42–2.48 (m, 1H), 2.52–2.70 (m, 3H), 3.38–3.44 (m, 1H), 3.65 (dd, J = 11.0, 3.5 Hz, 1H); 13C NMR (CDCl3) δ 26.1, 26.3, 26.5, 29.5, 30.1, 32.1, 34.1, 34.2, 36.3, 54.0, 64.1. Anal. Calcd for C11H20OS2: C, 56.85; H, 8.67%. Found: C, 56.62; H, 8.39%.
2-(2-methylpropyl)-1,3-dithiane 1-oxide (3f, the major diastereomer)
IR (neat) 2957, 2911, 2869, 2826, 1469, 1426, 1368, 1034, 682, 657 cm–1; 1H NMR (CDCl3) δ 0.97 (d, J = 6.5 Hz, 3H), 1.01 (d, J = 7.0 Hz, 3H), 1.51–1.60 (m, 1H), 1.92–2.02 (m, 1H), 2.16–2.34 (m, 2H), 2.43–2.49 (m, 1H), 2.54–2.72 (m, 3H), 3.38–3.46 (m, 1H), 3.63 (dd, J = 11.0, 3.5 Hz, 1H); 13C NMR (CDCl3) δ 21.4, 23.6, 25.0, 29.5, 30.1, 37.8, 54.0, 64.6. Anal. Calcd for C8H16OS2: C, 49.96; H, 8.38%. Found: C, 50.06; H, 8.35%.
2-heptyl-1,3-dithiane 1-oxide (3g, the major diastereomer)
IR (neat) 2955, 2926, 2855, 1466, 1426, 1231, 1036, 754, 725, 698, 663 cm–1; 1H NMR (CDCl3) δ 0.88 (t, J = 7.0 Hz, 3H), 1.25–1.50 (m, 9H), 1.62–1.77 (m, 2H), 2.22–2.35 (m, 2H), 2.42–2.49 (m, 1H), 2.57–2.73 (m, 3H), 3.40 (m, 1H), 3.60 (dd, J = 9.5 Hz, 3.5 Hz, 1H); 13C NMR (CDCl3) δ 14.3, 22.8, 26.0, 28.9, 29.2, 29.5, 29.7, 30.2, 31.9, 54.1, 66.5. HRMS (FAB+) (m/z) Observed: 234.1112 (Δ = 0.0 ppm). Calcd for C11H22OS2: 234.1112.
3,3-dimethylundecanal (5)
IR (neat) 2958, 2928, 2854, 2729, 1723, 1468, 1368, 1267 cm–1; 1H NMR (CDCl3) δ 0.89 (t, J = 7.0 Hz, 3H), 1.04 (s, 6H), 1.23–1.36 (br, 14H), 2.25 (d, J = 3.0 Hz, 2H), 9.84 (t, J = 3.0 Hz, 1H); 13C NMR (CDCl3) δ 14.3, 22.9, 24.2, 27.8, 29.5, 29.8, 30.5, 32.1, 33.7, 43.0, 55.0, 204.0. HRMS (m/z) Observed: 197.1909 (Δ = +1.8 ppm). Calcd for C13H25O [M–H]+: 197.1905.
2-(2,2-dimethyldecyl)-1,3-dithiane (6)
IR (neat) 2927, 2900, 2853, 1559, 1507, 1458, 1275 cm–1; 1H NMR (CDCl3) δ 0.89 (t, J = 7.0 Hz, 3H), 0.95 (s, 6H), 1.23–1.32 (m, 14H), 1.58 (d, J = 5.5 Hz, 2H), 1.76–1.86 (m, 1H), 2.05–2.12 (m, 1H), 2.77–2.82 (m, 2H), 2.92–2.99 (m, 2H), 4.04 (t, J = 5.5 Hz, 1H); 13C NMR (CDCl3) δ 14.4, 22.9, 24.3, 25.6, 27.6, 29.6, 29.9, 30.7, 31.6, 32.1, 34.0, 42.6, 43.5, 48.3. HRMS (m/z) Observed: 288.1941 (Δ = –1.5 ppm). Calcd for C16H32S2: 288.1945.
2-[2-(tert-butoxycarbonylaminomethyl)-2-ethylbutyl]-1,3-dithiane 1-oxide (8)
IR (neat) 3307, 2880, 1683, 1505, 1366, 1250, 1161, 1022 cm–1; 1H NMR (CDCl3) δ 0.84 (t, J = 7.5 Hz, 6H), 1.15–1.40 (m, 5H), 1.41 (s, 9H), 2.18–2.31 (m, 2H), 2.42–2.56 (m, 2H), 2.59–2.66 (m, 1H), 2.72–2.84 (m, 2H), 3.35 (dd, J = 14.5, 9.0 Hz, 1H), 3.41–3.50 (m, 2H), 5.80 (br s, 1H); 13C NMR (CDCl3) δ 7.4, 7.6, 25.5, 26.5, 28.6, 29.6, 31.1, 34.5, 40.5, 44.2, 54.3, 60.4, 78.8, 156.7. HRMS (m/z) Observed: 349.1742 (Δ = –1.1 ppm). Calcd for C16H31O3NS2: 349.1746.
N-benzoyl-3,3-diethyl-2,3-dihydropyrrole (9)
IR (neat) 2963, 2922, 1610, 1578, 1448, 1406, 1374, 831, 716, 700 cm–1; 1H NMR (CDCl3) δ 0.90 (t, J = 7.5 Hz, 6H), 1.48–1.56 (m, 4H), 3.74 (s, 2H), 4.99 (d, J = 4.0 Hz, 1H), 6.43 (d, J = 4.0 Hz, 1H), 7.40–7.46 (m, 3H), 7.48–7.52 (m, 2H); 13C NMR (CDCl3) δ 8.8, 31.4, 49.8, 55.0, 119.2, 128.0, 128.6, 129.6, 130.5, 135.8, 167.1. HRMS (m/z) Observed: 229.1466 (Δ = –0.2 ppm). Calcd for C15H19NO: 229.1466.
ACKNOWLEDGEMENTS
This work was supported by Grants-in-Aid for Scientific Research and Global COE Research Programs from JSPS. S.Y. acknowledges JSPS for financial support.
References
1. D. P. Curran, Synthesis, 1988, 417; CrossRef B. Giese, ‘Radicals in Organic Synthesis: Formation of Carbon–Carbon Bonds,’, Pergamon Press, Oxford, U.K., 1986, , Chapter 3; S. Z. Zard, ‘Radical Reactions in Organic Synthesis,’, Oxford University Press, Oxford, U.K., 2003, , Chapter 2; H. Togo, ‘Advanced Free Radical Reactions for Organic Synthesis,’ , Elsevier, Oxford, U.K., 2004, , Chapter 4.
2. S. Yoshida, H. Yorimitsu, and K. Oshima, J. Organomet. Chem., 2007, 692, 3110; CrossRef S. Yoshida, H. Yorimitsu, and K. Oshima, Org. Lett., 2007, 9, 5573; CrossRef S. Yoshida, H. Yorimitsu, and K. Oshima, Chem. Lett., 2008, 37, 786. CrossRef
3. S. Yoshida, H. Yorimitsu, and K. Oshima, Synlett, 2007, 1622; CrossRef S. Yoshida, H. Yorimitsu, and K. Oshima, Heterocycles, 2008, 76, 679. CrossRef
4. H. G. Viehe, Z. Janousek, and R. Merenyi, Acc. Chem. Res., 1985, 18, 148. CrossRef
5. A part of this work was communicated: S. Yoshida, H. Yorimitsu, and K. Oshima, Chem. Lett., 2009, 38, in press. CrossRef
6. Radical addition to ketene and its related compounds is rare and difficult to control: W. Huang, H. Henry-Riyad, and T. T. Tidwell, J. Am. Chem. Soc., 1999, 121, 3939; CrossRef K. Sung and T. T. Tidwell, J. Org. Chem., 1998, 63, 9690; CrossRef K. Ogura, N. Sumitani, A. Kayano, H. Iguchi, and M. Fujita, Chem. Lett., 1992, 1487. CrossRef
7. C. Chatgilialoglu and M. Newcomb, Adv. Organomet. Chem., 1999, 44, 67. CrossRef
8. The relative stereochemistry of 3 was determined by comparing the NMR spectra of 3 with those of closely related compounds. See: P. C. B. Page, R. D. Wilkes, E. S. Namwindwa, and M. J. Witty, Tetrahedron, 1996, 52, 2125. CrossRef
9. M. Greef and S. Z. Zard, Tetrahedron, 2004, 60, 7781. CrossRef
10. A. Sliwinska and A. Zwierzak, Tetrahedron, 2003, 59, 5927. CrossRef
11. D. Seebach, B. T. Grobel, A. K. Beck, M. Braun, and K. H. Geiss, Angew. Chem., Int. Ed. Engl., 1972, 11, 443; CrossRef R. Kaya and N. R. Beller, J. Org. Chem., 1981, 46, 196; CrossRef F. Sandrinelli, G. Fontaine, S. Perrio, and P. Beslin, J. Org. Chem., 2004, 69, 6916. CrossRef
12. H. Ohmiya, T. Tsuji, H. Yorimitsu, and K. Oshima, Chem. Eur. J., 2004, 10, 5640. CrossRef
13. M. Sai, H. Someya, H. Yorimitsu, and K. Oshima, Org. Lett., 2008, 10, 2545. CrossRef