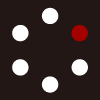
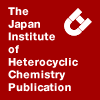
HETEROCYCLES
An International Journal for Reviews and Communications in Heterocyclic ChemistryWeb Edition ISSN: 1881-0942
Published online by The Japan Institute of Heterocyclic Chemistry
e-Journal
Full Text HTML
Received, 30th January, 2009, Accepted, 31st March, 2009, Published online, 1st April, 2009.
DOI: 10.3987/COM-09-11669
■ Regio and Stereo Selectivity on the α-Aryl-δ-epoxynitrile Anionic Cyclization Reactions: Modulation towards a 5-Endo or 4-Exo Process
Jesús Armando Luján-Montelongo, Adrián Vázquez-Sánchez, and José Gustavo Ávila-Zárraga*
Facultad de Química, Ciudad Universitaria, Universidad Nacional Autónoma de México, Mexico City, 04510, Mexico
Abstract
Here we present a study focused on the regio and stereoselectivity of the anionic intramolecular cyclization reactions of several 4-(3,3-dimethyloxiran-2-yl)-2-arylbutanonitriles. The first part comprises the exploration of the reaction varying the temperature/solvent as well as the base counter-ion; switching between Li, Na and K that companies hexamethyldisilylamide anion. This is a well-known base used widely. In the second part, we provide a pair of methodologies that lead almost exclusively to pentacarbocycles through a 5-endo pathway, although is known to be a non-favored process in many cases. We found that both the metal counter-ion and the temperature have an important effect on the regioselectivity of the reaction and, independently of the pathway presented, the preferred diastereoselectivity is trans.INTRODUCTION
Epoxides are intermediaries used commonly in the carbocyclic and heterocyclic syntheses, which are frequently the central moiety in compounds of biological and non-biological origin. One classic way to build these structures is by cyclization reactions of nucleophilic intramolecular displacement where the oxiranyl group participates as an electrophile. In such a way we found several examples where the use of heteroatom-based nucleophiles yields heterocycles.1 On the other hand, the use of carbon-based nucleophiles usually yields carbocycles.2
So, as a subtype of the last reaction type, we find a special circumstance in which epoxynitriles3 are used as precursors. It is important to mark that these types of intermediaries have been used in a very novel way in radical-type reactions.4 This protocol can achieve high regioselectivity because of the formation of the more stable radical species, leading to highly substituted C-C bonds. In most cases, the procedure occurs preferentially through an exo cyclization pathway5 and no evidence of a preference on an endo pathway based procedure have been published.
It’s a well-known fact, besides for a few selected cases6 that the endo pathway in intramolecular anionic cyclization of the epoxynitriles is not favored, exploiting this methodology only for the carbocyclic syntheses which go through an exo pathway7 (Scheme 1).
Undoubtedly, the difference in the regioselectivity of the epoxide opening arises in first place from the substitution degree of the oxiranyl function. Lallemand and Onanga6 were the first that complemented the Stork’s vision about the “collinearity requirements”8 of the nucleophilic attack as the main reason for the observed regioselectivity. Their argument was found only on steric factors9: δ-Epoxides bearing cis substituents generated cyclobutanes (through an exocyclic ring closure, minimizing the steric interactions in the transition state) and the complementary epoxides with trans substituted yielded cyclopentanes, mainly (through an endocyclic ring closure, where steric interactions are minimal through a staggered orientation).
Unfortunately, evidence for the regioselectivity prediction in cyclization reactions for trisubstituted epoxides was unclear for a long time.10 Ávila-Zárraga and Maldonado found that despite the Stork’s work-based prediction about the cyclobutane formation starting from α-substituted-δ-epoxynitriles, the specific case of α-tolyl-δ-epoxynitriles yielded mostly a cyclopentane structure. It was also evident that the so-called collinearity requirements were not the predominant factor in this reaction. The α-substituent of the δ-epoxynitrile had an important role too. On the other hand, Fleming and co-workers recently made a series of well accomplished works.9, 11 Now it can be concluded that the α-metalated nitriles are “chameleonic” species which can give regio, and stereochemically diverse consequences, depending on the experimental conditions as the counterions involved, solvents, additives, reaction temperature, etc.
Considering the epoxides as very reliable and easy-obtainable intermediaries, as of the potential of this methodology to give enantiomeric pure products because of the diastereoselectivity shown in many of the existent works, we show the study on six frameworks based on 2-aryl-6,6-dimethyl-5-epoxynitriles. We evaluate the regio and stereochemical effect of the reaction temperature and the metallic cation of the hexamethyldisilylamide anion, a well-known recurred base used to generate α-nitrile anionic species. We also provide a pair of methodologies that promote the cyclization reaction to go through a 5-endo pathway giving 1-aryl-2,2-dimethyl-cyclopentanecarbonitriles as main products, which could be potential precursors on the syntheses of some natural products of the cuparane family.12
RESULTS AND DISCUSSION
The syntheses of the 2-aryl-6,6-dimethyl-5-epoxynitriles 4a-f were accomplished by an alkylation-oxidation protocol. Extensive experimental exploration of the alkylation reaction of the corresponding nitrile131a-f with the alkenyl iodide 5-iodo-2-methyl-pent-2-ene14 2 showed that the use of the lithium hexamethyldisilylamide base in THF at -78 °C, usually give the best yields. Epoxidation of the alkylated products on the homoisoprenylic residue were then carried out employing MCPBA or dimethyldioxirane as oxidants. Both groups of alkylated nitriles 3a-f and epoxynitrile compounds 4a-f were completely characterized by spectroscopic analyses.15
Once we obtained the epoxynitrile compounds 4a-f, we then proceed to the study of the intramolecular cyclization reaction using the hexamethyldisilylamide anion in benzene and toluene, exchanging the counterion between Li, Na and K (Scheme 3). We also tried to explore the cyclization in xylene, but we found clearly that the chemoselectivity using it decreased significantly.16
It’s important to say that this reaction is only able to proceed when heating is applied to the system. Therefore, in all cases the reflux temperature of the chosen solvent was applied.
The first result that surprised us is that no evidence of the 6cis product was detected when chromatographic isolation-purification of compounds were made. Examination of 1H-NMR of the crude product could not provide enough evidence for the existence of this compound. On the other hand, we desisted on the proposal about the existence of the 5cis product on the reaction mixtures, because the isolated candidates showed inconsistency on 13C-NMR, IR and MS data. Identity of the cyclobutane or cyclopentane products was achieved through 1H-NMR (signals at δ 3.16-3.09 for the methine proton that holds the dimethylcarbinol moiety and δ 4.37-4-23 for the carbinol group). 6atrans and 6ftrans compounds were also oxidized to their corresponding cyclopentanones. 13C-NMR examination of the spectra is also useful to assign the C from the carbinol group (secondary cyclic alcohol) in the 6a-ftrans frameworks between δ 80.09 and 79.19, and for the C from the dimethylcarbinol moiety (tertiary acyclic alcohol) in the 5a-ftrans frameworks between δ 71.60-71-46. COSY and NOESY experiments of selected examples also supported the identity of the compounds (Scheme 4).
It is important to say that the criteria for the quantification of the cyclobutanes 5a-f and cyclopentanes 6a-f, were based on the signals shown by the high-field methyl group (π shielded) on the cyclopentane moiety for the case of 6a-f (δ 0.58-0.67) and for the compounds 5a-f the signals shown by the high field methyl group (nearer to the aryl) on the dimethylcarbinol moiety (δ 0.81-0.95). It’s remarkable that the 5ftrans product show important difference on the chemical shifts of the dimethylcarbinol moiety in both methyl and hydroxyl groups. In the other cases (5a-etrans) the high field methyl was located on δ 0.95-0.93, while in 5ftrans appeared on 0.81 ppm; the “oxygen-free” aryl frameworks showed high field hydroxyl protons on δ 0.58-0.63, and both 3 or 3,4-dioxygenated aryl frameworks shifted that signal slightly to lower field (δ 0.76-0.71). This can be understood by terms of more intense hydrogen-bonds caused by these oxygenated moieties that are higher when are located in the positions 2 and 5 (closer to the dimethyl carbinol moiety in the trans product) relative to the aryl. Yields are considerably good in all cases. In terms of the regioselectivity, we found that reaction temperature had the most important effect. In all cases, excepting entries 7, 13, and 25 cyclobutanes 5a-f were favored when the reaction was carried out in refluxing benzene over cyclopentanes 6a-f. These exceptions feature HMDSLi as the base used, and it can be seen that cyclopentanes were favored not only when the reaction was effected in benzene but in toluene also (with an increase on the regiopreference over cyclopentanes). In most of these cases, the use of HMDSNa led to a more cyclobutane enriched mix of carbocycles with a more defined regioselectivity when benzene is the solvent. On the other hand, the use of HMDSK showed no clear tendency. When the reaction was carried out at higher temperatures (110 ºC, refluxing toluene), it’s clear that the regioselectivity favors cyclopentanes in the reactions where the HMDSLi or HMDSK bases were used.17 In the case of HMDSNa, we found that preference over cyclobutanes is lowered, excepting 4e which reverses it and 4f that increase it, but is still present in most cases.
Lallemand-Onanga’s model in some way agrees with our results, but the regio and stereochemical divergence when we switch the counterion or temperature, prompt us to propose complementary electronical and thermal factors (Scheme 5).
According to our model, both temperature and a more oxophilic Lewis Acid promoted the B transition state.18 This is sustained by our experimental results because the 5-endo pathway is promoted by HMDSLi and HMDSK, and also with higher reaction temperatures.
Complementary to this it can be seen that the high stereoselectivity trans, when the reaction goes either 4-exo (A) or 5-endo pathway (B), is caused by the steric hindrance of the cyano group. This is known to exist as an N-metalated species19 when hydrocarbon solvents are used. The contrast with the representation of nitrile anions or “keteniminates” is because are commonly represented as individual coordinated structures. However, several spectroscopic analyses show that the metallic centers are commonly accompanied with solvent or counterion ligands.9 In fact, Stork’s appreciation is not all erroneous when he assumed that the steric hindrance of the “cyano anion” was larger than a conventional alkyl group in the case of the trisubstituted epoxide 2,6,6-trimethyl-5-epoxynitrile3a; unfortunately the “keteniminate” structure was claimed as the responsible of this regioselectivity instead of the whole steric bulk of ligands coordinated with the metal center. The apparent loss of stereoselectivity when the reaction is carried out in refluxing toluene and goes through a 4-exo pathway is understandable because of the increased mobility of the system promoted by a raising on the kinetic energy of the system.
The result of the exhaustive exploration to find conditions that could lead the reaction preferably toward an endo pathway led us to find a couple of methodologies that give cyclopentanes in moderately good yields. This is based on the use of amides or tertiary alkyl amines as solvents. Reactions carried out with NaH20 in DMF or N-methylmorpholine (Table 2) showed exceptional preference for the 5-endo pathway and gave moderately good yields.15 TMEDA, triethylamine, N,N-dimethylaniline and DIPEA showed poor yields, low regioselectivity or very slow rate of cyclization.
In conclusion, we found that both the metal that companies hexamethyldisilylamide anion and the temperature have an important effect on the regioselectivity of the reaction. It seems that lithium and potassium promote a more advanced transition state who slightly leads to a 5-endo process. This tendency is achieved with an increase of the reaction temperature too. Independently of the cyclization pathway, the preferred diasteroselectivity is that which leads to the trans product. Also, we could achieve high regio and stereo selectivity using DMF or N-methylmorpholine as solvents. We are still studying the methodologies presented and the potential use of them for racemic and enantioselective syntheses of natural products.
EXPERIMENTAL
Column chromatography was performed with vacuum on Merck® 60 G silica gel. For thin layer chromatography Merck® TLC silica gel 60 F254 aluminum plates were used. Tetrahydrofuran (THF), ethyl ether (Et2O), benzene, toluene and methanol (MeOH) were used freshly distilled from metallic sodium. N,N-Dimethylformamide (DMF) and N-methylmorpholine were used freshly distilled from P2O5 and calcium hydride respectively. HMDSK, HMDSLi and HMDSNa were used as standard commercial solutions in toluene from Sigma-Aldrich®. Other chemicals were obtained from commercial sources and had analytical or synthetic grade and were used as received. 1H and 13C NMR data was obtained using Varian Unity INOVA 300 (for 300MHz 1H-NMR and 75MHz 13C-NMR data). IR spectra were obtained using a Perkin-Elmer FT-IR RXI spectrometer. Low and high resolution EIME spectra were measured using a Thermo DFS spectrometer. Microanalyses (Elemental analyses) were carried out on a Fisons EA 1108 elemental analyzer.
2-(3-Methoxy-4-methylphenyl)acetonitrile (1d). To a stirred and cold (0 °C) suspension of LiAlH4 (2.3 g, 61.1 mmol) in 50 mL of dry THF is added slowly a previously made solution of commercially available methyl 3-methoxy-4-methyl benzoate (10g, 55.6 mmol) in 100 mL of dry THF. The solution is stirred for 2 h (monitored by TLC) and then, 100 mL of cold water is added. The reaction mixture is vacuum filtered to eliminate mostly of lithium and aluminum insoluble salts, which are washed with 20 mL of EtOAc. The aqueous mixture is then satured with NH4Cl and extracted with EtOAc. The solvent from the organic phases is removed by distillation and to the solvent-free residue is added slowly with stirring at 0 °C, a solution of 11 g of CaCl2 in 60 mL of conc. HCl. Stirring is maintained for 3 h and the reaction mixture is diluted with 50 mL of cold water. The aqueous phase is then extracted with CH2Cl2 and the organic extracts are dried with CaCl2. Next, solvent is removed at 0°C in vacuo and to the residue is added NaCN (5.45, 111.2 mmol) and 80 mL of DMSO. The mixture is immersed with agitation on a preheated oil bath (80-100°C) for 1.5 h. The reaction mixture is then poured on 100 mL of cold water and extracted with EtOAc. The solvent is removed by distillation and the residue is distillated with a short path integral distillation apparatus (80-85 °C, 0.1 mmHg) to yield 8.1 g of 1d as a pure oil (90%). IR (film): νmax/cm-1 3004, 2939, 2838, 2250 (CN), 1614, 1589, 1511, 1465, 1417, 1256, 1154, 1135, 1040,
809. EIMS m/z: 161 (100%, M+), 146 (55), 130 (10), 121 (33), 116 (10), 91 (35), 77 (8). HRMS: m/z Calculated for C10H11NO 161.0841. Found 161.0840. Anal. Calcd for C10H11NO: C, 74.51%; H, 6.88%; N, 8.69. Found: C, 74.24%; H 7.07%; N, 8.66%. 1H-NMR (300 MHz, CDCl3) δ ppm 7.11 (d, J = 7.6 Hz, 1H), 6.8 (d, J = 7.6 Hz, 1H), 6.77 (s, 1H), 3.84, (s, 3H), 3.72 (s, 2H), 2.20 (s, 3H). 13C-NMR (75 MHz, CDCl3) δ ppm 158.10, 130.98, 128.34, 126.54, 119.62, 118.05, 109.38, 55.29, 23.48, 15.86.
2-(2,5-Dimethoxy-4-methylphenyl)acetonitrile (1f). To a cold stirred solution of commercial 84% methylhydroquinone (15g, 121 mmol) and 95 mL of EtOH, is added a solution of KOH (17 g, 302 mmol) in water (30 mL). Dimethyl sulphate (37.2 g, 290 mmol) is then added dropwise through a controlled-pressure funnel on 25 or 30 min interval. Next, the mixture is alkalinized by a solution of KOH (5 g) on water (6 mL), and heating is applied with stirring until boiling is reached. This is maintained for 4 h and finally EtOH is removed in vacuo. A solution of NaOH (23.6 g, 0.59 mmol) in water (100 mL) is added to the reaction mixture and stirred for 12 h. The organic layer is then collected and the aqueous is extracted with Et2O. The organic phases are put together, washed with brine and CaCl2 dried. The solvent is removed by distillation and the residue is vacuum distilled (98-100 °C, 1 mmHg) obtaining 13.4 g of 1,4-dimethoxy-2-methylbenzene as a pure oil (96% yield). IR (film): νmax/cm-1 2996, 2948, 2833, 1504, 1466, 1281, 1224, 1050, 1032, 798, 712. EIMS m/z: 152 (52%, M+), 137 (100), 109 (19), 95 (11), 77 (16). Anal. Calcd for C9H12O2: C, 71.03%; H, 7.95%. Found: C, 71.29%; H 7.95%. 1H-NMR (300 MHz, CDCl3) δ ppm 6.77-6.65 (m, 3H), 3.78 (s, 3H), 3.75 (s, 3H), 2.21 (s, 3H). 13C-NMR (75 MHz, CDCl3) δ ppm 153.32, 151.99, 127.74, 116.99, 110.83, 110.64, 55.79, 55.55, 16.32. To a cold Vilsmeier reagent, prepared with POCl3 (40.35 g, 253.2 mmol) and DMF (39 g, 526.3 mmol) on an ice bath, is added slowly 1,4-dimethoxy-2-methylbenzene (10 g, 65.8 mmol). The red mixture is heated with a steam bath at 60-70 °C for 16 h. After this, the reaction mixture is poured slowly on water-ice (100 mL) and added with half saturated aqueous NaOH solution until pH=7. The resultant solid is quickly vacuum filtered and then diluted with hot MeOH (75 mL). Heating is applied and maintained until approximately one third of MeOH is evaporated. The solution is stored at -15°C overnight and the resultant solid is vacuum filtered and washed with cold MeOH. The white solid is finally vacuum dried to give 11.25 g of 2,5-dimethoxy-4-methylbenzaldehyde as pure white crystals (95% yield). mp 81-81.5 ºC. IR (KBr): νmax/cm-1 3010, 2919, 2832, 2780, 1661, 1613, 1501, 1407, 1213, 1046. EIMS m/z: 180 (100%, M+), 165 (48), 137 (30), 109 (16), 77 (10). Anal. Calcd for C10H12O3: C, 66.65%; H, 6.71%. Found: C, 66.34%; H, 6.59%. 1H-NMR (300 MHz, CDCl3) δ ppm 10.40 (1H, s), 7.25 (1H, s), 6.81 (1H, s), 3.89 (3H, s), 3.83 (3H, s), 2.28 (3H, s). 13C-NMR (76 MHz, CDCl3) δ ppm 189.09, 156.54, 151.93, 136.47, 122.83, 114.65, 107.61, 56.08, 55.67, 17.16. To a cold stirred suspension of 2,5-dimethoxy-4-methylbenzaldehyde (8 g, 44.4 mmol) in absolute MeOH, is added in small portions NaBH4 (1.2 g, 31.6 mmol) and stirred for 1 h. Next, the reaction mixture is poured on water-ice (20 mL) and added aqueous H3PO4 10% until pH=6. Once crystals appear, these are collected by vacuum filtration. The filtrate is concentrated by distillation to half of initial volume and new crystals are formed. These are collected by vacuum filtration too and both crops are washed with cold water and dried to air. (2,5-Dimethoxy-4-methylphenyl)methanol is obtained as white crystals that have enough purity to be used in the next reaction (9.9 g, 98% yield). mp 76-76.5 ºC. IR (KBr): νmax/cm-1 3288, 3002, 2951, 2933, 1511, 1466, 1402, 1213, 1048, 868. EIMS m/z: 182 (100%, M+), 165 (18), 149 (18), 139 (34), 124 (30), 107 (16), 91 (22), 77 (34). Anal. calcd. for C10H14O3: C, 65.91%; H, 7.74%. Found: 66.10%; H, 7.75%. 1H-NMR (300 MHz, CDCl3) δ ppm 6.79 (s, 1H), 6.70 (s, 1H), 4.64 (s, 1H), 3.80 (s, 1H), 3.78 (s, 1H), 2.45 (s, 1H, D2O), 2.22 (s, 1H). 13C-NMR (76 MHz, CDCl3) δ ppm 151.57, 150.95, 126.82, 126.57, 113.60, 111.52, 61.83, 56.01, 55.83, 16.21. To cold pulverized (2,5-dimethoxy-4-methylphenyl)methanol (5 g, 27.5 mmol) is added slowly with stirring conc. HCl (50 mL). After 10 min of stirring the mixture is poured on 60 mL of water-ice and crystallization is induced for not more than a minute. The solid is then vacuum filtered and air dried for 2-3 min. The solid is then added in very small portions to a preheated (50 °C) KCN/DMF suspension, which had been prepared with KCN (7.2 g, 110.8 mmol) and anhydrous DMF (54 mL). The reaction is stirred at 50-60 °C for 30 min. The orange reaction mix is poured on 100 mL of water-ice and is maintained at 0 °C until all the product crystallizes. The solid is then vacuum filtered and distilled with a Kugel-Rohr apparatus (155-160 °C, 5 mmHg). 2-(2,5-Dimethoxy-4-methylphenyl)acetonitrile (1f) crystallizes upon standing to give 4.41 g of a white solid (84% yield). mp 65.5-66 ºC. IR (KBr): νmax/cm-1 3049, 2931, 2835, 2250 (CN), 1514, 1464, 1403, 1416, 1219, 1043, 866. EIMS: m/z 182 (50%, M+), 176 (100), 161 (4), 148 (5), 133 (5). HRMS m/z Calcd for C11H13NO2 (M+): 191.0946. Found: 191.0936. Anal. Calcd for C11H13NO2: C, 69.09%; H, 6.85%; N, 7.32%. Found: 69.14%; H, 6.88%; N, 7.30%. 1H-NMR (300 MHz, CDCl3) δ ppm: 6.83 (s, 1H), 6.71 (s, 1H), 3.81 (s, 3H), 3.81 (s, 3H), 3.67 (s, 2H), 2.22 (s, 3H). 13C-NMR (76 MHz, CDCl3) δ ppm: 151.68, 150.34, 127.49, 118.23, 115.84, 113.73, 111.59, 56.04, 55.94, 18.37, 16.23.
5-Iodo-2-methylpent-2-ene (2) was prepared as proposed by Biernacki and Gdula (Reference 14). To a stirred and cold ethereal solution of methylmagnesium iodide, which had been prepared with methyl iodide (6.2 mL, 14.19 g, 100 mmol) and magnesium turnings (2.43 g, 100 mg-atom) in anhydrous Et2O (40 mL), was added dropwise a solution of commercial cyclopropyl methyl ketone (8.2 g, 97 mmol) in anhydrous Et2O (10 mL). The resultant slurry is slowly added to cold H2SO4 (50%) being careful that the temperature does not reach 10 °C. Next, the resulting mixture is stirred for 30 min. Phases are then separated and the aqueous is extracted with ethyl ether. Organic extracts are then washed twice with aqueous NaHSO3 (5%, 10 mL each), aqueous NaHCO3 (10%, 10 mL each) and brine (10 mL). Organic extract is then dried with Na2SO4 and concentrated in vacuo. Residue is distilled with a short path integral apparatus (65-75 °C, 15 mmHg) to give 16.8 g of product 2 as pure clear oil (82% yield). IR (film): νmax/cm-1 2969, 2927, 1670 (C=C), 1447, 1377, 1248, 1210, 1165, 1090, 832, 599, 520, 453. EIMS: m/z 210 (10%, M+), 127 (6), 95 (6), 83 (100), 67 (12), 55 (45), 41 (25). 1H-NMR (300 MHz, CDCl3) δ ppm 5.10 (mt, J = 7.2, 1H), 3.11 (t, J = 7.4 Hz, 2H), 2.57 (q, J = 7.4 Hz, 2H), 1.70 (s, 3H), 1.62 (s, 3H). 13C-NMR (75.5 MHz, CDCl3) δ ppm 134.49, 123.05, 32.50, 25.67, 17.93, 6.05.
General method for the syntheses of the 6-methyl-2-arylhept-5-enenitriles 3a-f: To a stirred solution of lithium 1,1,1,3,3,3-hexamethyldisilylamide, which had been prepared with by treating a solution of 1,1,1,3,3,3-hexamethyldisilazane (4.4 mL, 3.4 g, 21 mmol) with a 1.6 M n-BuLi solution in hexanes (7.9 mL, 12.6 mmol) in THF (100 mL) at -70 ºC, was added dropwise a solution of the 2-arylacetonitrile 1a-f (10.5 mmol) in THF (10 mL) through a syringe. The solution is stirred for 30 min and then, a solution of the 5-iodo-2-methylpent-2-ene (2) (2.31 g, 11 mmol) in THF (10 mL) is added in one portion through syringe. The mixture is maintained with stirring at low temperature for the next 2-3 h (monitored by TLC). After this, the resulting slurry is poured into a flask containing 50 mL of ice-water. The aqueous phase is satured with NH4Cl, and the phases are separated. The aqueous phase is extracted with EtOAc. The solvent from the organic phases is removed in vacuo while the crude is purified by flash column chromatography on silica gel using mixtures of hexane and EtOAc or acetone as eluants to give the pure monoalkylated product 3a-f. Yields: 77 – 95%.
6-Methyl-2-phenylhept-5-enenitrile (3a) IR (film): νmax/cm-1 3064, 3032, 2968, 2928, 2860, 2240 (CN), 1958, 1879, 1812, 1601, 1496, 1453, 1378, 1108, 1030, 831, 757, 699. EIMS m/z: 199 (30%, M+), 184 (14), 171 (18), 143 (6), 129 (30), 117 (38), 103 (14), 89(14), 83 (20), 69 (36), 55(100). HRMS: m/z Calculated for C14H17N 199.1361. Found 199.1353. Anal. Calcd for C14H17N: C, 84.37%; H, 8.60%; N, 7.03%. Found: 84.39%; H, 8.64%; N, 6.98%. 1H-NMR (300 MHz, CDCl3) δ ppm 7.41-7.27 (m, 5H, Ar), 5.07 (mt, J = 7.16 Hz, 1H), 3.77 (dd, J = 8.7, 6.2 Hz, 1H), 2.27-2.07 (m, 2H), 1.99 (dddd, J=5.7, 8.2, 8.7, 13.7 Hz, 1H), 1.86 (dtd, J=13.7, 7.8, 6.2 Hz, 1H), 1.71 (s, 3H), 1.60 (s, 3H). 13C-NMR (75 MHz, CDCl3) δ ppm 135.94, 133.95, 128.96 (2C), 127.91, 127.22 (2C), 121.83, 120.84, 36.56, 35.88, 25.65, 25.39, 17.75.
6-Methyl-2-p-tolylhept-5-enenitrile (3b) IR (film): νmax/cm-1 3052, 3026, 2968, 2926, 2860, 2240 (CN), 1902, 1797, 1673, 1514, 1450, 1377, 1111, 813, 511. EIMS m/z: 213 (100%, M+), 198 (17), 185 (13), 172 (11), 157 (8), 143 (70), 131 (32), 117 (12), 83 (12), 69 (7), 55 (17). HRMS: m/z Calculated for C15H19N: 213.1517. Found 213.1520. Anal. Calcd for C15H19N: C, 84.46%; H, 8.98%; N, 6.57%. Found: 84.33%; H, 8.97%; N, 6.53%. 1H-NMR (300 MHz, CDCl3) δ ppm 7.23-7.14 (m, 4H, Ar), 5.07 (mt, J = 7.15 Hz, 1H), 3.74 (dd, J = 8.7, 6.3 Hz, 1H), 2.34 (s, 3H), 2.26-2.06 (m, 2H), 1.97 (dddd, J = 13.7, 9.2, 8.7, 8.2, 5.8 Hz, 1H), 1.84 (dtd, J = 13.7, 7.8, 6.3 Hz, 1H), 1.70 (s, 3H), 1.60 (s, 3H). 13C-NMR (75 MHz, CDCl3) δ ppm 137.71, 133.90, 132.94, 129.62 (2C), 127.12 (2C), 121.93, 121.05, 36.20, 35.91, 25.68, 25.41, 20.99, 17.78.
2-(3,4-Dimethoxyphenyl)-6-methylhept-5-enenitrile (3c) IR (film): νmax/cm-1 2962, 2935, 2838, 2239 (CN), 1594, 1518, 1464, 1421, 1379, 1262, 1241, 1145, 1027, 854, 811, 764. EIMS m/z: 259 (100%, M+), 244 (22), 218 (10), 189 (72), 177 (62), 162 (20), 146 (16). HRMS: m/z Calculated for C16H21NO2 259.1572. Found 259.1571. Anal. Calcd for C16H21NO2: C, 74.10%; H, 8.16%; N, 5.40%. Found: 74.10%; H, 8.10%; N, 5.34%. 1H-NMR (300 MHz, CDCl3) δ ppm 6.86-6.84 (m, 2H), 6.83-6.81 (bs, 1H), 5.08 (mt, J = 7.2 Hz, 1H), 3.90 (s, 3H), 3.88 (s, 3H), 3.73 (dd, J = 8.6, 6.4 Hz, 1H), 2.25-2.09 (m, 2H), 2.03-1.79 (m, 2H), 1.98 (dddd, J = 13.6, 8.6, 8.1, 6.0 Hz, 1H), 1.85 (dtd, J = 13.6, 7.8, 6.4 Hz, 1H), 1.71 (s, 3H), 1.61 (s, 1H). 13C-NMR (75 MHz, CDCl3) δ ppm 149.29, 148.72, 133.80, 128.28, 121.83, 121.00, 119.54, 111.39, 110.29, 55.88, 55.84, 36.09, 35.79, 25.59, 25.34, 17.71.
2-(3-Methoxy-4-methylphenyl)-6-methylhept-5-enenitrile (3d) IR (film): νmax/cm-1 2927, 2860, 2239 (CN), 1612, 1588, 1511, 1465, 1415, 1378, 1254, 1136, 1040, 850, 814, 642. EIMS m/z: 243 (85%, M+), 228 (15), 173 (60), 161 (100), 146 (30), 121 (10), 83 (10), 55 (15). HRMS: m/z Calculated for C16H21NO 243.1623. Found 243.1634. Anal. Calcd for C16H21NO: C, 78.97%; H, 8.70%; N, 5.76%. Found: 79.05%; H, 8.68%; N, 5.70%. 1H-NMR (300 MHz, CDCl3) δ ppm 7.10 (d, J = 7.5 Hz, 1H), 6.80-6.76 (m, 2H), 5.08 (mt, J = 6.9 Hz, 1H), 3.84 (s, 3H), 3.73 (dd, J = 8.9, 6.3 Hz, 1H), 2.22-2.13 (m, 2H), 2.20 (s, 3H), 1.99 (dddd, J = 13.4, 8.9, 8.1, 6.0 Hz, 1H), 1.86 (dtd, J = 13.4, 7.9, 6.3 Hz, 1H) , 1.71 (s, 3H), 1.62 (s, 3H). 13C-NMR (75 MHz, CDCl3) δ ppm 158.06, 134.58, 133.91, 130.89, 126.50, 121.93, 121.08, 119.06, 108.74, 55.31, 36.56, 35.92, 25.68, 25.46, 17.81, 15.86.
2-(3-Methoxyphenyl)-6-methylhept-5-enenitrile (3e) IR (film): νmax/cm-1 2965, 2931, 2861, 2838, 2240 (CN), 1602, 1588, 1491, 1455, 1438, 1378, 1324, 1264, 1164, 1043, 783, 697. EIMS m/z: 229 (10%, M+), 159 (12), 147 (100), 161 (10), 77 (8), 55 (16), 41 (20). HRMS: m/z Calculated for C15H19NO 229.1467. Found 229.1456. Anal. Calcd for C15H19NO: C, 78.56%; H, 8.35%; N, 6.11%. Found: 78.64%; H, 8.33%; N, 6.05%.1H-NMR (300 MHz, CDCl3) δ ppm 7.31-7.25 (m, 1H), 6.92-6.82 (m, 3H), 5.07 (mt, J = 7.2 Hz, 1H), 3.81 (s, 3H), 3.75 (dd, J = 8.7, 6.3 Hz, 1H), 2.25-2.10 (m, 2H), 1.99 (dddd, J = 13.7, 8.7, 8.1, 5.8 Hz, 1H), 1.86 (dtd, J = 13.7, 7.9, 6.3 Hz, 1H), 1.71 (s, 3H), 1.61 (s, 3H). 13C-NMR (75 MHz, CDCl3) δ ppm 159.97, 137.36, 134.04, 130.02, 121.81, 120.83, 119.53, 113.27, 113.06, 55.26, 36.54, 35.79, 25.71, 25.40, 17.81.
2-(2,5-Dimethoxy-4-methylphenyl)-6-methylhept-5-enenitrile (3f) IR (film): νmax/cm-1 2932, 2854, 2240 (CN), 1513, 1466, 1400, 1214, 1045, 862, 682. EIMS m/z: 273 (100%, M+), 258 (10), 203 (36), 191 (40), 190 (32), 176 (30), 152 (16). HRMS m/z Calculated for C17H23NO2: 273.1729. Found: 273.1728. Anal. Calcd for C17H23NO2: C, 74.69%; H, 8.48%; N, 5.12%. Found: 74.67%; H, 8.52%; N, 5.13%. 1H-NMR (300 MHz, CDCl3) δ ppm 6.85 (s, 1H), 6.70 (s, 1H), 5.12 (mt, J =7.1 Hz, 1H), 4.15 (dd, J = 8.8, 5.9 Hz, 1H), 3.81 (s, 3H), 3.79 (s, 3H), 2.22 (s, 3H), 2.18 (m, 2H), 1.97-1.76 (m, 2H), 1.70 (s, 3H), 1.62 (s, 3H). 13C-NMR (75 MHz, CDCl3) δ ppm 151.75, 149.69, 133.37, 127.24, 122.25, 121.80, 121.37, 114.06, 110.47, 55.99 (2C), 34.01, 30.81, 25.69, 25.63, 17.70, 16.19.
Syntheses of the 4-(3,3-dimethyloxiran-2-yl)-2-arylbutanenitriles: Method A (This method is suitable for the preparation of the epoxides 3c and 3f.): To a stirred cold solution (0 ºC) of the 2-aryl-6-methylhept-5-enonitrile (5.9 mmol) in 16 mL of CH2Cl2, was added in three portions (intervals of 15 min) commercial 77% m-chloroperoxybenzoic acid (1.6 g, 7.1 mmol). The resulting mixture was stirred for 3 h at 0 ºC. The reaction mixture was then vacuum filtered and the solid was washed with 45 mL of cold CH2Cl2. The filtrate was added then 15 mL of 5% aqueous sodium sulfite and 15 mL of saturated aqueous sodium bicarbonate. The flask is then pulled out from the bath and is stirred at ambient temperature for 15 min. The organic phase is collected and the aqueous is extracted with CH2Cl2. After this, the organic extracts are put together, dried with Na2SO4 and concentrated in vacuo at ambient temperature. The residue was chromatographed over silica gel using hexane-EtOAc mixtures as eluants. Yields: 85-96%. Method B (This method is suitable for the preparation of the epoxides 3a, 3b, 3d and 3e.): To a stirred cold solution of the 2-aryl-6-methylhept-5-enonitrile (5.9 mmol) and 2 mL of water, in 20 mL of acetone, was added in one portion Oxone® (7.25g, 11.8 mmol). After this, NaHCO3 is added in very small portions taking care of maintain the temperature below 10 ºC. Gas development occurs and the mixture is stirred for 30 min. Then the reaction is diluted with 50 mL of brine and extracted with EtOAc. The organic extracts are put together, dried with Na2SO4, and concentrated in vacuo at ambient temperature. The product is purified over silica gel using hexane-acetone mixtures as eluants to give the pure products. Yields: 95%-100%.
Diastereoisomeric mixture of the 4-(3,3-dimethyloxiran-2-yl)-2-phenylbutanenitrile (4a) IR (film): νmax/cm-1 3064, 3032, 2964, 2928, 2868, 2240 (CN), 1959, 1884, 1601, 1496, 1455, 1379, 1326, 1251, 1123, 900, 758, 700. EIMS m/z: 215 (5%, M+), 200, (5), 172 (22), 156 (15), 145 (10), 130 (58), 128 (80), 117 (45), 116 (72), 104 (100), 91 (32), 86 (28), 77 (12), 71 (16), 59 (19). HRMS m/z Calculated for C14H17NO: 215.1310. Found: 215.1309. Anal. Calcd for C14H17NO: C, 78.10%; H, 7.96%; N, 6.51%. Found: 78.11%; H, 7.99%; N, 6.50%. 1H-NMR (300 MHz, CDCl3) δ ppm 7.43-7.32 (m, 10H), 3.94-3.87 (m, 2H), 2.74, (dd, J = 5.4, 4.9 Hz, 1H), 2.72 (dd, J = 5.7, 4.8 Hz, 1H), 2.25-1.95 (m, 4H), 1.91-1.50 (m, 4H), 1.30 (s, 6H), 1.28 (s, 3H), 1.22 (s, 3H). 13C-NMR (75 MHz, CDCl3) δ ppm 135.61, 135.30, 129.09 (2C), 129.06 (2C), 128.15, 128.12, 127.34 (2C), 127.11 (2C), 120.51, 120.39, 63.26, 62.93, 58.31, 58.20, 37.25, 36.65, 33.19, 32.73, 26.53, 25.83, 24.64 (2C), 18.67, 18.65.
Diastereoisomeric mixture of the 4-(3,3-dimethyloxiran-2-yl)-2-p-tolylbutanenitrile (4b) IR (film): νmax/cm-1 3026, 2964, 2927, 2868, 2240 (CN), 1906, 1802, 1514, 1455, 1380, 1326, 1250, 1121, 900, 874, 816, 678, 530, 514. EIMS m/z: 229 (18%, M+), 186 (14), 159 (10), 156 (14), 143 (100), 130 (48), 118 (95), 103 (19), 91 (16), 86 (39), 77 (12), 71 (16). HRMS m/z Calculated for C15H19NO: 229.1467. Found: 229.1464. Anal. Calcd for C15H19NO: C, 78.56%; H, 8.35%; N, 6.11%. Found: 78.57%; H, 8.38%; N, 6.10%. 1H-NMR (300 MHz, CDCl3) δ ppm 7.27-7.15 (m, 8H), 3.90-3.82 (m, 2H), 2.73 (t, J = 4.9 Hz, 1H), 2.71 (t, J = 5.0 Hz, 1H), 2.34 (s, 6H), 2.22-1.92 (m, 4H), 1.89-1.50 (m, 4H), 1.30 (s, 6H), 1.27 (s, 3H), 1.22 (s, 1H). 13C-NMR (75 MHz, CDCl3) δ ppm 137.89, 137.87, 132.55, 132.24, 129.67 (2C), 129.65 (2C), 127.16 (2C), 126.93 (2C), 120.65, 120.54, 63.24, 62.93, 58.23, 58.13, 36.79, 36.22, 33.12, 32.68, 26.46, 25.81, 24.61, 24.60, 20.93 (2C), 18.62 (2C).
Diastereoisomeric mixture of the 2-(3,4-dimethoxyphenyl)-4-(3,3-dimethyloxiran-2-yl)butanenitrile (4c) IR (KBr): νmax/cm-1 2934, 2860, 2838, 2239 (CN), 1594, 1518, 1465, 1421, 1378, 1342, 1262, 1240, 1145, 1028, 850, 809, 764, 641. mp 50-52 ºC (diasteroisomeric mixture) EIMS m/z: 275 (12%, M+), 189 (100), 176 (62), 164 (15), 146 (10), 119 (11), 91 (5), 77(5). HRMS m/z Calculated for C16H21NO3: 275.1521. Found: 275.1523. Anal. Calcd for C16H21NO3: C, 69.79%; H, 7.69%; N, 5.09%. Found: 69.81%; H, 7.66%; N, 5.14%. 1H-NMR (300 MHz, CDCl3) δ ppm 6.92-6.82 (m, 3H), 3.90 (s, 6H), 3.88 (s, 6H), 3.86 (m, 2H), 2.76 (t, J = 4.7 Hz, 1H), 2.73 (t, J = 4.7 Hz, 1H), 2.24-1.49 (m, 8H), 1.32 (s, 6H), 1.29 (s, 3H), 1.24 (s, 3H). 13C-NMR (75 MHz, CDCl3) δ ppm 149.41, 149.36, 148.89 (2C), 127.99, 127.64, 120.74, 120.60, 119.72, 119.43, 111.49, 111.46, 110.45, 110.19, 63.31, 63.01, 58.27, 58.22, 55.95 (2C), 55.91 (2C), 36.83, 36.14, 33.22, 32.81, 26.52, 25.74, 24.63, 24.62, 18.67 (2C).
Diastereoisomeric mixture of the 4-(3,3-dimethyloxiran-2-yl)-2-(3-methoxy-4-methylphenyl)- butanenitrile (4d) IR (Film): νmax/cm-1 2962, 2928, 2868, 2839, 2240 (CN), 1612, 1588, 1511, 1465, 1416, 1379, 1254, 1154, 1136, 1040, 853, 817, 676, 642. EIMS m/z: 259 (4%, M+), 243 (4), 186 (5), 173 (100), 161 (27), 148 (14), 121 (4), 103 (5), 91 (5), 77 (5). HRMS m/z Calculated for C16H21NO2: 259.1572. Found: 259.1563. Anal. Calcd for C16H21NO2: C, 74.10%; H, 8.16%; N, 5.40%. Found: 74.14%; H, 8.14%; N, 5.31%. 1H-NMR (300 MHz, CDCl3) δ ppm 7.12 (d, J = 7.5 Hz, 2H), 6.82 (dd, J = 7. 6, 1.8 Hz, 2H), 6.78 (d, J = 1.8 Hz, 1H), 6.8 (d, J = 1.78 Hz, 1H), 3.90-3.84 (m, 2H), 3.85 (s, 3H), 3.84 (s, 3H), 2.75 (t, J = 4.8 Hz, 1H), 2.73 (t, J = 4.8 Hz, 1H), 2.20 (s, 6H), 2.18-1.94 (m, 4H), 1.93-1.51 (m, 4H), 1.31 (s, 6H), 1.29 (s, 3H), 1.24 (s, 3H). 13C-NMR (75 MHz, CDCl3) δ ppm 158.14, 158.11, 134.24, 133.92, 131.01, 130.98, 126.74 (2C), 120.74, 120.61, 119.14, 118.88, 108.91, 108.65, 63.36, 63.04, 58.30, 58.23, 55.35 (2C), 37.25, 36.60, 33.26, 32.82, 26.60, 25.87, 24.67, 24.66, 18.71 (2C), 15.85 (2C).
Diastereoisomeric mixture of the 4-(3,3-dimethyloxiran-2-yl)-2-(3-methoxyphenyl)butanenitrile (4e) IR (KBr): νmax/cm-1 2963, 2930, 2839, 2240 (CN), 1603, 1588, 1490, 1456, 1438, 1380, 1325, 1264, 1152, 1124, 1047, 871, 785, 698. EIMS m/z: 245 (6%, M+), 202 (5), 159 (100), 147 (29), 134 (38), 121 (9), 116 (11), 103 (9), 91 (10), 77 (9), 43 (15). HRMS m/z Calculated for C15H19NO2: 245.1416. Found: 245.1406. Anal. Calcd for C15H19NO2: C, 73.44%; H, 7.81%; N, 5.71%. Found: 73.29%; H, 7.79%; N, 5.65%. 1H-NMR (300 MHz, CDCl3) δ ppm 7.33-7.26 (m, 2H), 6.96-6.83 (m, 6H), 3.92-3.84 (m, 2H), 3.82 (s, 6H), 2.75 (t, J = 4.8 Hz, 1H), 2.72 (t, J = 4.9 Hz, 1H), 2.17 (s, 3H), 2.24-1.95 (m, 4H), 1.92-1.51 (m, 4H), 1.31 (s, 6H), 1.28 (s, 3H), 1.24 (s, 3H). 13C-NMR (75 MHz, CDCl3) δ ppm 160.05, 160.02, 137.02, 136.70, 130.15, 130.11, 120.47, 120.35, 119.58, 119.35, 113.55, 113.48, 113.13, 112.93, 63.30, 62.97, 58.34, 58.25, 55.28 (2C), 37.23, 36.63, 33.11, 32.65, 26.53, 25.84, 24.65 (2C), 18.68 (2C).
Diastereoisomeric mixture of the 2-(2,5-dimethoxy-4-methylphenyl)-4-(3,3-dimethyloxiran-2-yl)- butanenitrile (4f) IR (KBr): νmax/cm-1 2961, 2854, 2240 (CN), 1510, 1466, 1400, 1214, 1044, 863. mp: 41-42 ºC (Diasteroisomeric mixture) EIMS: m/z 289 (64%, M+), 216 (10), 203 (100), 190 (56), 188 (58), 178 (20), 163 (14), 150 (14). HRMS m/z calculated for C17H23NO3 (M+): 289.1678. Found: 289.1655. Anal. Calcd for C17H23NO3: C, 70.56%; H, 8.01%; N, 4.84%. Found: 70.40%; H, 8.00%; N, 4.80%. 1H-NMR (300 MHz, CDCl3) δ ppm 6.87 (s, 1H), 6.86 (s, 1H), 6.72 (s, 2H), 4.25 (t, J = 6.3 Hz, 1H), 4.22 (t, J = 6.1 Hz, 1H), 3.83 (s, 3H), 3.82 (s, 3H), 3.80 (s, 6H), 2.73 (m, 2H), 2.23 (s, 6H), 2.16-1.90 (m, 4H), 1.88-1.58 (m, 4H), 1.32 (s, 6H), 1.28 (s, 3H), 1.27 (s, 3H). 13C-NMR (76 MHz, CDCl3) δ ppm 151.85 (2C), 149.70 (2C), 127.64, 127.60, 121.35, 121.15, 121.06, 121.00, 114.15, 114.11, 110.62, 110.46, 63.40, 63.17, 58.37, 58.21, 56.10 (2C), 56.05 (2C), 31.40, 31.22, 31.09, 30.83, 26.78, 26.38, 24.74 (2C), 18.70 (2C), 16.29 (2C).
General Method A for the hexamethyldisilylamide base / benzene or toluene system: To a stirred solution of the corresponding 2-aryl-4-(3,3-dimethyloxiran-2-yl)butanenitrile (0.086 mmol) 4a-f in the chosen solvent (toluene or benzene, 1.3 mL) at 0ºC, was added drop wise through syringe a commercial solution of the 1,1,1,3,3,3-hexamethyldisilylamide base in toluene (0.216 mmol). The solution is maintained with stirring in the cold bath for 15 min, and then pulled out from to allow the temperature to increase; the stirring is maintained for other 15 min. After this, the reaction mixture is immersed in a pre-heated oil bath at the corresponding reflux temperature of the chosen solvent, and then refluxed for 30-45 min. After this time, the mixture is immersed in a water bath and then poured on 4 mL of ice-water. The organic phase is collected, and the aqueous is satured with NH4Cl, and extracted successively with Et2O. The crude product is analyzed immediately by 1H-NMR to obtain the proportion of the products. Yields: 70-90%.
General Method B for the sodium hydride / DMF or N-methylmorpholine system. To a stirred suspension of benzene washed sodium hydride (2 g, 5.19 mmol, in the chosen solvent (DMF or N-methylmorpholine, 2 mL) at 0 ºC, was added drop wise through a syringe a solution of the 2-aryl-4-(3,3-dimethyloxiran-2-yl)butanenitrile 4a-f (0.086 mmol) in 0.5 mL of anhydrous solvent. The solution is maintained with stirring in the cold bath for 15 min, and then pulled out from it to allow the temperature to increase; the stirring is maintained for other 5 min. After this, the reaction mixture is immersed in a pre-heated oil bath at the corresponding boiling temperature of the chosen solvent, and refluxed for 10 min (DMF) or 24 h (N-methylmorpholine). Next, the mix is immersed in a water bath and then poured on 5 mL of ice, followed by a NH4Cl saturation, and addition of citric acid until pH=3-4 is reached. The organic phase is collected, the aqueous is extracted successively with Et2O, and the organic extracts are concentrated in vacuo. The crude product is then chromatographed using mixtures of hexane-acetone collecting all products that are included in the carbocycles mix fractions. This paraffin-free product is analyzed by 1H-NMR to obtain the proportion of the products. Yields: 72-25%.
l-2-(2-Hydroxypropan-2-yl)-1-phenylcyclobutanecarbonitrile (5atrans) IR (film): νmax/cm-1 3468, 2971, 2930, 2879, 2230 (CN), 1498, 1465, 1447, 1370, 1238, 1151, 946, 761, 701. EIMS: m/z 215 (3%, M+), 200 (1), 182 (4), 172 (88), 156 (8), 143 (18), 129 (80), 115 (18), 103 (52), 86 (37), 77 (15), 71 (100). HRMS m/z Calculated for C14H17NO: 215.1310. Found: 215.1300. Anal. Calcd for C14H17NO: C, 78.10%; H, 7.96%; N, 6.51%. Found: 78.07%; H, 7.94%; N, 6.48%. 1H-NMR (300 MHz, CDCl3) δ ppm 7.70-7.65 (m, 2H), 7.48-7.34 (m, 3H), 3.12 (dd, J = 10.8, 8.8 Hz, 1H), 2.80 (ddd, 12.0, 10.8, 8.8 Hz, 1H), 2.70 (ddd, J = 12.0, 8.8, 2.6 Hz, 1H), 2.53 (ddd, J = 11.5, 10.8, 8.8 Hz, 1H), 2.18 (dtd, J = 11.5, 8.8, 2.6 Hz, 1H), 1.10 (s, 3H), 0.93 (s, 3H), 0.63 (bs, 1H, D2O). 13C-NMR (75 MHz, CDCl3) δ ppm 134.53, 128.98 (2C), 128.43, 127.92 (2C), 124.24, 71.46, 55.61, 42.07, 29.95, 27.16, 26.99, 20.17.
l-2-(2-Hydroxypropan-2-yl)-1-p-tolylcyclobutanecarbonitrile (5btrans) IR (film): νmax/cm-1 3470, 3029, 2973, 2927, 2879, 2231 (CN), 1759, 1666, 1514, 1464, 1445, 1371, 1238, 1151, 945, 814, 506. EIMS m/z: 229 (3%, M+), 211 (4), 196 (5), 186 (25), 143 (100), 131 (9), 115 (21), 91 (28), 86 (8), 77 (5), 71 (28), 59(10). HRMS m/z Calculated for C15H19NO: 229.1467. Found: 229.1466. Anal. Calcd for C15H19NO: C, 78.56%; H, 8.35%; N, 6.11%. Found: 78.37%; H, 8.33%; N, 6.05%. 1H-NMR (300 MHz, CDCl3) δ ppm 7.55 (d, J = 8.3 Hz, 2H), 7.25 (d, J = 8.3 Hz, 2H), 3.09 (dd, J = 10.8, 8.8 Hz, 1H), 2.78 (ddd, J = 11.7, 10.8, 8.8 Hz, 1H), 2.66 (ddd, J = 11.7, 8.8, 2.4 Hz, 1H), 2.52 (ddd, J = 11.4, 10.8, 8.8 Hz, 1H), 2.37 (s, 3H), 2.16 (dddd, J = 11.4, 8.9, 8.8, 2.4 Hz, 1H), 1.12 (s, 3H), 0.93 (s, 3H), 0.63 (bs, 1H, D2O). 13C-NMR (75 MHz, CDCl3) δ ppm 138.47, 131.26, 129.76 (2C), 127.75 (2C), 124.34, 71.51, 55.40, 41.66, 29.98, 27.17, 26.97, 20.95, 20.11.
l-1-(3,4-Dimethoxyphenyl)-2-(2-hydroxypropan-2-yl)cyclobutanecarbonitrile (5ctrans). IR (film): νmax/cm-1 3521, 2968, 2838, 2230 (CN), 1605, 1590, 1519, 1464, 1455, 1414, 1261, 1241, 1172, 1153, 1026, 950, 853, 810, 768. EIMS m/z: 275 (2%, M+), 257 (4), 226 (4), 214 (4), 189 (100), 176 (6), 174 (14), 146 (7), 119 (10), 103 (3), 91 (3). HRMS m/z Calculated for C16H21NO3: 275.1521. Found: 275.1523. Anal. Calcd for C16H21NO3: C, 69.79%; H, 7.69%; N, 5.09%. Found: 69.58%; H, 7.67%; N, 5.05%. 1H-NMR (300 MHz, CDCl3) δ ppm 7.20 (dd, J = 8.3, 2.3 Hz, 1H), 7.16 (d, J = 2.3 Hz, 1H), 6.92 (d, J = 8.3 Hz, 1H), 3.93 (s, 3H), 3.90 (s, 1H), 3.09 (dd, J = 10.7, 8.9 Hz, 1H), 2.80 (ddd, J = 12.0, 10.7, 8.9 Hz, 1H), 2.67 (ddd, J = 12.0, 8.8, 2.6 Hz, 1H), 2.51 (ddd, J = 11.3, 10.7, 8.9 Hz, 1H), 2.18 (dddd, J = 11.3, 8.9, 8.8, 2.6 Hz, 1H), 1.14 (s, 3H), 0.95 (s, 3H), 0.76 (bs, 1H, D2O). 13C-NMR (75 MHz, CDCl3) δ ppm 149.23 (2C), 126.52, 124.40, 120.10, 111.57, 111.34, 71.50, 56.07, 55.91, 55.49, 41.75, 30.13, 27.29, 26.96, 20.19.
l-2-(2-Hydroxypropan-2-yl)-1-(3-methoxy-4-methylphenyl)cyclobutanecarbonitrile (5dtrans). IR (film): νmax/cm-1 3469, 2966, 2927, 2836, 2230 (CN), 1612, 1581, 1510, 1465, 1408, 1379, 1253, 1173, 1143, 1037, 947, 820. EIMS m/z: 159 (2%, M+), 241 (5), 226 (4), 216 (8), 198 (5), 187 (4), 173 (100), 158 (22), 121 (6), 115 (10), 103 (12), 91 (5), 77 (8), 71 (7). HRMS m/z Calculated for C16H21NO2: 259.1572. Found: 259.1570. Anal. Calcd for C16H21NO2: C, 74.10%; H, 8.16%; N, 5.40%. Found: 74.17%; H, 8.19%; N, 5.35%. 1H-NMR (300 MHz, CDCl3) δ ppm 7.19 (d, J = 7.8 Hz, 1H), 7.14 (dd, J = 7.8, 1.8 Hz, 1H), 7.10 (d, J = 1.8 Hz, 1H), 3.87 (s, 3H), 3.10 (dd, J = 10.7, 8.9 Hz, 1H), 2.79 (ddd, J = 11.9, 10.7, 8.9 Hz, 1H), 2.68, (ddd, 11.9, 8.9, 2.7 Hz, 1H), 2.53, (ddd, J = 11.4, 10.7, 8.9 Hz, 1H), 2.17 (dtd, J = 11.4, 8.9, 2.7 Hz, 1H), 1.15 (s, 3H), 0.95 (s, 3H), 0.76 (bs, 1H, D2O). 13C-NMR (75 MHz, CDCl3) δ ppm 158.08, 132.95, 131.08, 127.46, 124.41, 119.34, 109.95, 71.54, 55.62, 55.42, 42.08, 30.10, 27.26, 27.01, 20.26, 15.84.
l-2-(2-Hydroxypropan-2-yl)-1-(3-methoxyphenyl)cyclobutanecarbonitrile (5etrans) IR (film): νmax/cm-1 3468, 2967, 2917, 2848, 2230 (CN), 1602, 1584, 1491, 1464, 1433, 1318, 1293, 1252, 1169, 1044, 948, 806, 784, 702. EIMS m/z: 245 (3%, M+), 227 (3), 202 (30), 173 (20), 159 (100), 147 (28), 133 (12), 129 (17), 116 (15), 89 (15), 77 (5), 71 (17). HRMS m/z Calculated for C15H19NO2: 245.1416. Found: 245.1413. Anal. Calcd for C15H19NO2: C, 73.44%; H, 7.81%; N, 5.71%. Found: 73.24%; H, 7.79%; N, 5.65%. 1H-NMR (300 MHz, CDCl3) δ ppm 7.36 (dd, J = 8.3, 7.7 Hz, 1H), 7.25, (dd, 7.7, 1.9 Hz, 1H), 7.20 (dd, J = 2.3, 1.9 Hz, 1H), 6.90 (dd, J = 8.3, 2.3 Hz, 1H), 3.84, (s, 3H), 3.13 (dd, J = 10.8, 8.8 Hz, 1H), 2.78 (ddd, J = 11.8, 10.8, 8.8 Hz, 1H), 2.68 (ddd, J = 11.7, 8.8, 2.5, Hz, 1H), 2.53 (ddd, J =11.4, 10.8, 8.8 Hz, 1H), 2.17 (dtd, J = 11.4, 8.8, 2.5 Hz, 1H), 1.15 (s, 3H), 0.95 (s, 3H), 0.71 (s, 1H, D2O). 13C-NMR (75 MHz, CDCl3) δ ppm 159.96, 136.03, 130.14, 124.20, 120.03, 114.41, 113.42, 71.55, 55.66, 55.35, 42.05, 30.00, 27.25, 27.00, 20.25.
l-1-(2,5-Dimethoxy-4-methylphenyl)-2-(2-hydroxypropan-2-yl)cyclobutanecarbonitrile (5ftrans) mp: 84-85 ºC. IR (KBr): νmax/cm-1 3523, 3491, 3391, 2972, 2853, 2230 (CN), 1504, 1468, 1398, 1216, 1047, 857, 786. EIMS: m/z 289 (5%, M+), 271 (2), 246 (2), 203 (100), 188 (34), 173 (5), 160 (3), 99 (3), 77 (2), 71 (3). HRMS m/z calculated for C17H23NO3 (M+): 289.1678. Found: 289.1674. Anal. Calcd. for C17H23NO3: C, 70.56%; H, 8.01%; N, 4.84%. Found: 70.62%; H, 7.98%; N, 4.86%. 1H-NMR (300 MHz, CDCl3) δ ppm 6.82 (s, 1H), 6.74 (s, 1H), 3.93 (s, 3H), 3.81 (s, 3H), 3.16 (ddd, J = 8.5, 6.9, 2.0 Hz, 1H), 2.92 (ddd, J = 11.4, 9.4, 6.7 Hz, 1H), 2.81 (s, 1H, D2O), 2.61 (dddd, J = 11.4, 8.5, 6.0, 2.0 Hz, 1H), 2.47 (dtd, J = 11.6, 8.5, 6.7 Hz, 1H), 2.24 (s, 3H), 1.96 (dddd, J = 11.6, 9.4, 6.9, 6.0 Hz, 1H), 1.04 (s, 3H), 0.81 (s, 3H). 13C-NMR (101 MHz, CDCl3) δ ppm 152.58, 150.63, 128.65, 124.95, 121.92, 116.03, 109.85, 71.60, 56.61, 56.26, 56.21, 39.44, 29.19, 28.39, 24.92, 21.02, 16.20.
u-3-Hydroxy-2,2-dimethyl-1-phenylcyclopentanecarbonitrile (6atrans) IR (film): νmax/cm-1 3436, 2966, 2877, 2232 (CN), 1496, 1470, 1448, 1386, 1370, 1102, 1084, 1030, 767, 743, 699. EIMS: m/z 215 (16%, M+), 197 (1), 172 (6), 156 (2), 143 (3), 129 (18), 115 (16), 103 (12), 86 (100), 77 (10), 71 (90). HRMS m/z Calculated for C14H17NO: 215.1310. Found: 215.1306. Anal. Calcd. for C14H17NO: C, 78.10%; H, 7.96%; N, 6.51%. Found: 78.12%; H, 7.94%; N, 6.52%. 1H-NMR (300 MHz, CDCl3) δ ppm 7.49-7.31 (m, 5H), 4.37 (dd, J = 8.8, 8.0 Hz, 1H), 2.75 (ddd, J = 13.7, 11.9, 6.3 Hz, 1H), 2.45 (dddd, J = 13.4, 9.6, 8.8, 6.3 Hz, 1H), 2.27 (ddd, J = 13.7, 9.6, 4.1 Hz, 1H), 2.11 (bs, 1H, D2O), 1.79 (dddd, J = 13.4, 11.9, 8.0, 4.1 Hz, 1H), 1.20 (s, 3H), 0.58 (s, 3H). 13C-NMR (75 MHz, CDCl3) δ ppm 134.75, 128.30 (2C), 128.05, 127.43 (2C), 123.72, 79.26, 53.99, 48.98, 30.42, 28.72, 22.26, 15.76.
u-3-Hydroxy-2,2-dimethyl-1-p-tolylcyclopentanecarbonitrile (6btrans) IR (Film): νmax/cm-1 3436, 3029, 2964, 2930, 2877, 2232 (CN), 1515, 1469, 1452, 1385, 1370, 1100, 1072, 1034, 1021, 814, 528. EIMS m/z: 229 (39%, M+), 211 (20), 196 (21), 169 (12), 157 (11), 143 (100), 128 (6), 115 (28), 91 (17), 86 (48), 77(5), 71 (32). HRMS m/z Calculated for C15H19NO: 229.1467. Found: 229.1459. Anal. Calcd for C15H19NO: C, 78.56%; H, 8.35%; N, 6.11%. Found: 78.60%; H, 8.39%; N, 6.05%. 1H-NMR (300 MHz, CDCl3) δ ppm 7.33 (d, J = 8.5 Hz, 2H), 7.19 (d, J = 8.5 Hz, 2H), 4.36 (dd, J = 9.1, 7.8 Hz, 1H), 2.71 (ddd, J = 13.7, 12.0, 6.3 Hz, 1H), 2.44 (dddd, J = 13.5, 9.6, 9.1, 6.3 Hz, 1H), 2.36 (s, 3H), 2.25 (ddd, J = 13.7, 9.6, 4.1 Hz, 1H), 2.13 (bs, 1H, D2O), 1.78 (dddd, J = 13.5, 12.0, 7.8, 4.1 Hz, 1H), 1.18 (s, 3H), 0.58 (s, 3H). 13C-NMR (75 MHz, CDCl3) δ ppm 137.87, 131.66, 128.97 (2C), 127.31 (2C), 123.84, 79.28, 53.66, 48.86, 30.40, 28.66, 22.19, 20.90, 15.70.
u-1-(3,4-Dimethoxyphenyl)-3-hydroxy-2,2-dimethylcyclopentanecarbonitrile (6ctrans) IR (Film): νmax/cm-1 3484, 2964, 2937, 2837, 2231 (CN), 1663, 1590, 1520, 1466, 1413, 1329, 1262, 1151, 1026, 854, 809, 758. EIMS m/z: 275 (6%, M+), 203 (2), 189 (100), 174 (10), 146 (2), 128 (2), 119 (5), 103 (2), 91 (2), 77 (2). HRMS m/z Calculated for C16H21NO3: 275.1521. Found: 275.1513. Anal. Calcd for C16H21NO3: C, 69.79%; H, 7.69%; N, 5.09%. Found: 69.60%; H, 7.67%; N, 5.03%. 1H-NMR (300 MHz, CDCl3) δ ppm 7.00 (d, J = 8.2, 2.2 Hz, 1H), 6.96 (d, J = 2.2 Hz, 1H), 6.87 (d, J=8.2 Hz, 1H), 4.36 (dd, J = 8.8, 7.8 Hz, 1H), 3.91 (s, 3H), 3.90 (s, 3H), 2.68 (ddd, J = 13.6, 11.8, 6.3 Hz, 1H), 2.44 (dddd, J = 13.3, 9.5, 8.8, 6.3 Hz, 1H), 2.28 (ddd, J = 13.6, 9.5, 3.9 Hz, 1H), 2.12 (s, 1H), 1.78 (dddd, J = 13.3, 11.8, 7.8, 3.9 Hz, 1H), 1.21 (s, 3H), 0.60 (s, 3H). 13C-NMR (75 MHz, CDCl3) δ ppm 148.76, 148.47, 127.10, 123.79, 119.70, 111.04, 110.55, 79.19, 55.98, 55.85, 53.58, 48.96, 30.61, 28.66, 22.32, 15.78.
u-3-Hydroxy-1-(3-methoxy-4-methylphenyl)-2,2-dimethylcyclopentanecarbonitrile (6dtrans) mp 71-72 ºC. IR (KBr): νmax/cm-1 3437, 2963, 2835, 2232 (CN), 1612, 1582, 1514, 1466, 1407, 1261, 1164, 1142, 1102, 1074, 1037, 846, 814, 734, 648.. EIMS m/z: 259 (8%, M+), 241 (2), 216 (2), 187 (4), 173 (100), 158 (12), 115 (6), 103 (5), 91 (5), 77 (4), 71 (3). HRMS m/z Calculated for C16H21NO2: 259.1572. Found: 259.1565. Anal. Calcd for C16H21NO2: C, 74.10%; H, 8.16%; N, 5.40%. Found: 74.13%; H, 8.21%; N, 5.39%. 1H-NMR (300 MHz, CDCl3) δ ppm 7.13 (d, J = 7.9 Hz, 1H), 6.91 (dd, J = 7.9, 1.9 Hz, 1H), 6.90 (d, J = 1.9 Hz, 1H), 4.36 (dd, J = 8.8, 7.9 Hz, 1H), 3.86 (s, 3H), 2.70 (ddd, J = 13.6, 11.9, 6.2 Hz, 1H), 2.46 (dddd, J = 13.4, 9.6, 8.8, 6.2 Hz, 1H), 2.27 (ddd, J = 13.6, 9.6, 4.0 Hz, 1H), 2.22 (s, 3H), 1.89 (bs, 1H, D2O), 1.78 (dddd, J = 13.4, 11.9, 7.9, 4.0 Hz, 1H), 1.22 (s, 3H), 0.60 (s, 3H). 13C-NMR (75 MHz, CDCl3) δ ppm 157.49, 133.50, 130.19, 126.75, 123.81, 119.12, 109.68, 79.37, 55.39, 54.00, 48.96, 30.63, 28.81, 22.41, 15.86, 15.78.
u-3-Hydroxy-1-(3-methoxyphenyl)-2,2-dimethylcyclopentanecarbonitrile (6etrans) IR (film): νmax/cm-1 3349, 2964, 2878, 2838, 2232 (CN), 1602, 1584, 1493, 1469, 1433, 1294, 1264, 1165, 1107, 1049, 1034, 782, 698. EIMS: 245 (8%, M+), 202 (2), 159 (100), 147 (4), 133 (4), 129 (5), 86 (9), 77 (4), 71 (14). HRMS m/z Calculated for C15H19NO2: 245.1416. Found: 245.1414. Anal. Calcd for C15H19NO2: C, 73.44%; H, 7.81%; N, 5.71%. Found: 73.27%; H, 7.78%; N, 5.66%. 1H-NMR (300 MHz, CDCl3) δ ppm 7.31 (dd, J = 8.2, 7.8 Hz, 1H), 7.04 (dd, J = 7.8, 2.0 Hz, 1H), 6.99 (dd, J = 2.3, 2.0 Hz, 1H), 6.88 (ddd, J = 8.24, 2.3 Hz, 1H), 4.36 (dd, J = 9.0, 7.8 Hz, 1H), 3.83 (s, 3H), 2.71 (ddd, J = 13.7, 11.8, 6.3 Hz, 1H), ), 2.45 (dddd, J = 13.4, 9.6, 9.0, 6.3 Hz, 1H), 2.27 (ddd, J = 13.7, 9.6, 4.0 Hz, 1H), 1.78 (dddd, J = 13.4, 11.8, 7.8, 4.00 Hz, 1H), 1.78 (bs, 1H, D2O), 1.22 (s, 3H), 0.60 (s, 3H). 13C-NMR (75 MHz, CDCl3) δ ppm 159.44, 136.40, 129.27, 123.61, 119.84, 114.12, 112.84, 79.34, 55.32, 53.94, 49.01, 30.51, 28.77, 22.37, 15.84.
l-1-(2,5-Dimethoxy-4-methylphenyl)-3-hydroxy-2,2-dimethylcyclopentanecarbonitrile (6f) mp: 98-98.5 ºC. IR (KBr): νmax/cm-1 3471, 2973, 2947, 2917, 2226 (CN), 1516, 1469, 1395, 1375, 1288, 1217, 1096, 1044, 856, 785, 714. EIMS: m/z 289 (18%, M+), 256 (2), 217 (2), 203 (100), 188 (23), 173 (2), 152 (2), 91 (2), 77 (2). HRMS m/z calculated for C17H23NO3 (M+): 289.1678. Found: 289.1676. Anal. Calcd for C17H23NO3: C, 70.56%; H, 8.01%; N, 4.84%. Found: 70.61%; H, 8.05%; N, 4.81%. 1H-NMR (300 MHz, CDCl3) δ ppm 6.80 (s, 1H), 6.78 (s, 1H), 4.23 (dd, J = 7.6, 6.1 Hz, 1H), 3.82 (s, 3H), 3.80 (s, 3H), 2.99 (ddd, J = 13.2, 10.1, 7.5 Hz, 1H), 2.43 (tdd, J = 13.2, 8.7, 7.5 Hz, 1H), 2.28 (ddd, J = 13.2, 8.7, 3.9 Hz, 1H) , 2.22 (s, 3H), 1.8 (dddd, J = 13.2, 10.1, 6.1, 3.9 Hz, 1H), 1.75 (bs, 1H, D2O), 1.31 (s, 3H), 0.67 (s, 3H). 13C-NMR (101 MHz, CDCl3) δ ppm 151.74, 151.53, 127.93, 123.84, 121.75, 116.03, 111.55, 80.09, 56.25, 56.18, 51.63, 49.88, 32.83, 29.89, 25.23, 17.32, 16.02.
ACKNOWLEDGEMENTS
This research was supported by Facultad de Química UNAM, and the CONACYT through a PhD scholarship for J. A. Luján-Montelongo. We wish to thank to Rosa Del Villar, Nuria Esterau, Marisela Gutiérrez, Margarita Guzmán, Nayeli López and Georgina Duarte for their assistance in acquiring the spectral data, and Christopher Pulichene for helpful suggestion this manuscript.
References
1. a) J. Gyimesi and A. Melera, Tetrahedron Lett,. 1967, 17, 1665; CrossRef b) T. Masamune, S. Sato, A. Abiko, M. Ono, and A. Murai, Bull. Chem. Soc. Jpn., 1980, 53, 2895; CrossRef c) M. Ando, K. Wada, and K. Takase, Tetrahedron Lett., 1985, 26, 235; CrossRef d) K. Ishii, T. Nakano, T. Zenko, M. Kotera, and M. Sakamoto, J. Chem. Soc., Perkin Trans. 1, 1991, 2057; CrossRef e) Y. Mori, K. Yaegashi, and H. Furukawa, J. Am. Chem. Soc., 1996, 118, 8158; CrossRef f) C. Beier and E. Schaumann, Synthesis, 1997, 11, 1296; CrossRef g) T. Kakuchi, R. Nonokawa, S. Umeda, T. Satoh, and K. Yokota, Macromolecules, 2000, 33, 246; CrossRef h) Y. Cui, Y. Dang, Y. Yang, and R. Ji, J. Heterocycl. Chem., 2006, 43, 1071. CrossRef
2. a) P. Cruickshank and M. Fishman, J. Org. Chem., 1969, 34, 4060; CrossRef b) M. Kodama, Y. Matsuki, and S. Itô, Tetrahedron Lett., 1976, 14, 1121; CrossRef c) B. Corbel and T. Durst, J. Org. Chem., 1976, 41, 3648; CrossRef d) R. Maurya and E. Ghera, Tetrahedron Lett., 1990, 31, 5179; CrossRef e) D. F. Tabber, L. J. Silverberg, and E. D. Robinson, J. Am. Chem. Soc., 1991, 113, 6639; CrossRef f) K. Afarinkia and F. Mahmood, Tetrahedron Lett., 2000, 41, 1287; CrossRef One example of a carbon based nucleophile used in nucleophilic intramolecular displacement leading to an heterocycle: g) C. K. Bradsher and D. C. Reames, J. Org. Chem., 1978, 43, 3800. CrossRef
3. a) G. Stork and J. F. Cohen, J. Am. Chem. Soc., 1974, 96, 5270; CrossRef b) P. Garratt, C. W. Doecke, J. C. Weber, and L. A. Paquette, J. Org. Chem., 1986, 51, 449; CrossRef c) A. Wahhab, M. Ottosen, and F. W. Bachelor, Can. J. Chem., 1991, 69, 570; CrossRef d) L. Lambs, N. P. Singh, and J. Biellmann, Tetrahedron Lett., 1991, 32, 2637; CrossRef e) I. Petschen, A. Parrilla, M. P. Bosch, C. Amela, A. A. Botar, F. Camps, and A. Guerrero, Chem. Eur. J., 1999, 5, 3299; CrossRef f) J. G. Avila-Zárraga and L. A. Maldonado, Chem. Lett., 2000, 512; CrossRef g) J. Wang, S. Sakamoto, K. Kamada, A. Nitta, T. Noda, H. Oguri, and M. Hirama. Synlett, 2003, 6, 891; CrossRef h) T. Hübscher and G. Helmchen, Synlett, 2006, 9, 1323. CrossRef
4. a) A. Fernández-Mateos, L. Mateos Buón, R. Rabanedo Clemente, A. I. Ramos Silvo, and R. Rubio González, Synlett, 2004, 6, 1011; CrossRef b) L. M. Monleón, M. Grande, and J. Anaya, Tetrahedron, 2007, 63, 3017; CrossRef c) A. Fernández-Mateos, P. Herrero Teijn, L. Mateos Burn, R. Rabanedo Clemente, and R. Rubio González, J. Org. Chem., 2007, 72, 9973; CrossRef d) L. Monleón, M. M. Grande, and J. Anaya, Synlett, 2007, 8, 1243; CrossRef e) A. Fernández-Mateos, P. Herrero Teijón, R. Rabanedo Clemente, R. Rubio González, and F. Sanz González, Synlett, 2007, 17, 2718. CrossRef
5. J. E. Baldwin, J. Chem. Soc., Chemm. Commun., 1976, 734. CrossRef
6. J. Y. Lallemand and M. Onanga, Tetrahedron Lett. 1975, 8, 585. CrossRef
7. See references 3a-b, 3d-e, and 3g-h.
8. G. Stork, L. D. Cama, and D. R. Coulson, J. Am. Chem. Soc., 1974, 96, 5268. CrossRef
9. F. F. Fleming and B. C. Shook, Tetrahedron, 2002, 58, 1. CrossRef
10. Besides the pioneering work of Stork on δ-epoxynitriles (ref 8), no attemps were made for the synthetic exploit of this reaction in long time.
11. F. F. Fleming, Y. Wei, W. Liu, and Z. Zhang, Tetrahedron, 2008, 64, 7477, and references included within. CrossRef
12. Selected examples of the cuparane family: a) C. Enzell and H. Erdtman, Tetrahedron, 1958, 4 361; CrossRef b) B. Tomita, Y. Hirose, and Y. Nakatsuka, Tetrahedron Lett., 1968, 7, 843; CrossRef c) G. Chetty and S. Dev, Tetrahedron Lett., 1964, 73; CrossRef d) N. Ishikawa, K. Yamaji, S. Tahara, Y. Fukushi, and K. Takahashi, Phytochemistry, 2000, 54, 777; CrossRef e) J. D. Bu'lock and J. Darbyshire, Phytochemistry, 1976, 15, 2004; CrossRef f) S. Natori, H. Nishikawa, and H. Ogawa, Chem. Pharm. Bull., 1964, 12, 236. CrossRef
13. Compounds 1a, 1b, 1c, and 1e are commercially available from Sigma-Aldrich. Compounds 1d and 1f were prepared as described on experimental section.
14. W. Biernacki and A. Gdula, Synthesis, 1979, 37. CrossRef
15. See experimental section.
16. Refluxing temperatures are needed for the complete conversion of the carbocycles. When the reaction is carried out at temperatures below 50 ºC, transformation does not occur.
17. This can be rationalized in terms of the oxygen’s affinity for lithium and potassium over sodium. In fact the experimental and theoretical ΔHf values of their corresponding oxide or hydroxide show that preference. See M. B. Sullivan, M. A. Iron, P. C. Redfern, J. M. L. Martin, L. A. Curtiss, and L. Radom, J. Phys. Chem. A, 2003, 107, 5617. CrossRef
18. It is well known that oxirane rings present increased electrophilicity when acid entities are coordinated to oxygen. It also could contribute for a more advanced transition state leading to an attack to the more substituted position. For a general, easily comprehensible explanation about this see F. A. Carey and R. J. Sundberg, ‘Advanced Organic Chemistry, Part B: Reactions and Synthesis’, Fourth Edition, Kluwer Academic – Plenum Publishers, New York, 2001, pp. 773-774.
19. In general, hydrocarbon solvents favor N-metalation; see F. F. Fleming and B. C. Shook, J. Org. Chem., 2002, 67, 2885. CrossRef
20. We tried also to use HMDS-M+ bases with the mentioned trialkylamine solvents but results were poor either in regioselectivity or yields.