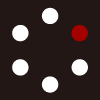
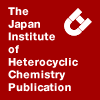
HETEROCYCLES
An International Journal for Reviews and Communications in Heterocyclic ChemistryWeb Edition ISSN: 1881-0942
Published online by The Japan Institute of Heterocyclic Chemistry
e-Journal
Full Text HTML
Received, 28th January, 2009, Accepted, 5th March, 2009, Published online, 9th March, 2009.
DOI: 10.3987/COM-09-S(S)7
■ Efforts toward the Synthesis of Pseudolaric Acid A: Intramolecular Bromoetherification as a Multipurpose Synthetic Tool
Jeremy D. Pettigrew and Leo A. Paquette*
Evans Chemical Laboratories, The Ohio State University, 120 W. 18th Avenue, Columbus, Ohio 43210, U.S.A.
Abstract
Regioselective double bond cleavage within 5, required for access to the trans-fused perhydroazulene framework of pseudolaric acid A (1), has been accomplished via a highly diastereoselective intramolecular bromoetherification process, the latter serving a dual role as a protecting group tactic.INTRODUCTION
The oxygenated diterpene pseudolaric acid A (1),1 initially discovered to possess antifungal and antifertility properties,2 has more recently been shown to exhibit exciting cytotoxic potential.3 Our retrosynthetic conceptualization for construction of the uncommon trans-fused perhydroazulene framework of the compact tricyclic core of 1 relies on the regioselective oxidative cleavage of the more highly strained olefinic linkage in 2, thereby revealing a direct means for installing the three contiguous tetrahedral carbon centers of 1 (Scheme 1). The precursor 2 was envisioned to originate from the previously described norbornenol 3.4
RESULTS AND DISCUSSION
Addition of the reagent prepared from 4-bromo-2-methylbut-1-ene5 and lithium metal to 3 in THF afforded the corresponding bridged alcohols in satisfactory yield (79%) albeit with moderate selectivity (dr = 5:2 anti:syn) (Scheme 2). The TBS group was removed with TBAF to liberate the corresponding diols, at which point the two diastereomers could be readily separated by column chromatography. Chemoselective oxidation of the anti alcohol with the Dess-Martin periodinane led efficiently to 4. Conversion of 4 into 5 was accomplished via the Wittig olefination involving the known ylide 66 followed by relay ring closing metathesis7 upon exposure of the resulting triene to the first-generation Grubbs catalyst in 69% overall yield. The selection of 6 instead of Ph3P=CH2, which would potentially lead to the same desired ruthenium carbene, was made in an effort to avoid ring opening polymerization metathesis of the norbornene moiety by reducing the steric congestion imparted by the bicycle during the initial ruthenium insertion. The correspondence in framework structure residing in 5 and 1 was now made apparent.
The anticipated direct regioselective cleavage of the more strained double bond in 5 proved unrealizable, as the undesired regioselectivity was observed. This unexpected result was attributed to steric crowding by the 7-membered ring in the vicinity of the more strained olefinic center. Consequently, the decision was made to mask this site of unsaturation. The first ploy to be attempted involved the use of an epoxide ring as a protecting group. Thus, alcohol 5 was allowed to react with one equivalent of m-CPBA to afford 7 (Scheme 3). As expected, the double bond of the 7-membered ring underwent smooth reaction to afford the single diastereomer 7 which was attributed to the directing ability of the free hydroxyl group. The remaining double bond in 7 was next dihydroxylated with OsO4. Cleavage of the resultant diol was accomplished with Pb(OAc)4 to deliver 9, which was found to be an unstable compound whose ready degradation is possible by retro aldol reactions similar to the observations made in Trost’s reported synthesis of pseudolaric acid B.8 To circumvent these retro aldol reactions, the hydroxyl group resident in 7 was protected as the MOM ether, the double bond in 8 was dihydroxylated with OsO4 and the resultant diol was cleaved with NaIO4 to afford the stable aldehyde 10. The attempted direct oxidation of this aldehyde to the corresponding ester with Oxone afforded the mixed acetals 11 or 12 resulting from backside opening of the epoxide by the hemiacetal reaction intermediates.9 Formation of the carboxylic acid was next attempted. However, similar backside attack operated to furnish 13.
Our alternative strategy involved concomitant protection of the double bond and the hydroxyl moiety in 5 as a cyclic bromoether. To this end, alcohol 5 was reacted with NBS in t-BuOH in a darkened reaction flask to afford 14 as a single diastereomer in good yield (Scheme 4). The regiochemistry of this reaction was easily deduced from the HMQC NMR spectra, which showed that the 13C signal assigned to the ether carbon (δ 83.3 ppm) had no hydrogen atoms attached to it. Notably, the relative configurations inherent to this transformation product are seen to be entirely controlled by the stereochemistries of the starting material. The remaining double bond was dihydroxylated and the resulting diol was cleaved to afford the corresponding keto aldehyde. Oxidation of this aldehyde to the carboxylic acid, followed by O-methylation with diazomethane, afforded ester 15. Subsequent deprotection of 15 with zinc-copper couple gave rise to 16.
In conclusion, the implementation of a dual protecting group strategy based on the regio- and diastereoselective intramolecular formation of a bromo ether has allowed for the preparation of the trans-fused perhydroazulene framework of 1. We are currently investigating the completion of its total synthesis and we hope to report our results in due course.
ACKNOWLEDGEMENTS
JDP would like to acknowledge NSERC for a postdoctoral fellowship.
References
1. For synthetic efforts towards 1 see: Z. Geng, B. Chen, and P. Chiu, Angew. Chem. Int. Ed., 2006, 45, 6197; CrossRef B. Chen, R. Y. Y. Ko, M. S. M. Yuen, K. F. Cheng, and P. Chiu, J. Org. Chem., 2003, 68, 4195; CrossRef P. Chiu, B. Chen, and K. F. Cheng, Org. Lett., 2001, 3, 1721; CrossRef L. Ou, Y. Hu, G. Song, and D. Bai, Tetrahedron, 1999, 55, 13999; CrossRef Y. Hu, L.Ou, and D. Bai, Tetrahedron Lett., 1999, 40, 545; CrossRef P. Chiu, B. Chen, and K. F. Cheng, Tetrahedron Lett., 1998, 39, 9229; CrossRef B. C. Pan, H. Y. Chang, G. L. Cai, and Y. S. Guo, Pure Appl. Chem., 1989, 61, 389. CrossRef
2. (a) E. Li, A. M. Clark, and C. D. Hufford, J. Nat. Prod., 1995, 58, 57; CrossRef B. N. Zhou, B. P. Ying, G. Q. Song, Z. X. Chen, J. Han, and Y. F. Yan, Planta Med., 1983, 47, 35. CrossRef
3. (a) V. K. W. Wong, P. Chiu, S. S. M. Chung, L. M. C. Chow, Y. Z. Zhao, B. B. Yang, and B. C. B. Ko, Clin. Cancer Res., 2005, 11, 6002; CrossRef M. S. Jardat, D. J. Noonan, B. Wu, M. A. Avery, and D. R. Feller, Planta Med., 2002, 68, 667; CrossRef D. J. Pan, Z. L. Li, C. Q. Hu, K. Chen, J. J. Chang, and K. H. Lee, Planta Med., 1990, 56, 383; CrossRef M. O. Hamburger, H. L. Shieh, B. N. Zhou, J. M. Pezzuto, and G. A. Cordell, Magn. Reson. Chem., 1989, 27, 1025. CrossRef
4. L. A. Paquette and J. D. Pettigrew, Synthesis, 2009, 379. CrossRef
5. W. F. Berkowitz and Y. Wu, J. Org. Chem., 1997, 62, 1536. CrossRef
6. M. R. Pitts, J. R. Harrison, and C. J. Moody, J. Chem. Soc., Perkin Trans. 1, 2001, 955. CrossRef
7. P. A. Roethle, I. T. Chen, and D. Trauner, J. Am. Chem. Soc., 2007, 129, 8960; CrossRef D. J. Wallace, Angew. Chem. Int. Ed., 2005, 44, 1912; CrossRef X. Wang, E. J. Bowman, B. J. Bowman, and J. A. Porco, Jr., Angew. Chem. Int. Ed., 2004, 43, 3601; CrossRef T. R. Hoye, C. S. Jeffrey, M. A. Tennakoon, J. Wang, and H. Zhao, J. Am. Chem. Soc., 2004, 126, 10210. CrossRef
8. B. M. Trost, J. Waser, and A. Meyer, J. Am. Chem. Soc., 2008, 130, 16424; CrossRef B. M. Trost, J. Waser, and A. Meyer, J. Am. Chem. Soc., 2007, 129, 14556. CrossRef
9. B. R. Travis, M. Sivakumar, G. O. Hollist, and B. Borhan, Org. Lett., 2003, 5, 1031. CrossRef