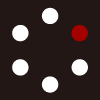
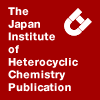
HETEROCYCLES
An International Journal for Reviews and Communications in Heterocyclic ChemistryWeb Edition ISSN: 1881-0942
Published online by The Japan Institute of Heterocyclic Chemistry
e-Journal
Full Text HTML
Received, 2nd February, 2009, Accepted, 5th March, 2009, Published online, 9th March, 2009.
DOI: 10.3987/COM-09-S(S)8
■ Synthesis of 4-Aryl-Substituted Butenolides and Pentenolides by Copper-Catalyzed Hydroarylation
Yoshihiko Yamamoto* and Naohiro Kirai
Graduate School of Engineering, Nagoya University, Chikusa, Nagoya, Aichi 464-8601, Japan
Abstract
We have investigated the effect of the type of protecting group used and the tether length of 4- and 5-hydroxy-substituted 2-alkynoates on the yields and selectivity of the product of hydroarylation. The use of methyl, methoxymethyl, and tert-butyldimethylsilyl (TBS) as protecting groups increased the total yield of the products. While the protected 4-hydroxy-2-butynoates afforded products formed from two or more alkyne substrates, 5-hydroxy-2-pentynoate derivatives exclusively yielded 1:1 adducts. These facts suggest that the product selectivity depends on the distance of the alkoxy group from the alkyne moiety rather than the type of the protecting group. Among the protecting groups used in this study, the TBS group was found to produce butenolides and pentenolides in good total yields via the one-pot hydroarylation/lactonization.INTRODUCTION
Furan-2(5H)-one (butenolide) is a privileged oxygen heterocycle, which is widely distributed in nature, and its naturally occurring derivatives exhibit interesting biological activities. Therefore, a considerable number of studies have been devoted to the development of an efficient and versatile method to synthesize butenolides.1 In contrast, 5,6-dihydro-2H-pyran-2-one (pentenolide), which is homologous to butenolide, has not received significant attention, even though this structural motif found in natural products is also exhibit bioactivity.2
Recently, we have reported that the Cu-catalyzed hydroarylation of alkynoates with arylboronic acids stereoselectively produced trisubstituted cinnamate derivatives in good yields.3 On the basis of the success of the above study, we envisaged that by applying this method to alkynoate substrates with a hydroxy terminal, we can obtain unsaturated lactones via concomitant cyclization between the hydroxy and ester carbonyl groups. In fact, we obtained 4-phenylbutenolide (3a), albeit in a moderate yield of 61%, when methyl 4-hydroxy-2-butynoate (1a) reacted with phenylboronic acid (2a) under our optimal conditions (Scheme 1). As an extension of this sequential hydroarylation/lactonization process, we also developed the synthetic method for 4-arylcoumarins, which constitute a subgroup of flavonoids (neoflavones), using phenol-derived propiolates as substrates.4 During this study, we found that the undesired 4-methoxycoumarin is produced in a significant yield along with the formation of 4-phenylcoumarin, by carrying out the reaction between 2a and methyl 3-(2-hydroxyphenyl)propiolate (Scheme 1). In contrast, when substrates protected with an easily removable methoxymethyl (MOM) group were used, the desired 4-arylcoumarins were selectively produced in high yields after the acidic deprotection of the MOM group in one pot. This result indicated the importance of the protection of the hydroxy moiety in the substrates. In this paper, we report the results of the synthesis of 4-aryl-substituted butenolides and pentenolides by Cu-catalyzed hydroarylation, with the emphasis on the effect of protecting groups on the yields and selectivity of the products.5,6
RESULTS AND DISCUSSION
At the outset of this study, we revisited the Cu-catalyzed hydroarylation of alkynoates with a terminal hydroxy group. As mentioned above, the hydroarylation of the alkyne triple bond in 1a and the subsequent lactonization of the resultant adduct resulted in the formation of 3a in 61% yield. We then carried out this reaction using phenyl-substituted butynoate 1b as the substrate to obtain the corresponding 4,5-diphenylbutenolide 3b in a yield of 59%, which is comparable to the above reaction (Scheme 1). In order to examine the effect of the tether length between the alkyne and hydroxy moieties on the reaction, we carried out the Cu-catalyzed hydroarylation of 5-hydroxy-2-pentynoate 1c and obtained 4-phenylpentenolide 4a in 55% yield as the sole product (Scheme 2). In contrast to the result obtained from 3-(2-hydroxyphenyl)propiolate with the same tether length (Scheme 1), the corresponding 4-methoxypentenolide was not formed from 1c.
We then studied the hydroarylation of substrates with protected hydroxy groups with the aim of improving the product yields. First, we carried out the hydroarylation of 4-methoxy-2-butynoate 5a with 3 equivalents of phenylboronic acid 2a (Scheme 3). After stirring the reaction mixture for 1 h, the products were purified by column chromatography on silica gel to afford the desired 1:1 adduct 6a along with an unexpected 2:1 adduct 7a in yields of 57% and 32%, respectively.7 The total yield of 89% obtained by this method is much higher than that of 3a (61%) obtained by using the unprotected substrate 1a (Scheme 1). Therefore, the protection of the terminal hydroxy moiety enhances the product yield. The unexpected formation of 7a is ascribed to the strong bidentate coordination of 5a to a presumed vinyl copper intermediate (Scheme 4). As a result, the second molecule of 5a was incorporated into the C–Cu bond prior to methanolysis of the vinyl copper species. We believed that if this were the case, then by using cyclic boronate 2a’, the rate of methanolysis must be lowered, resulting in an increase in the yield of 7a. Therefore, to verify the above speculation, we repeated the reaction with 1 equivalent of 2a’ with stirring for 3 h and obtained 7a as a major product in 59% yield along with 6a in ca. 20% yield. Moreover, it is worth noting that the geometries of both the alkene moieties in 7a have been demonstrated to be controlled perfectly via syn carbocupration.
With the aim of obtaining the corresponding butenolides, we examined the use of different easily removable protecting groups. First, MOM-protected 5b was allowed to react with 1 equivalent of phenylboronate 2a’ for 2 h by following the same procedure as above to obtain a 2:1 adduct 7b as the major product along with a 1:1 adduct 6b in yields of 63% and 12%, respectively (Scheme 5). In this case, a 3:1 adduct 8b was also obtained in 15% yield, suggesting that the two oxygen atoms of the MOM protecting group enhance the coordination ability of 5b and hence facilitate its incorporation into the C–Cu bond. We then attempted to carry out lactonization of the obtained products by the removal of MOM groups under acidic conditions (6 M HCl in MeOH). However, the reaction was sluggish at ambient temperature and led to a low total yield of less than 25%. The deprotection reaction at elevated temperature, on the other hand, resulted in the decomposition of products.
The use of tert-butyldimethylsilyl (TBS) instead of MOM as the protecting group proved to be advantageous (Scheme 6). The hydroarylation of 4-siloxy-2-butynoate 5c with 3 equivalents of 2a was carried out by stirring for 6 h under optimal conditions to produce 1:1 and 2:1 adducts, which were detected by TLC analysis. When the reaction mixture was treated with 6 M HCl in the same reaction vessel, the deprotection effectively took place even at ambient temperature for 1 h, and butenolide 3a was obtained as the major product in 56% yield along with bis(butenolide) 9 in 31% yield.8 In contrast, when this reaction was carried out using 1 equivalent of phenylboronate 2a’ instead of 2a with stirring for 24 h, 9 was obtained as the major product in 58% yield along with 3a in 21% yield (Scheme 6).
Finally, we investigated the use of this one-pot Cu-catalyzed hydroarylation/lactonization method for the synthesis of 4-arylpentenolides 4 from 5-silyloxy-2-pentynoate 5d (Scheme 7 and Table 1). In the presence of 2 mol % CuOAc, 5d was treated with 3 equivalents of phenylboronic acid 2a in MeOH at 28
˚C for 3 h and subsequent acidic removal of the TBS group at room temperature for 1 h afforded the desired product 4-phenylpentenolide 4a in a high yield of 86% (Table 1, entry 1). In the case of the homopropargyl system 5d, it was found that only 1:1 coupling of the alkyne and arylboronic acid occurred, indicating that the ease of the insertion of the second alkyne molecule is influenced by the tether length between the alkyne and silyloxy moieties rather than the protecting groups used. In fact, when the methoxy analogue of 5d, i.e., 5e, was subjected to hydroarylation with 2a, it afforded a 1:1 adduct 10 in a moderate yield (Scheme 8); this result supports the above inference. We have previously reported that a bishomo congener such as methyl 6-methoxy-2-hexynoate also exclusively yields a 1:1 adduct on hydroarylation.3
In order to demonstrate the feasibility of this method, the performance of different substituted arylboronic acids was also examined, and the results are summarized in Table 1. p-Tolylboronic acid 2b underwent hydroarylation to afford 4b in a high yield comparable to that obtained in the case of 2a; however, a longer reaction time of 6 h was required (Table 1, entry 2). The hydroarylation using sterically demanding o-tolylboronic acid did not proceed to completion for 24 h, even though an increased amount of catalyst was employed (6 mol %). It was found that this one-pot hydroarylation/lactonization method gave good results with arylboronic acids that possess both electron-donating and electron-withdrawing substituents such as 2c–e (entries 3–5); although in the cases of electron-deficient arylboronic acids 2d and 2e, an increased amount of copper catalyst was required.
In conclusion, we have demonstrated that the use of protecting groups such as methyl, MOM, and TBS increased the yields of hydroarylation, and the product selectivity mainly depends on the distance between the alkoxy group and the alkyne moiety rather than the type of protecting group. Among the protecting groups used in this study, the TBS group was found to produce butenolides and pentenolides in high total yields by the proposed one-pot hydroarylation/lactonization method.
EXPERIMENTAL
Column chromatography was performed on silica gel (Cica silica gel 60N) with mixed solvents [hexane / AcOEt]. 1H and 13C NMR spectra were obtained on a Varian Gemini 2000 NMR spectrometer for samples in CDCl3 solutions at 25 ˚C. 1H NMR chemical shifts are reported in terms of chemical shift (δ, ppm) relative to the singlet at 7.26 ppm for chloroform. Splitting patterns are designated as follows: s, singlet; d, doublet; t, triplet; q, quartet; quint, quintet; sext, sextet; sept, septet; m, multiplet. Coupling constants are reported in Hz. 13C NMR spectra were fully decoupled and are reported in terms of chemical shift (δ, ppm) relative to the triplet at 77.0 ppm for CDCl3. EI mass measurements were performed with a Shimadzu GCMS-QP2010 plus mass spectrometer. ESI mass spectra were recorded on a JEOL JMS-T100CS mass spectrometer as a MeOH solution. Elemental analyses were performed at Center for Advanced Materials Analysis of Tokyo Institute of Technology. Melting points were obtained in capillary tubes. All solvents and CuOAc were purchased and used as received. Alkynoates 1b,9a 1c,9b 5a,9c 5b,9c 5c,9b 5d,9d and 5e9e were prepared according to the procedures reported in the literatures. Products 3a,10a 3b,10b and 4a10c are known compounds.
Representative Procedure for Cu-catalyzed Hydroarylation of Alkynoates with Phenyloronic Acid: Synthesis of Butenolide 3b from Alkynoate 1b and Phenylboronic acid 2a. To a solution of 1b (95 mg, 0.5 mmol) and 2a (183 mg, 1.5 mmol) in MeOH (1.0 mL) was added CuOAc (1.23 mg, 0.01 mmol). The reaction mixture was degassed at –78 ˚C, and then stirred at 28 ˚C under Ar atmosphere for 24 h. After filtration through a pad of celite to remove insoluble materials, the filtrate was concentrated in vacuo. The residue was purified by silica gel flash column chromatography (hexane/AcOEt 10:1–5:1) to give 4,5-diphenyl-2(5H)-furanone (3b) (70 mg, 59%) as colorless solids (mp 154–157 ˚C, lit.,10b mp 147.5–150 ˚C): 1H NMR (300 MHz, CDCl3): δ 6.33 (d, J = 1.6 Hz, 1 H), 6.55 (d, J = 1.6 Hz, 1 H), 7.30–7.43 (m, 10 H); 13C NMR (75 MHz, CDCl3): δ 84.1, 114.4, 127.4, 127.7, 128.8, 129.0, 129.4, 129.5, 131.1, 134.8, 165.7, 172.4.
4-Phenyl-5,6-dihydro-2H-pyran-2-one (4a): colorless solid (mp 55–57 ˚C, lit.,10c mp 56–58 ˚C); 1H NMR (300 MHz, CDCl3): δ 2.87 (dt, J = 6.0, 0.9 Hz, 2 H), 4.54 (t, J = 6.0 Hz, 2 H), 6.38 (t, J = 0.9 Hz, 1 H), 7.44–7.48 (m, 3 H), 7.53–7.57 (m, 2 H); 13C NMR (75 MHz, CDCl3): δ 26.2, 65.9, 114.9, 125.9, 128.9, 130.6, 135.9, 155.2, 164.8.
Methyl 4-methoxy-3-phenylbut-2-enoate (6a): colorless oil; 1H NMR (300 MHz, CDCl3): δ 3.33 (s, 3 H), 3.77 (s, 3 H), 4.94 (d, J = 0.9 Hz, 2 H), 6.21 (d, J = 0.9 Hz, 1 H), 7.35–7.41 (m, 3 H), 7.49–7.53 (m, 2 H); 13C NMR (75 MHz, CDCl3): δ 51.2, 58.0, 67.9, 119.4, 127.0, 128.3, 129.0, 138.7, 154.9, 166.2; MS (EI): m/z (%): 206 (87) [M+], 191 (23) [M+ – CH3], 174 (36) [M+ – CH3OH], 159 (100) [M+ – CH3OH – CH3]; Anal. Calcd (%) for C12H14O3 (206.09): C 69.88; H 6.84. Found: C 69.83; H 6.83.
Dimethyl 4-(2-methoxy-1-phenylethylidene)-3-(methoxymethyl)pent-2-enedioate (7a): colorless oil; 1H NMR (300 MHz, CDCl3): δ 3.31 (s, 3 H), 3.33 (s, 3 H), 3.57 (s, 3 H), 3.79 (s, 3 H), 4.41 (d, J = 2.1 Hz, 2 H), 4.55 (s, 2 H), 5.50 (t, J = 2.1 Hz, 1 H), 7.21–7.34 (m, 5 H); 13C NMR (75 MHz, CDCl3): δ 51.0, 51.9, 58.1, 58.8, 71.3, 72.5, 120.8, 127.9, 128.0, 128.2, 132.5, 138.8, 148.0, 155.4, 165.7, 167.0; MS (ESI): calcd for C18H22O6 (334.14): found m/z 357.05 [M + Na]+; Anal. Calcd (%) for C18H22O6 (334.36): C 64.66; H 6.63. Found: C 64.66; H 6.71.
Methyl 5-methoxy-3-phenylpent-2-enoate (10): colorless oil; 1H NMR (300 MHz, CDCl3): δ 3.30 (s, 3 H), 3.37–3.42 (m, 2 H), 3.48–3.53 (m, 2 H), 3.76 (s, 3 H), 6.12 (s, 1 H), 7.36–7.40 (m, 3 H), 7.45–7.50 (m, 2 H); 13C NMR (75 MHz, CDCl3): δ 31.6, 51.2, 58.4, 71.3, 118.3, 126.7, 128.5, 129.0, 141.1, 157.3, 166.6; MS (EI): m/z (%): 220 (10) [M+], 188 (100) [M+ – CH3OH], 175 (62) [M+ – CH2OCH3], 157 (28) [M+ – CH3OH – OCH3]; Anal. Calcd (%) for C13H16O3 (220.26): C 70.89; H 7.32. Found: C 70.69; H 7.52.
Representative Procedure for Cu-catalyzed Hydroarylation of Protected Hydroxyalkynoates with Phenylboronate: Reaction of Alkynoate 5b and Phenylboronate 2a’. To a solution of 5b (633 mg, 4.0 mmol) and 2a’ (760 mg, 4.0 mmol) in MeOH (8.0 mL) was added CuOAc (9.81 mg, 0.08 mmol). The reaction mixture was degassed at –78 ˚C, and then stirred at 28 ˚C under Ar atmosphere for 2 h. After filtration through a pad of celite to remove insoluble materials, the filtrate was concentrated in vacuo. The residue was purified by alumina short column chromatography (hexane/AcOEt 1:2) to remove residual 2a’. The crude products were purified by silica gel flash column chromatography (hexane/AcOEt 20:1) to give methyl 4-(methoxymethoxy)-3-phenylbut-2-enoate (6b) (113 mg, 12%) as colorless oil: 1H NMR (300 MHz, CDCl3): δ 3.30 (s, 3 H), 3.77 (s, 3 H), 4.65 (s, 2 H), 5.08 (d, J = 0.3 Hz, 2 H), 6.20 (d, J = 0.3 Hz, 1 H), 7.36–7.42 (m, 3 H) , 7.49–7.54 (m, 2 H); 13C NMR (75 MHz, CDCl3): δ 51.3, 55.4, 63.5, 96.4, 119.4, 127.0, 128.3, 129.0, 138.9, 154.5, 166.1; MS (EI): m/z (%): (no molecular ion peak) 204 (23) [M+ – CH3OH], 191 (56) [M+ – CH2OCH3], 175 (33) [M+ – OCH2OCH3], 159 (100) [M+ – C6H5]; MS (ESI): calcd for C13H16O4 (236.10): found m/z 259.06 [M + Na]+; Anal. Calcd (%) for C13H16O4 (236.26): C 66.09; H 6.83. Found: C 66.05; H 6.87.
Further elution (hexane/AcOEt 3:1) gave dimethyl 4-(2-(methoxymethoxy)-1-phenylethylidene)-3- ((methoxymethoxy)methyl)pent-2-enedioate (7b) (497 mg, 63%) as colorless oil: 1H NMR (300 MHz, CDCl3): δ 3.25 (s, 3 H), 3.36 (s, 3 H), 3.57 (s, 3 H), 3.79 (s, 3 H), 4.60–4.62 (m, 6 H), 4.71 (s, 2 H), 5.49 (t, J = 2.0 Hz, 1 H), 7.25–7.30 (m, 5 H); 13C NMR (75 MHz, CDCl3): δ 51.2, 52.0, 55.3, 55.4, 66.9, 67.6, 96.1, 96.5, 121.5, 128.0, 128.2, 128.3, 132.8, 139.0, 148.3, 154.8, 165.7, 166.8; MS (ESI): calcd for C20H26O8 (394.16): found m/z 417.07 [M + Na]+; Anal. Calcd (%) for C20H26O8 (394.42): C 60.90; H 6.64. Found: C 60.71; H 6.83.
Further elution (hexane/AcOEt 1:1) gave trimethyl 7-(methoxymethoxy)-2,4-bis((methoxymethoxy)- methyl)-6-phenylhepta-1,3,5-triene-1,3,5-trioate (8b) (113 mg, 15%) as colorless gummy oil: 1H NMR (300 MHz, CDCl3): δ 3.18 (s, 3 H), 3.25 (s, 3 H), 3.37 (s, 3 H), 3.62 (s, 3 H), 3.70 (s, 3 H), 3.81 (s, 3 H), 4.16 (s, 2 H), 4.51 (s, 2 H), 4.55 (s, 2 H), 4.63 (s, 6 H), 5.80 (br s, 1 H), 7.29–7.31 (m, 5 H); 13C NMR (75 MHz, CDCl3): δ 51.2, 51.7, 52.0, 55.2, 55.4, 65.9, 67.3, 68.1, 95.8, 96.1, 96.3, 120.8, 128.0, 128.4, 128.5, 130.3, 132.2, 138.3, 144.6, 147.4, 151.7, 165.8, 166.3, 167.3; MS (ESI): calcd for C27H36O12 (552.22): found m/z 575.11 [M + Na]+; Anal. Calcd (%) for C27H36O12 (552.57): C 58.69; H 6.57. Found: C 58.73; H 6.52.
Representative Procedure for Cu-catalyzed One-pot Hydroarylation/lactonization of Silyloxyalkynoates: Reaction of 5c with Phenylboronic Acid 2a. To a solution of 5c (114 mg, 0.5 mmol) and 2a (183 mg, 1.5 mmol) in MeOH (1.0 mL) was added CuOAc (1.23 mg, 0.01 mmol). The reaction mixture was degassed at –78 ˚C, and then stirred at 28 ˚C under Ar atmosphere for 6 h. To this solution was added MeOH (2 mL) and 6 M HCl (1 mL) in this order, and the reaction mixture was stirred for 1 h at room temperature. The resultant mixture was extracted with AcOEt (10 mL × 3). The combined organic layer was washed with H2O (15 mL) and brine (15 mL), dried over Na2SO4, and concentrated in vacuo. The residue was purified by silica gel flash column chromatography (hexane/AcOEt 5:1) to give 4-phenylfuran-2(5H)-one (3a) (45 mg, 56%) as colorless solids (mp 92–93 ˚C, lit.,10a mp 91–93 ˚C); 1H NMR (300 MHz, CDCl3): δ 5.24 (d, J = 1.8 Hz, 2 H), 6.39 (t, J = 1.8 Hz, 1 H), 7.46–7.52 (m, 5 H); 13C NMR (75 MHz, CDCl3): δ 71.0, 113.0, 126.4, 129.3, 129.6, 131.7, 163.9, 173.8.
Further elution (hexane/AcOEt 3:1) gave 4-phenyl-3,3'-bifuran-2,5'(2'H,5H)-dione (9) (19 mg, 31%) as pale-yellow solids (mp 115–118 ˚C): 1H NMR (300 MHz, CDCl3): δ 5.01 (d, J = 2.0 Hz, 2 H), 5.13 (s, 2 H), 6.55 (t, J = 2.0 Hz, 1 H), 7.36–7.40 (m, 2 H), 7.50–7.60 (m, 3 H); 13C NMR (75 MHz, CDCl3): δ 71.6, 72.4, 118.3, 119.1, 126.9, 129.7, 130.0, 131.6, 153.1, 164.8, 170.6, 172.8; MS (ESI): calcd for C14H10O4 (242.06): found m/z 265.00 [M + Na]+; Anal. Calcd (%) for C14H10O4 (242.23): C 69.42; H 4.16. Found: C 69.32; H 4.26.
4-p-Tolyl-5,6-dihydro-2H-pyran-2-one (4b): colorless solid (mp 90–91 ˚C); 1H NMR (300 MHz, CDCl3): δ 2.40 (s, 3 H), 2.85 (dt, J = 6.0, 1.2 Hz, 2 H), 4.52 (t, J = 6.0 Hz, 2 H), 6.35 (t, J = 1.2 Hz, 1 H), 7.25 (d, J = 8.4 Hz, 2 H), 7.45 (d, J = 8.4 Hz, 2 H); 13C NMR (75 MHz, CDCl3): δ 21.2, 26.1, 65.9, 113.9, 125.8, 129.6, 132.9, 141.1, 155.1, 165.1; MS (EI): m/z (%): 188 (100) [M+], 158 (67) [M+ – CH2O], 130 (60) [M+ – CH2O – CO]; Anal. Calcd (%) for C12H12O2 (188.22): C 76.57; H 6.43. Found: C 76.45; H 6.36.
4-(4-Methoxyphenyl)-5,6-dihydro-2H-pyran-2-one (4c): colorless solid (mp 97–98 ˚C); 1H NMR (300 MHz, CDCl3): δ 2.84 (t, J = 6.0 Hz, 2 H), 3.86 (s, 3 H), 4.51 (t, J = 6.0 Hz, 2 H), 6.31 (s, 1 H), 6.95 (d, J = 8.7 Hz, 2 H), 7.52 (d, J = 8.7 Hz, 2 H); 13C NMR (75 MHz, CDCl3): δ 26.0, 55.3, 65.8, 112.6, 114.3, 127.5, 128.0, 154.6, 161.6, 165.3; MS (EI): m/z (%): 204 (100) [M+], 174 (39) [M+ – CH2O], 146 (41) [M+ – CH2O – CO]; Anal. Calcd (%) for C12H12O3 (204.22): C 70.57; H 5.92. Found: C 70.60; H 5.89.
4-(4-Chlorophenyl)-5,6-dihydro-2H-pyran-2-one (4d): colorless solid (mp 96–98 ˚C); 1H NMR (300 MHz, CDCl3): δ 2.84 (dt, J = 6.0, 1.2 Hz, 2 H), 4.53 (t, J = 6.0 Hz, 2 H), 6.36 (t, J = 1.2 Hz, 1 H), 7.42 (d, J = 8.7 Hz, 2 H), 7.48 (d, J = 8.7 Hz, 2 H); 13C NMR (75 MHz, CDCl3): δ 26.1, 65.8, 115.3, 127.2, 129.2, 134.3, 136.7, 153.8, 164.6; MS (EI): m/z (%): 208 (100) [M+], 178 (77) [M+ – CH2O], 150 (35) [M+ – CH2O – CO]; Anal. Calcd (%) for C11H9ClO2 (208.64): C 63.32; H 4.35. Found: C 63.09; H 4.34.
4-(4-Formylphenyl)-5,6-dihydro-2H-pyran-2-one (4e): colorless solid (mp 137–139 ˚C); 1H NMR (300 MHz, CDCl3): δ 2.91 (dt, J = 6.0, 1.2 Hz, 2 H), 4.57 (t, J = 6.0 Hz, 2 H), 6.46 (t, J = 1.2 Hz, 1 H), 7.70 (d, J = 8.7 Hz, 2 H), 7.96 (d, J = 8.7 Hz, 2 H), 10.07 (s, 1 H); 13C NMR (75 MHz, CDCl3): δ 26.2, 65.9, 117.4, 126.5, 130.02, 137.3, 141.6, 153.7, 164.2, 191.2; MS (EI): m/z (%): 202 (100) [M+], 172 (9) [M+ – CH2O], 144 (50) [M+ – CH2O – CO]; Anal. Calcd (%) for C12H10O3 (202.21): C 71.28; H 4.98. Found: C 71.09; H 5.10.
ACKNOWLEDGEMENTS
This research was partially supported by the MEXT, Grant-in-Aid for Scientific Research (B), 20350045, Scientific Research on Priority Area "Chemistry on Concerto Catalysis" (20037018). We thank Prof. K. Tomooka (Kyushu University) for use of a NMR spectrometer and Profs. H. Suzuki, T. Takao, T. Ikariya, and S. Kuwata (Tokyo Instituted of Technology) for use of mass spectrometers.
References
1. For reviews, see: Y. S. Rao, Chem. Rev., 1976, 76, 625; CrossRef D. W. Knight, Contemp. Org. Synth., 1994, 1, 287; CrossRef E. Negishi and M. Kotora, Tetrahedron, 1997, 53, 6707; CrossRef J. C. Slaughter, Biol. Rev., 1999, 74, 259; CrossRef R. Brückner, Chem. Commun., 2001, 141; CrossRef N. B. Carter, A. E. Nadany, and J. B. Sweeney, J. Chem. Soc., Perkin Trans. 1, 2002, 2324. CrossRef
2. For a review, see: V. Boucard, G. Broustal, and J. M. Campagne, Eur. J. Org. Chem., 2007, 225. CrossRef
3. Y. Yamamoto, N. Kirai, and Y. Harada, Chem. Commun., 2008, 2010. CrossRef
4. Y. Yamamoto and N. Kirai, Org. Lett., 2008, 10, 5513. CrossRef
5. For examples of transition metal-catalyzed hydroarylation/lactonization using alkynoates and arylboronic acids, see: (a) C. H. Oh, S. J. Park, J. H. Ryu, and A. Kumar Gupta, Tetrahedron Lett., 2004, 45, 7039; CrossRef (b) M. Alfonsi, A. Arcadi, M. Chiarini, and F. Marinelli, J. Org. Chem., 2007, 72, 9510. CrossRef
6. For examples of transition metal-catalyzed hydroarylation/lactonization using alkynoates and aryl halides and congeners, see: (a) A. Arcadi, E. Bernocchi, A. Burini, S. Cacchi, F. Marinelli, and B. Pietroni, Tetrahedron, 1988, 44, 481; CrossRef (b) A. Arcadi, E. Bernocchi, A. Burini, S. Cacchi, F. Marinelli, and B. Pietroni, Tetrahedron Lett., 1989, 30, 3465; CrossRef (c) A. Arcadi, S. Cacchi, G. Fabrizi, F. Marinelli, and P. Pace, Eur. J. Org. Chem., 1999, 3305. CrossRef
7. Palladium-catalyzed 2:2 coupling of diarylalkynes and arylboronic acids has been reported, see: (a) T. Satoh, S. Ogino, M. Miura, and M. Nomura, Angew. Chem. Int. Ed., 2004, 43, 5063; CrossRef (b) H. Horiguchi, H. Tsurugi, T. Satoh, and M. Miura, Adv. Synth. Catal., 2008, 350, 509. CrossRef
8. Oligobutenlides were obtained from the Rh-catalyzed hydroarylation of ethyl 4-hydroxy-4-dimethyl-2-pentynoate with phenylboron reagents, see ref 5b.
9. (a) S. P. Shahi and K. Koide, Angew. Chem. Int. Ed., 2004, 43, 2525; CrossRef (b) E. Piers, J. M. Chong, and H. E. Morton, Tetrahedron, 1989, 45, 363; CrossRef (c) M. E. Jung and J. A. Hagenah, J. Org. Chem., 1987, 52, 1889; CrossRef (d) A. T. Koppisch, B. S. J. Blagg, and C. D. Poulter, Org. Lett., 2000, 2, 215; CrossRef (e) D. Ma and X. Lu, Tetrahedron, 1990, 46, 6319. CrossRef
10. (a) T. Taniguchi, H. Nagata, R. M. Kanada, K. Kadota, M. Tekeuchi, and K. Ogasawara, Heterocycles, 2000, 52, 67; CrossRef (b) P. Yates and J. A. Eeisbach, J. Am. Chem. Soc., 1963, 85, 2943; (c) J. M. Stewart and D. W. Woolley, Biochem., 1964, 3, 1998. CrossRef